Functional interactions among neurons within single columns of macaque V1
Figures
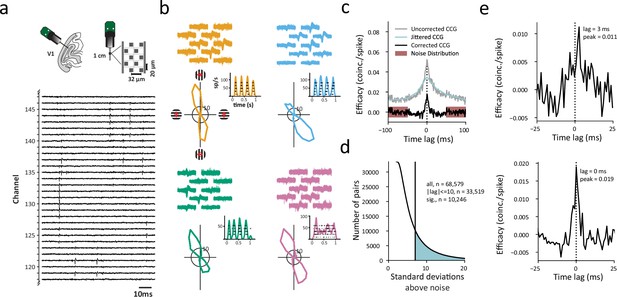
Identifying functional interactions within single columns of visual cortex.
(a) Upper left cartoon depicts the angle of Neuropixels probe penetrations made into the lateral surface and underlying calcarine sulcus of V1. Upper right, Neuropixels probe base and shank, and layout of electrode contacts for a section of the recording shank. Lower, raw voltage traces recorded from an exemplar section of channels and time period. (b) Example single-neuron recordings with Neuropixels probes, three simple cells (orange, blue, green) and one complex cell (purple). Top, spike waveforms recorded across multiple adjacent electrode contacts are shown for each neuron. Bottom, each neuron’s response to its preferred orientation (rasters and instantaneous spike rates) and their corresponding tuning curves. Red arrows (upper left) denote the drift direction of oriented gratings. (c) Example CCG between an example pair of V1 neurons. Corrected CCGs were generated from the difference between a jittered and an uncorrected CCG. Significance of each CCG was determined from comparisons between the peak and the noise distribution. (d) Distribution of ratios of CCG peaks to the noise (SD) for all recorded pairs. Shaded area denotes CCGs with peaks >7 SDs above the mean of the noise distribution. (e) Two example CCGs differing in both peak lag and peak efficacy.
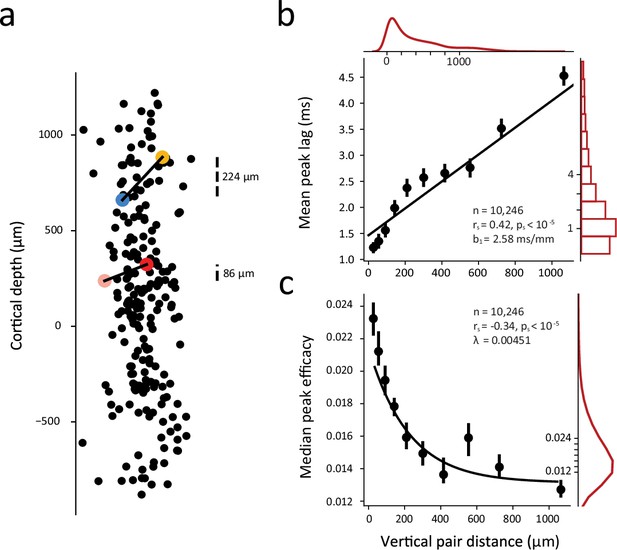
Dependence of synchrony and strength of functional interactions on vertical distance within single cortical columns.
(a) Example session (M1, session 3) with 221 visually responsive neurons recorded simultaneously and their locations across cortical depth. (Horizontal axis is magnified for visualization). Cortical depth 0 denotes the boundary between Layer 4c and Layer 5. Laminar boundaries were determined using histological data and current-source-density (CSD) profile for each session (Methods). Two example correlated pairs from Figure 1e with varied CCGs are shown in color (blue-yellow pair and pink-red pair corresponding to Figure 1e top and bottom, respectively). (b) Linear dependence of synchrony on vertical pair distance. (c) Strength of CCGs decay with greater pair distance. In b and c, all significantly correlated pairs from all sessions are combined and each dot denotes the mean peak lag or median peak efficacy of significantly correlated CCGs within a (10% quantile) vertical distance bin. Error bars denote 95% confidence intervals. Black lines denote the linear and exponential fits in b and c, respectively; slope (b) and decay constant (λ) are shown. Red lines and bar plots show marginal distributions.
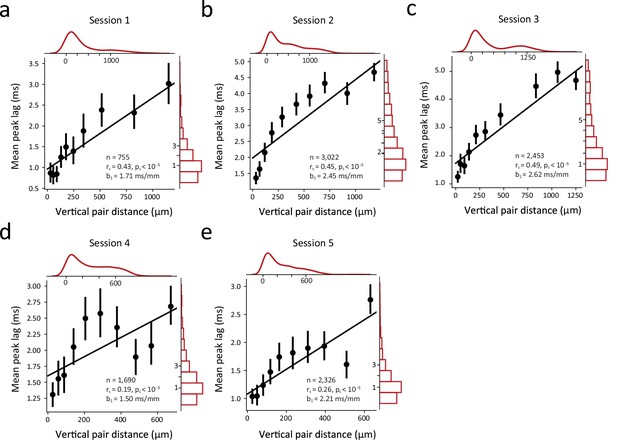
Dependence of synchrony on vertical distance within single cortical columns for all individual sessions.
Linear dependence of synchrony on vertical pair distance for each of the 5 sessions (a–e). Plots follow the conventions used in Figure 2.
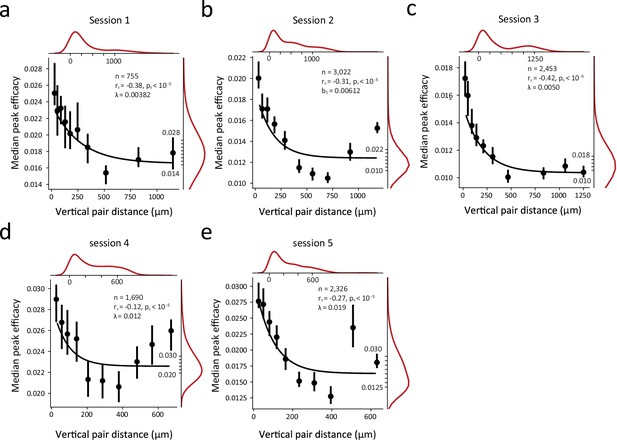
Dependence of the strength of functional interactions on vertical distance within single cortical columns for all individual sessions.
Peak efficacy of CCGs decays with greater pair distance in each of the 5 sessions (a–e). Plots follow the conventions used in Figure 2.
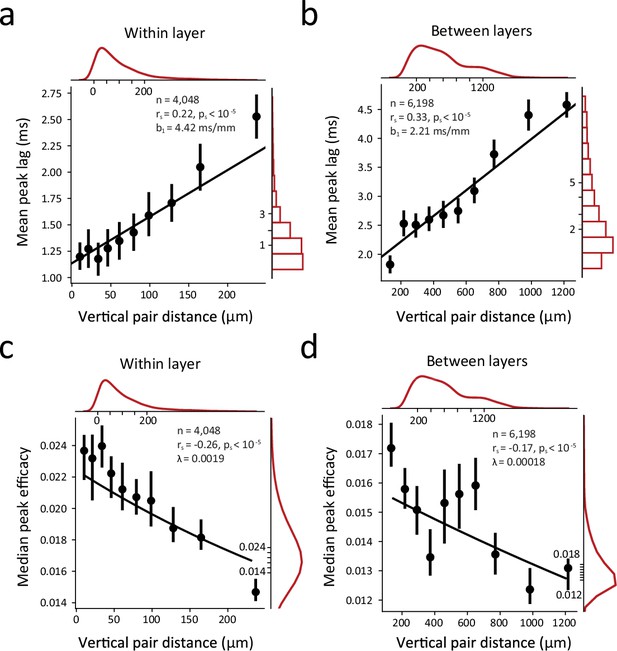
Dependence of the synchrony and strength of functional interactions on vertical distance within single cortical columns, separated by laminar pairings.
(a–b) Linear dependence of CCG peak lag on the distance between neurons for pairs of neurons composed of two neurons in the same layer of V1 (a) or two neurons in different layers of V1 (b). (c–d) Peak efficacy of CCGs decays with greater pair distance in pairs of neurons composed of two neurons in the same layer (c) and different layers (d). Plots follow the conventions used in Figure 2.
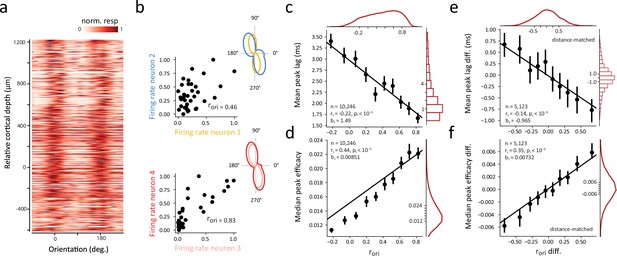
Dependence of synchrony and strength of functional interactions on tuning similarity within single cortical columns.
(a) Heat map of visual responses across drift directions of oriented gratings and across cortical depth. The response tuning of each of 802 neurons was aligned to the overall preferred orientation shared by neurons recorded from the same session, and all sessions were combined. (b) Signal correlation between exemplar neurons. Left, Scatter plot of normalized responses to different stimulus orientations (n=36) for the two example pairs shown in Figure 1e and Figure 2a. Signal correlations (rori) are also shown. Right, each neuron’s orientation tuning curve. (c) Linear dependence of synchrony on the corresponding signal correlation. (d) Linear dependence of CCG strength on the corresponding signal correlation. (e) Difference in peak lag of distance-matched CCGs was negatively correlated with difference in signal correlation. (f) Difference in peak efficacy of distance-matched CCGs was positively correlated with difference in signal correlation. In c-f, all significantly correlated pairs from all sessions are combined and each dot denotes mean peak lag or median peak efficacy of significantly correlated CCGs within a (10% quantile) signal correlation bin. Error bars denote 95% confidence intervals. Black lines denote linear fits; slopes (b) are shown. Red lines and bar plots show marginal distributions.
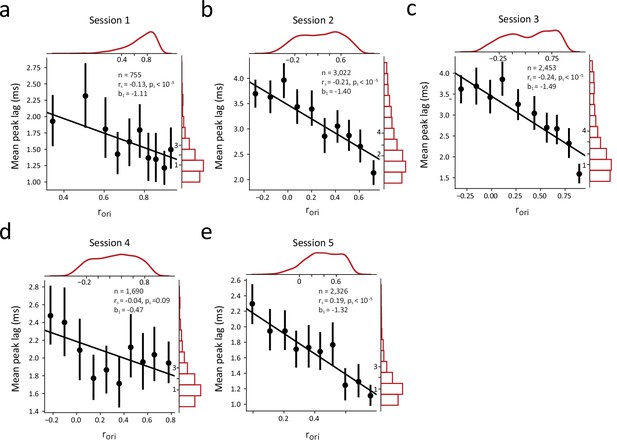
Dependence of synchrony on tuning similarity within single cortical columns for all individual sessions.
Linear dependence of synchrony on tuning similarity in each of the 5 sessions (a–e). Plots follow the conventions used in Figure 3.
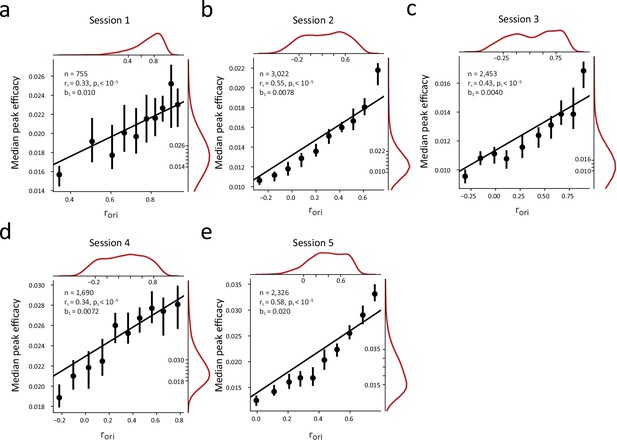
Dependence of the strength of functional interactions on tuning similarity within single cortical columns for all individual sessions.
Peak efficacy of CCGs is positively correlated with tuning similarity in each of the 5 sessions (a–e). Plots follow the conventions used in Figure 3.
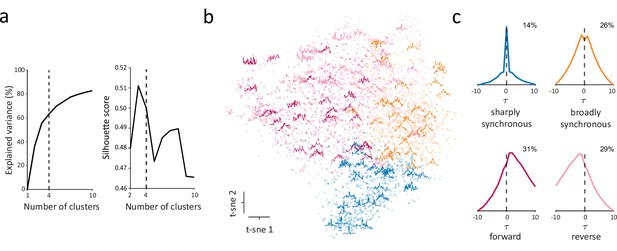
Identification of distinct classes of functional interactions within the full population.
(a) Explained variance (left) and silhouette score (right) as a function of number of clusters. The dashed vertical line indicates the selected number of clusters (n=4). (b) Scatter plot of dimensionality-reduced CCGs in the first two dimensions of t-SNE space. Randomly selected example CCGs are overlayed on the scatterplot in their corresponding location in t-SNE space. (c) CCG templates generated by averaging over all the CCGs in each cluster. The templates include a ‘sharply synchronous’ class (Ssync) with a narrow peak at , a ‘broadly synchronous’ class (Bsync) with a wide peak at , a ‘forward’ class (Fasync) (leading) with more probability density after and a ‘reverse’ class (Rasync) (lagging) with more probability density before . Numbers denote the percentage of each class among all significantly correlated pairs.
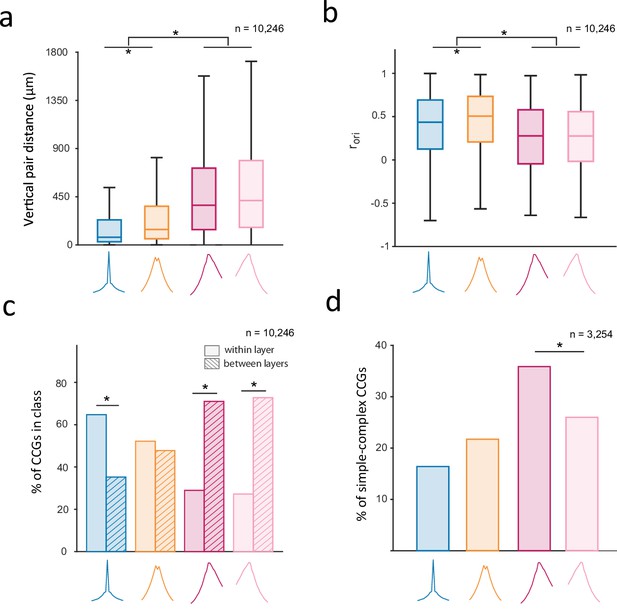
Corroboration of putative classes of functional interactions with V1 microcircuitry.
(a, b) Boxplots of vertical pair distance (a) and orientation signal correlation (b) across the 4 identified CCG classes. Boxplots illustrate the medians, first and third quartiles, and non-outlier (1.5*IQR method) minima and maxima. Asterisks denote significant differences in medians between pairs of classes (Wilcoxon rank-sum test; P<0.05, Bonferroni corrected). (c) Percentage of CCGs in each class composed of two neurons from the same (‘within layer’) or different layers (‘between layer’). In a-c, the reference neuron in a neuronal pair was selected randomly. (d) Distribution of putative CCG classes among neuronal pairs composed of a simple cell as the reference neuron and complex cell as the target neuron. Only the pairwise comparison between Fasync and Rasync is illustrated to show the direction of functional interactions between simple and complex cells.
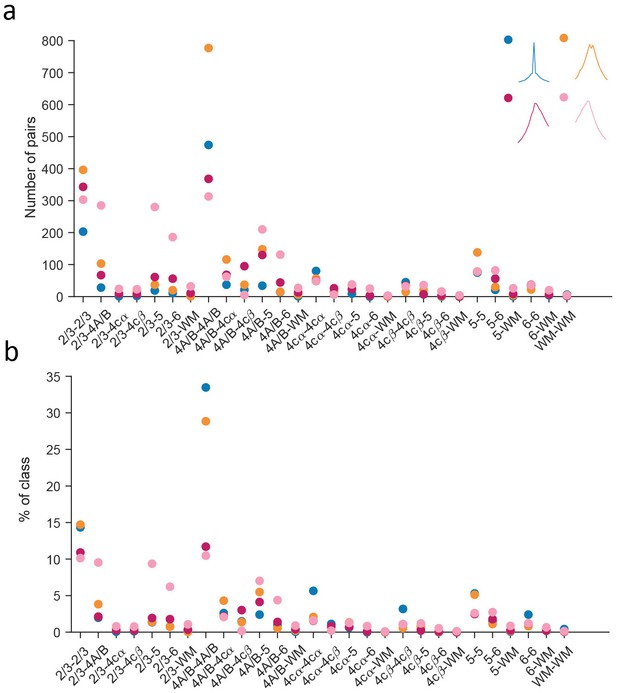
Distribution of putative CCG classes across layer pairings.
Plotted is the number (a) and percentage (b) of significant CCGs in each class composed of two neurons in the indicated layer pairing. For pair of neurons from the same layer, the reference neuron was selected randomly. For pair of neurons from different layers, the reference neuron was chosen from the layer noted first. For example, a CCG in the 2/3–4A/B pairing is composed of a reference neuron in layer 2/3 and a target neuron in 4A/B.
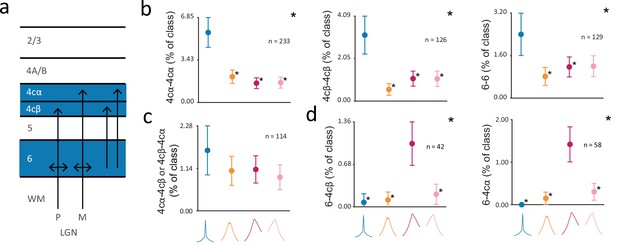
Distribution of different putative classes of functional interactions within V1 input layers.
(a) Diagram of dLGN input to V1 layers 4cα, 4cβ, and 6; dLGN axons terminate in layers 4cα and 4cβ and layer 6, and layer 6 projects to layers 4cα and 4cβ. (b) Percentage of CCGs in each class composed of two neurons in layer pairings of 4cα-4cα, 4cβ-4cβ, or 6–6, out of all the pairwise layer pairing combinations. (c) Percentage of CCGs in each class composed of one neuron in layer 4cα and one in 4cβ (4cα-4cβ or 4cβ-4cα). In (b-c), the reference neuron in a neuronal pair was selected randomly. (d) Percentage of CCGs in each class composed of a reference neuron in layer 6 and a target neuron in 4cβ (left) or 4cα (right). For (b-d), error bars denote 95% confidence intervals. Large asterisks denote significant chi-squared test across all classes (p<0.05, Bonferonni corrected). Small asterisks denote significant chi-squared tests between a particular class and the class with the maximum percentage (p<0.05, Bonferroni corrected).
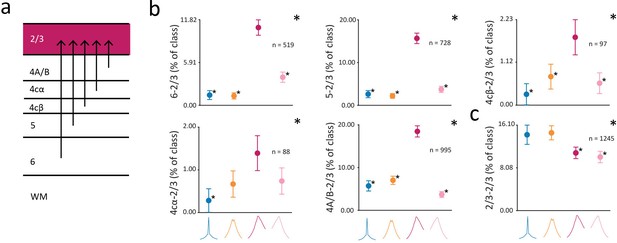
Distribution of different putative classes of functional interactions with layer 2/3.
(a) Diagram of input to layer 2/3 from V1 laminar compartments. (b) Percentage of CCGs in each class composed of a reference neuron in layer 6, 5, 4cβ, 4cα, or 4A/B and a target neuron in layer 2/3. (c), Percentage of CCGs in each class composed of pairs of neurons in layer 2/3. The reference neuron in a neuronal pair was selected randomly. For b-c, conventions are the same as in Figure 6.
Tables
Dependence of putative classes on laminar pairing and vertical distance for pairs of neurons separated by 86–310 μm.
Dependent variable (in/out of cluster) | Predictor | Coefficient | Standard error | p-Value |
---|---|---|---|---|
Ssync | Distance | –0.0043 /μm | 0.001 | 4.00*10–5 |
Layer | 0.104/layer | 0.126 | 0.41 | |
Bsync | Distance | –0.0017 /μm | 0.0007 | 9.24*10–3 |
Layer | 0.244/layer | 0.085 | 4.11*10–3 | |
Fasync or Rasync | Distance | 0.0032 /μm | 0.0005 | 4.23*10–7 |
Layer | –0.262/layer | 0.0802 | 1.13*10–3 |
-
Coefficients, standard errors, and p-values from logistic regressions predicting class membership using the distance between pairs of neurons and whether pairs were located in the same or different cortical layer(s). Only pairs of neurons with pair distances greater than the 5% of pairs located in different cortical layers (>86 μm) and less than 5% of pairs located in the same cortical layer (<310 μm) were included. Significant predictors are highlighted.
Number of CCGs with peaks or troughs significantly above or below noise.
Stdev. above/below noise | Number of pairs with peaks above noise | Number of pairs with troughs below noise |
---|---|---|
1 | 33,502 | 15,582 |
2 | 33,502 | 11,347 |
3 | 31,660 | 4,537 |
4 | 25,180 | 1,555 |
5 | 18,739 | 603 |
6 | 13,757 | 265 |
7 | 10,246 | 136 |
-
The number of recorded pairs with a peak or trough at least 1–7 standard deviations (SD) above or below noise is shown. Only CCGs with peaks or troughs within 10ms of zero time lag were considered. 136 CCGs had troughs that were more than 7 SD below noise whereas 10,246 CCGs had peaks that were more than 7 SD above noise.
Percentage of overlapping spikes | >5% | >10% | >20% |
N_pair_overlapped | 26 | 16 | 7 |
N_pair_overlapped /N_pair_sharp synchronous | 26/1408 =1.85% | 16/1408 =1.14% | 7/1408 =0.5% |