Sensory input-dependent gain modulation of the optokinetic nystagmus by mid-infrared stimulation in pigeons
Figures
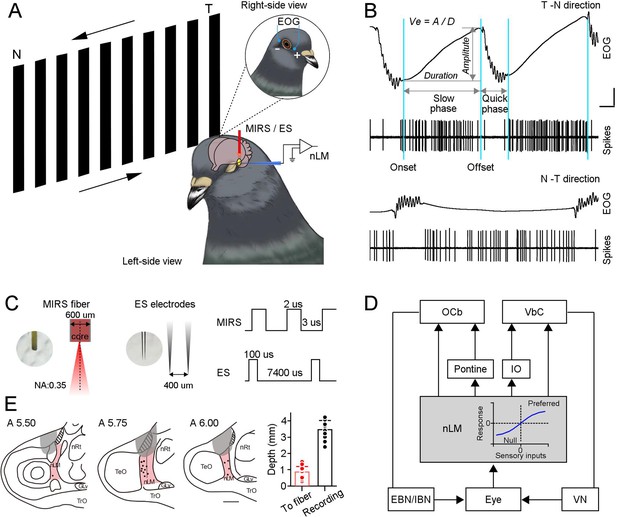
MIRS or ES applications to the pretectal nLM in pigeons.
(A) Schematic drawing of neuronal activities (extracellular spikes) and behavioral performances (EOG) recording systems in awake-behaving pigeons, together with the arrangement of MIRS fiber or ES electrodes. Animals viewed grating motion in the T-N and N-T directions. (B) Raw traces of eye movements and action potentials of an example nLM while a pigeon viewing grating motion in the T-N (top traces) and N-T direction (bottom traces). Sky blue lines label time points of the onset and offset of pursuit eye movements during the slow phase of OKN. Arrow segments show the measurement of the amplitude and duration of the slow phase. An equation on the top defines pursuit eye velocity as amplitude divided by duration of pursuit eye movement. (C) The parameters of MIRS and ES used in the study. (D) The retina-nLM-cerebellum circuit involving the OKN eye movement generation in birds. nLM is a core sensory center that processes the direction and speed of visual motion in a large field and transfers these signals to the oculomotor system. The inserted plot in the gray box showed that firing responses of nLM evoked as a function of visual inputs. (E) Marked recording sites (dots) and MIRS/ES sites (gray shading) in the pretectal nLM (pink shading) across brain sections under study (from the interaural midpoint, A 5.5–6.0). On the right panel, the red bar presents the distance between the fiber tip and marked recording sites (n=15 pigeons, red dots). The black bar shows the depth of recorded neurons when recording electrodes were introduced laterally (n=37 neurons, black dots), as shown in A. Abbreviations are: OCb, oculomotor cerebellum; VbC, vestibular cerebellum; IO, inferior olive; nLM, the pretectal nucleus lentiformis mesencephali; EBN, excitatory burst neurons; IBN, inhibitory burst neurons; VN, vestibular nucleus; nRt, the nucleus rotundus; TeO, the optic tectum.
-
Figure 1—source data 1
Numerical data for Figure 1B and E.
- https://cdn.elifesciences.org/articles/78729/elife-78729-fig1-data1-v1.xlsx
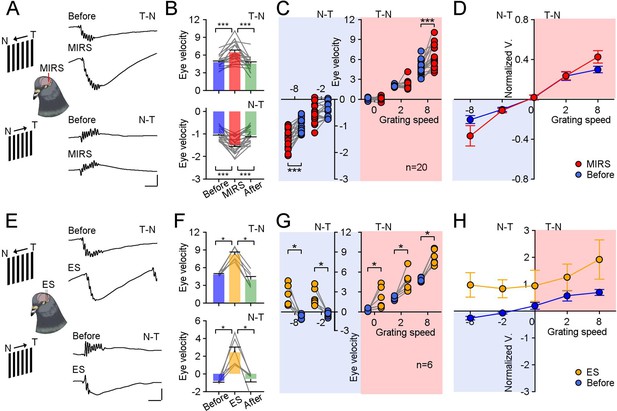
Comparison of modulation effects by MIRS and ES on OKN eye movements under different directions and velocities of grating motion.
(A and E) Raw traces of OKN eye movements modulated by MIRS (A) and ES (E) while pigeons viewing grating motion in the T-N and N-T direction (top to bottom traces: before and during MIRS/ES in the T-N and N-T direction motion). Scale bars: 200ms, 4 deg. (B and F) Comparison of pursuit eye velocities to T-N and N-T grating motion (top and bottom plots) before, during, and after MIRS (B, n=20 pigeons) and ES (F, n=6 pigeons). (C and G) MIRS (C) and ES (G) modulated pursuit eye velocities under different grating motion stimuli (blue symbols: before stimulation; red symbols: during MIRS; yellow symbols: during ES). Two-sided Wilcoxon signed-rank test, * p<0.05, *** p<0.001. Gray lines represent data from individual animals. (D and H) Pursuit eye velocity data normalized to average values from the top 10 trials with highest peak pursuit velocities before MIRS (D) and ES (H) under different grating speeds. Error bars represent 1 SEM.
-
Figure 2—source data 1
Numerical data for Figure 2.
- https://cdn.elifesciences.org/articles/78729/elife-78729-fig2-data1-v1.xlsx
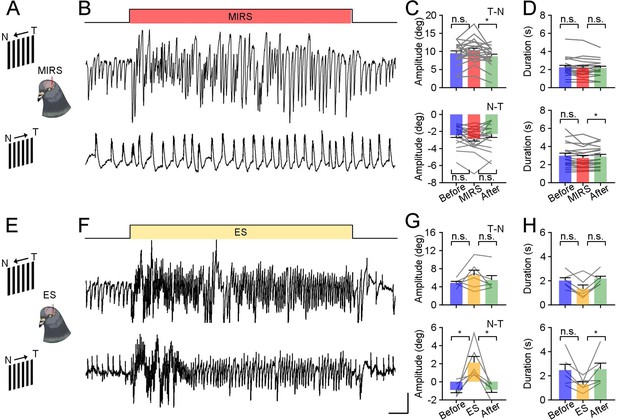
Modulation of sensorimotor behaviors by MIRS and ES in pigeons.
Schematic drawings of MIRS (A) and ES (E) applied in pigeons, while animals viewing visual stimuli of grating motion at 8 deg/s in the T-N and N-T direction. (B and F) Raw EOG traces of evoked OKN eye movements in the T-N and N-T direction (from top to bottom traces) during 120 s MIRS (B) or 120 s ES (F) in example animals. Scale bars: 10 s, 4 deg. (C and G) Comparison of amplitudes of pursuit eye movements during the slow phase of OKN in the T-N and N-T grating motion (from top and bottom plots) during MIRS (C, n=20) and ES (G, n=6). (D and H) Comparison of durations of pursuit in the T-N and N-T grating motion (from top and bottom plots) during MIRS (D, n=20) and ES (H, n=6). * p<0.05, ** p<0.01, two-sided Wilcoxon signed-rank test. Error bars represent 1 SEM. Gray lines represent data from individual animals.
-
Figure 2—figure supplement 1—source data 1
Numerical data for Figure 2—figure supplement 1B-D and F-H.
- https://cdn.elifesciences.org/articles/78729/elife-78729-fig2-figsupp1-data1-v1.xlsx
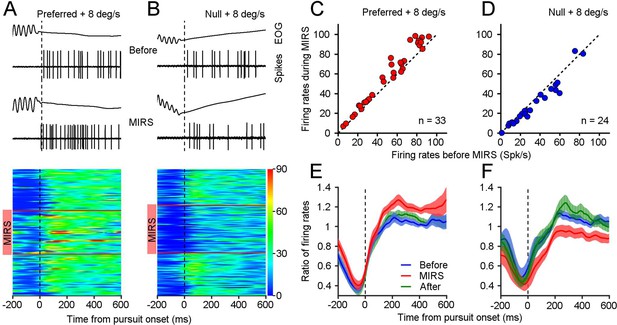
MIRS modulates visual responses of nLM neurons during the slow phase of OKN.
(A and B) Representative eye movements and spiking responses to grating motion at 8 deg/s in the preferred (A) and null direction (B) of an example neuron. From top to bottom: example raw traces of EOG signals and action potential responses in a single OKN eye movement, and visual responses of the example neuron during individual OKN eye movements before, during, and after MIRS. Each horizontal colored band shows neuronal firing rates during pursuit of one OKN. Neuronal responses are color-coded with a scale on the right color bar (spikes/s). Red horizontal lines show the beginning and ending timepoints of MIRS. (C and D) Visual responses of population neurons showed the effect of MIRS in preferred (C, n=33 neurons) and null directions (D, n=24 neurons). Data points lie well above or below the dashed unity line. (E and F) Ratio of neuronal firing rates across population responses to grating motion at 8 deg/s before, during, and after MIRS in the preferred (E) and null (F) directions. Data were normalized to the mean value of visual responses in the 600ms interval after the onset of pursuit before stimulation. Error bars represent 1 SEM.
-
Figure 3—source data 1
Numerical data for Figure 3.
- https://cdn.elifesciences.org/articles/78729/elife-78729-fig3-data1-v1.xlsx
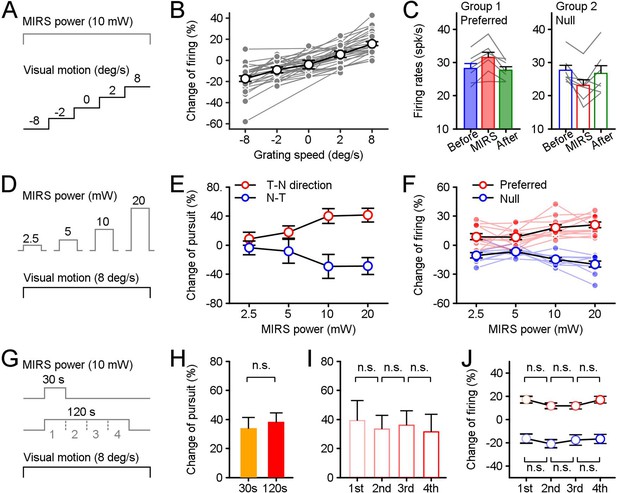
Effects of stimulus parameters on MIRS modulations of behavioral and neuronal responses.
The parameters included the strength of visual inputs (A), the MIRS output power (D), and the duration of MIRS irradiation (G). (A–C) MIRS alters sensory coding in pretectal neurons associated with the level of visual responses. (B) The change ratio of visual responses across populations was correlated with the strength of visual inputs. (C) MIRS effects on different neuronal populations with similar evoked firing rates to grating motion at 8 deg/s in the preferred (Group 1, n=7 neurons) and null (Group 2, n=7 neurons) directions. (D–F) The size of MIRS output power alters modulations on behavioral and neuronal responses. (E) MIRS modulations on pursuit eye movements in the T-N and N-T directions increased with the MIRS output power. (F) MIRS effects on neuronal responses in the preferred (n=12 neurons) and null (n=9 neurons) directions increased with the MIRS output power. Filled symbols and lines show data from individual neurons. Open symbols with black line show the average across populations. (G–J) The duration of MIRS irradiation failed to modify modulations on behavioral and neuronal responses. (H) Comparison of 30 s (n=5 pigeons) and 120 s (n=20 pigeons) MIRS on pursuit eye velocities in the T-N direction. (I and J) Comparison effects on behavioral and neuronal responses in each 30 s period of 120 s MIRS irradiation. Pursuit velocity in the T-N direction (n=20 pigeons, I) and neuronal responses in the preferred (red cycles, n=33 neurons) and null (blue cycles, n=24 neurons) directions (H) failed to show significant changes across different periods. Two-sided Wilcoxon signed rank test, p>0.05. Error bars represent 1 SEM.
-
Figure 4—source data 1
Numerical data for Figure 4.
- https://cdn.elifesciences.org/articles/78729/elife-78729-fig4-data1-v1.xlsx
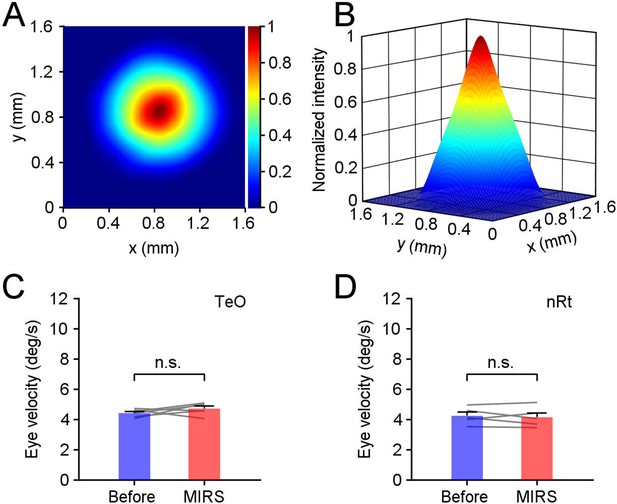
Profile of the mid-infrared light and the MIRS effects in other visual regions of pigeons.
MIRS with 34.88 THz is coupled and emitted from the fiber tip. We scanned the intensity map of light spot at a test surface with a distance of 850 μm from the fiber tip, with a scanning resolution of 100 μm along x and y axis. Between any two sample points, we fitted data using the linear interpolation with a step of 10 μm. Data are presented in two-dimensional (A) and three-dimensional (B) views. The spot diameters were ~1000 μm in both x and y axis when the laser intensity was reduced to 1/e2 of the peak in our setting. (C) and (D) Modulation of pursuit eye movements during the slow phase when MIRS was applied in the optic tectum (TeO) (C, n=6 MIRS times in 3 pigeons) and the nucleus rotundus (nRt) (D, n=5 MIRS times in 3 pigeons). p>0.05, two-sided Wilcoxon signed-rank test. Error bars represent 1 SEM. Gray lines represent data from individual MIRS.
-
Figure 4—figure supplement 1—source data 1
Numerical data for Figure 4—figure supplement 1.
- https://cdn.elifesciences.org/articles/78729/elife-78729-fig4-figsupp1-data1-v1.xlsx
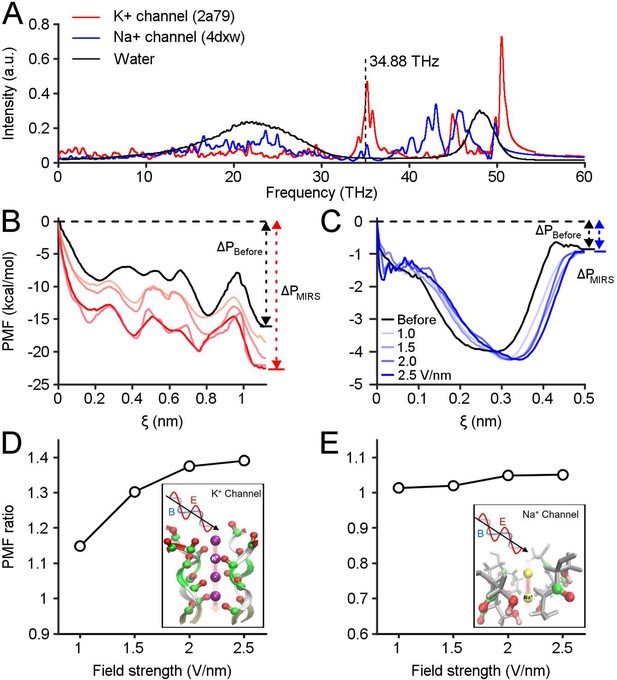
Computational simulations reveal preferential regulation of K+ channels by MIRS.
(A) Absorption spectra of K+and Na+ channels calculated using a molecular dynamics simulation. The vertical dashed line indicates MIRS with a frequency of 34.88 THz used in this study. (B, C) The potential of mean force (PMF) of K+and Na+ions permeate through ion channels before and during MIRS with a frequency of 34.88 THz, under field strengths varied from 0 to 2.5 V/nm (black and colored lines), respectively. (D) The PMF ratio of K+ channels increased by 1.15–1.4 folds at the exit site around ξ=1.1 nm when the field strength increased from 1.0 to 2.5 V/nm. (E) The ratio of Na+ channels was kept closely to 1 at the exit site around ξ=0.5 nm under different field strengths.
-
Figure 5—source data 1
Numerical data for Figure 5.
- https://cdn.elifesciences.org/articles/78729/elife-78729-fig5-data1-v1.xlsx
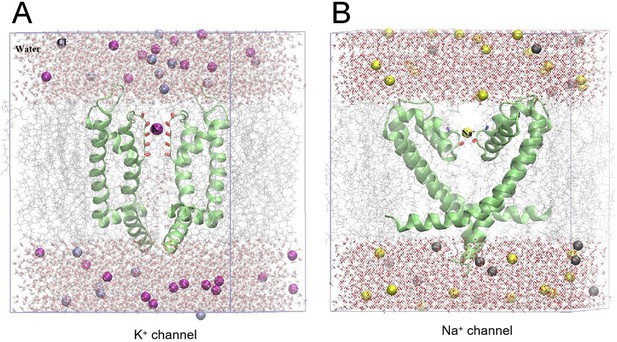
The composite atomic models contain the K+ channel (A) and the Na+ channel (B).
In our models, we constructed channels containing the whole protein placed at the middle of phospholipid bilayer to separate water and ions on each side. There are 12 sodium, 12 potassium and 20 chloride ions for K+ channels, and 14 sodium, 14 potassium and 24 chloride ions for Na+ channels, respectively. Both the water thickness of K+ and Na+ channels are ~0.3 nm. (A) The K+ channel (PDB ID: 2a79) contains 10,294 atoms, including 2,725 TIP3P water molecules, 12 K+ and 20 Cl- ions, thus the concentration of a salt solution is 0.15 M. The protein has four negative charges, the addition of four counterbalance ions ensures that the whole system is electrically neutral. The size of the PBC box is 5.04 nm × 5.16 nm×6.25 nm. (B) The Na+ channel (PDB ID: 4dxw) contains 14,922 atoms, including 4,067 water molecules, 14 Na+ and 24 Cl- ions, thus the concentration of a salt solution is 0.15 M. Similarly, the addition of four counterbalance ions also ensures that the whole system is electrically neutral. The size of the PBC box is 5.40 nm × 5.40 nm×7.30 nm. The purple atom represents K+, the yellow atom represents Na+, and the gray atom represents Cl-. The TIP3P water molecule is displayed in the format of a red ball stick. Green strips show K+ and Na+ channels, red stripes show −C=O groups located at the inner wall of the filter region, blue stripes show -OH- groups of the filter, and light gray lines show a phospholipid bilayer, respectively.
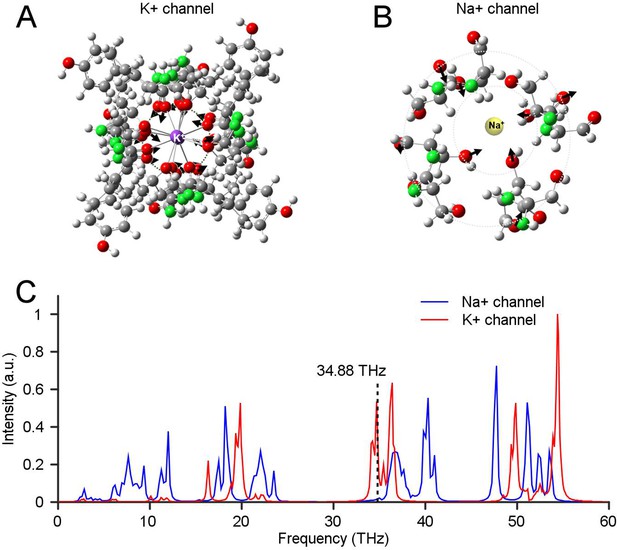
The eigen-modes and intrinsic spectrum calculation for K+ and Na+ channels.
(A and B) Calculations on the eigen-mode frequencies of –C=O groups for K+ channels, and –C=O, –OH− groups for Na+ channels. The filter structures extracted from the model of K+ channels (PDB ID: 2a79) contained 284 atoms (A), and Na+ channels (PDB ID: 4dxw) contained 96 atoms (B). (C) The intrinsic spectrum was calculated by using Gaussian 09 code based on the density functional theory (DFT) methods at B3LYP/6-31G(d) level.
-
Figure 5—figure supplement 2—source data 1
Numerical data for Figure 5—figure supplement 2C.
- https://cdn.elifesciences.org/articles/78729/elife-78729-fig5-figsupp2-data1-v1.xlsx
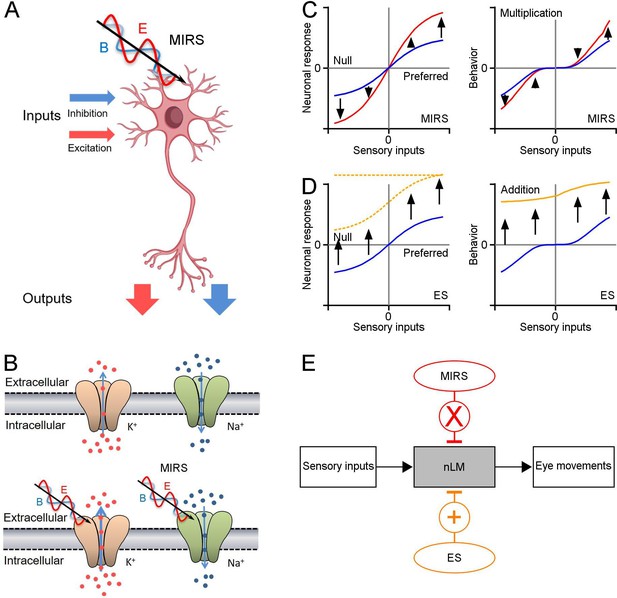
Schematic diagrams showing the mechanism of MIRS and ES suggested by our data.
(A) MIRS produces gain modulations on visual responses in the same nLM neuron. (B) MIRS preferentially regulates the permeation of K+ channels instead of Na+ channels. (C) MIRS exerts multiplicative gain modulations on neuronal responses to sensory and behavior performance, suggested by our experimental data. Blue and red lines present neuronal and behavioral responses before and during MIRS. (D) ES could exert additive modulations on neuronal firing and cause unidirectional deflections in behaviour in our results. Blue and yellow lines present neuronal and behavioral responses before and during ES. Dashed lines present the possible changes in neuronal firing by ES suggested by prior research. (E) Summary of different effects of MIRS and ES on sensorimotor transformation: gain modulation by MIRS and additive modulation by ES.
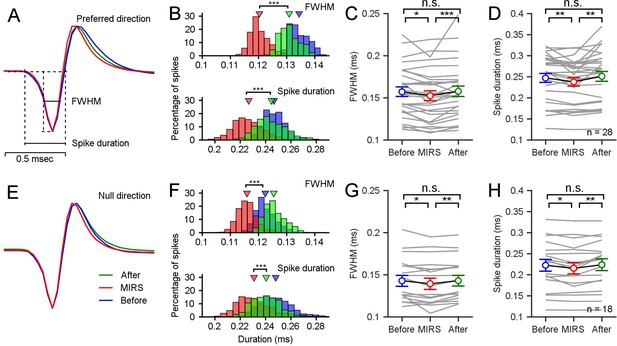
Extracellar recordings showed shortened action potential durations of pretectal nLM neurons during MIRS in the preferred (A–D) and null direction (E–H).
(A and E) average waveforms of action potentials from an example pretectal neuron before, during and after MIRS to grating motion at 8 deg/s in its preferred (A) and null (E) direction. (B and F) Distributions of the full width at half maximum (FWHM) and the duration of spikes’ negative phases (Spike duration) of the example pretectal neuron’s action potentials (*** p<0.001, two-sided Wilcoxon rank sum test). (C, D, G, and H) Statical summarization of the FWHM and the Spike duration across population neurons in the preferred (C and D, n=28 neurons) and null direction (G and H, n=18 neurons). To reduce the influence of recording noises on the definition of duration, we analyzed neurons with a higher signal-to-noise ratio above 4:1 in recording. * p<0.05, ** p<0.01, *** p<0.001, two-sided Wilcoxon signed-rank test. Error bars represent 1 SEM.
-
Figure 6—figure supplement 1—source data 1
Numerical data for Figure 6—figure supplement 1.
- https://cdn.elifesciences.org/articles/78729/elife-78729-fig6-figsupp1-data1-v1.xlsx
Additional files
-
MDAR checklist
- https://cdn.elifesciences.org/articles/78729/elife-78729-mdarchecklist1-v1.docx
-
Source code 1
Custom code to determine the onset and offset timepoints of the slow phases in OKN.
- https://cdn.elifesciences.org/articles/78729/elife-78729-code1-v1.zip