Epac2 in midbrain dopamine neurons contributes to cocaine reinforcement via enhancement of dopamine release
Figures
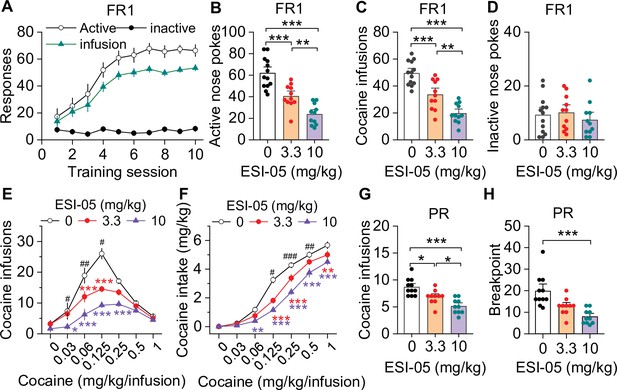
ESI-05 dose-dependently decreased cocaine self-administration under fixed ratio-1 (FR1) and progressive ratio (PR) reinforcement schedules in mice.
(A) Active nose pokes and inactive nose pokes and cocaine infusions during 10-day FR1 self-administration training (0.5 mg/kg/infusion, n=18, 9 male and 9 female). The FR1 schedule has a brief timeout (10 s) period following each active nose poke during which additional nose pokes do not result in cocaine infusions. As a result, the number of nose pokes is higher than the number of cocaine infusions. (B–D) The mean number of active nose pokes (one-way ANOVA: F2,32 = 30.9, p<0.001), cocaine infusions (one-way ANOVA: F2,32=31.9, p<0.001), and inactive nose pokes (one-way ANOVA: F2,32 = 0.5, p=0.613) in response to ESI-05 pretreatments (0, 3.3, 10 mg/kg, i.p.) under an FR1 reinforcement schedule (n=11–13, 6 male and 5–7 female per group). (E–F) ESI-05 pretreatments produced a significant downward shift in the dose-response curve for cocaine infusions (two-way repeated-measures ANOVA: ESI-05 treatment, F2,22 = 45.5, p<0.001; cocaine dose, F6,132 = 51.0, p<0.001; ESI-05 treatment × cocaine dose interaction, F12,132 = 6.5, p<0.001; 3.3 vs. 0 mg/kg ESI-05, red *; 10 vs. 0 mg/kg ESI-05, violet *; 3.3 vs. 10 mg/kg ESI-05 black #) and decreased total cocaine intake (two-way repeated-measures ANOVA: ESI-05 treatment, F2,22 = 66.5, p<0.001; cocaine dose, F6,132 = 498.1, p<0.001; ESI-05 × cocaine dose interaction, F12,132 = 6.7, p<0.001; 3.3 vs. 0 mg/kg ESI-05, red *; 10 vs. 0 mg/kg ESI-05 violet *; 3.3 vs. 10 mg/kg ESI-05 black #) on the dose-response curve (n=8–9, 5 male and 4 female). (G–H) ESI-05 dose-dependently decreased the number of cocaine infusions (one-way ANOVA: F2,28 = 16.1, p<0.001; n=10–11, 5 male and 5–6 female) and breakpoint (Brown-Forsythe equal variance: p<0.05; Kruskal-Wallis one-way ANOVA on ranks: ESI-05, H=17.5, p<0.001; n=10–11, 5 male and 5–6 female) under a PR reinforcement schedule. *p<0.05, **p<0.01, ***p<0.001; #p<0.05, ##p<0.01, ###p<0.001 (see also Figure 1—figure supplement 1).
-
Figure 1—source data 1
Raw data shown in Figure 1 and Figure 1—figure supplement 1.
- https://cdn.elifesciences.org/articles/80747/elife-80747-fig1-data1-v2.xlsx
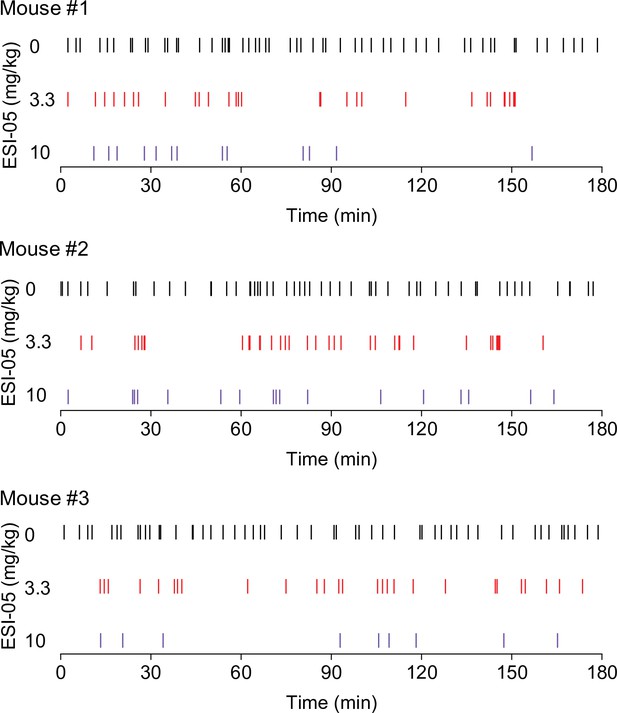
Representative temporal patterns of cocaine infusions in vehicle- and ESI-05-treated mice (associated with Figure 1).
ESI-05 dose-dependently reduced the number of infusions, resulting in sporadic intake with uneven intervals.
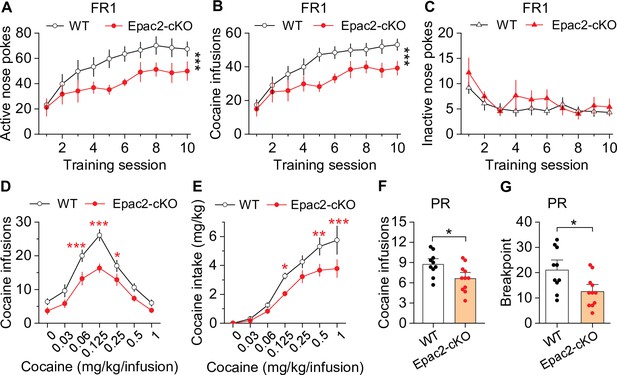
Dopamine neuron-specific knockout of Epac2 impaired cocaine self-administration acquisition and reinforcing efficacy.
(A) Epac2 conditional knockout (Epac2-cKO) mice displayed significantly lower active nose pokes during fixed ratio-1 (FR1) self-administration training (two-way repeated-measures ANOVA: genotype, F1,19 = 8.2, p=0.010; training session, F9,171 = 10.0, p<0.001; genotype × training session interaction, F9,171 = 0.7, p=0.670; n=10–11, 5 male and 5–6 female per group). (B) The number of cocaine infusions during training was significantly reduced by Epac2-cKO (two-way repeated-measures ANOVA: genotype, F1,19 = 8.3, p=0.010; training session, F9,171 = 13.3, p<0.001; genotype × training session interaction, F9,171 = 0.9, p=0.543; n=10–11). (C) There was no significant difference in inactive nose pokes between genotypes (two-way repeated-measures ANOVA: genotype, F 1,19 = 1.4, p=0.247; n=10–11, 5 male and 5–6 female per group). (D–E) Epac2-cKO mice exhibited a downward shift in the cocaine dose-response curve ( two-way repeated-measures ANOVA: genotype, F1,15 = 20.0, p<0.001; cocaine dose, F6,90 = 49.4, p<0.001; genotype × cocaine dose interaction, F6,90 = 2.2, p=0.046; n=8–9, 4–5 male and 4 female per group) and decreased cocaine intake (two-way repeated-measures ANOVA: genotype, F 1,15 = 10.4, p=0.006; cocaine dose, F 6,90 = 62.0, p<0.001; genotype × cocaine dose interaction, F6,90 = 2.5, p=0.025; n=8–9, 4 male and 4–5 female per group). (F–G) Epac2-cKO mice displayed a reduced number of cocaine infusions (t-test: t19=2.6, p=0.019) and lower breakpoint (t-test: t19=2.7, p=0.015) under a progressive ratio (PR) reinforcement schedule (n=9–11, 5 male and 4–6 female per group). *p<0.05, **p<0.01, ***p<0.001 (see also Figure 2—figure supplements 1–4).
-
Figure 2—source data 1
Raw data shown in Figure 2, Figure 2—figure supplement 1, Figure 2—figure supplement 3, and Figure 2—figure supplement 4.
- https://cdn.elifesciences.org/articles/80747/elife-80747-fig2-data1-v2.xlsx
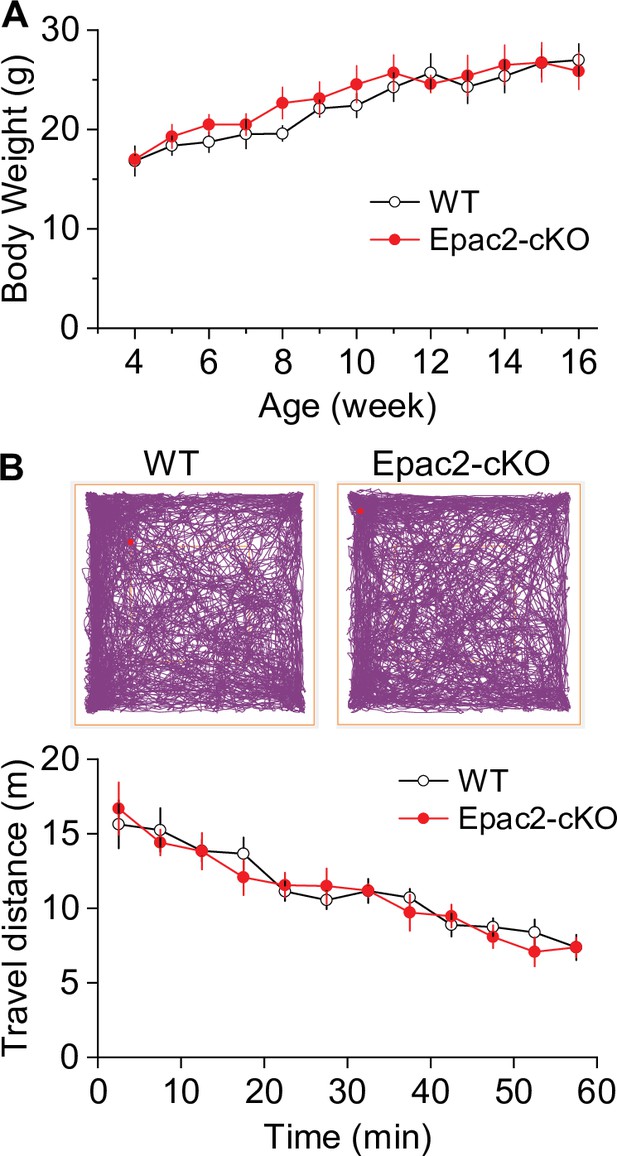
There were no significant differences in body weight and baseline locomotor activity between Epac2 conditional knockout (Epac2-cKO) and wild-type (WT) mice (associated with Figure 2).
(A) Epac2-cKO and WT mice had no significant difference in body weight (t-test: t18=0.4, p=0.667) at 4–16 weeks of age. n=10, 5 male and 5 female per group. (B) During a 1 hr open-field test, Epac2-cKO and WT mice had no significant difference in baseline locomotor activity (two-way repeated-measures ANOVA: genotype, F1,14 = 0.1, p=0.799; time, F11,154 = 21.2, p<0.001; genotype × time interaction, F11,154 = 0.5, p=0.512). n=8, 4 male and 4 female per group.
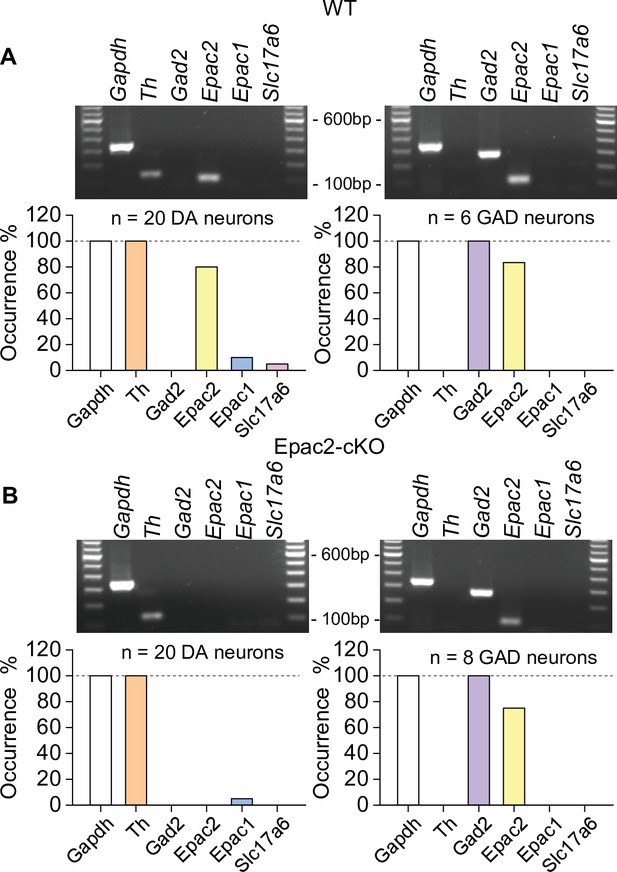
Epac2 was selectively deleted from midbrain dopamine neurons in Epac2 conditional knockout (Epac2-cKO) mice (associated with Figure 2).
(A) In wild-type (WT) mice, Epac2 expression was detected in 80% of dopamine neurons (TH+) and 83% of GABAergic neurons (Gad2+). (B) In Epac2-cKO mice, Epac2 was not detected in any TH+ neurons but was present in 75% of Gad2+ neurons.
-
Figure 2—figure supplement 2—source data 1
Uncropped gels shown in Figure 2—figure supplement 2.
- https://cdn.elifesciences.org/articles/80747/elife-80747-fig2-figsupp2-data1-v2.zip
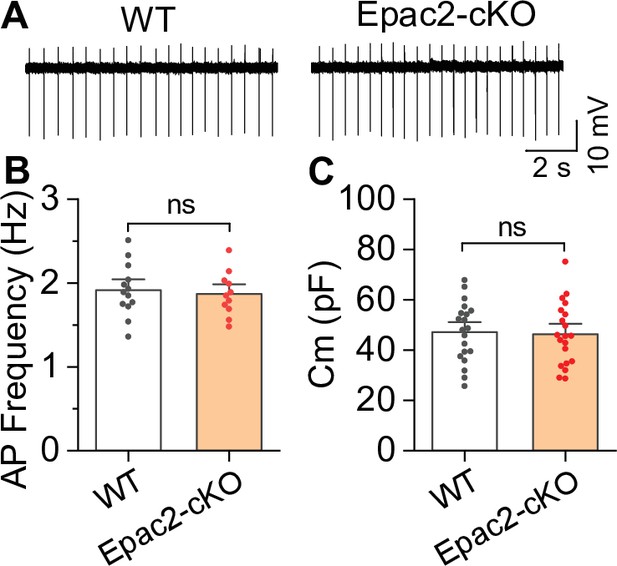
Epac2 conditional knockout (Epac2-cKO) did not alter the frequency of spontaneous action potential firing of ventral tegmental area (VTA) dopamine neurons (associated with Figure 2).
(A–C) The frequency of action potential firing (t-test: t22=0.4, p=0.705; n=11–13 cells from 4 to 5 male and female mice) or membrane capacitance (t-test: t38=0.2, p=0.827; n=20 cells from 4 to 5 male and female mice) in VTA dopamine neurons were not significantly different between wild-type (WT) and Epac2-cKO mice. ns, not significant. p>0.05.
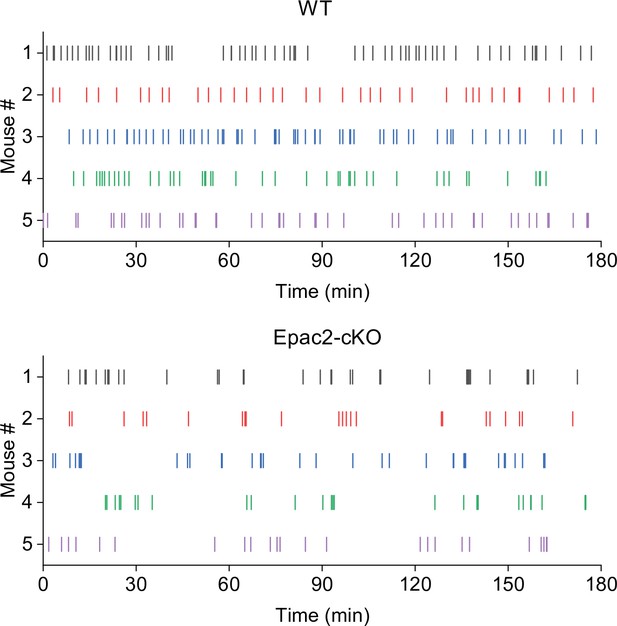
Representative temporal patterns of cocaine infusions in wild-type (WT) and Epac2 conditional knockout (Epac2-cKO) mice (associated with Figure 2).
WT mice show predominantly even-spaced, high-rate cocaine responding, while Epac2-cKO mice exhibited sporadic intake interspersed by long gaps.
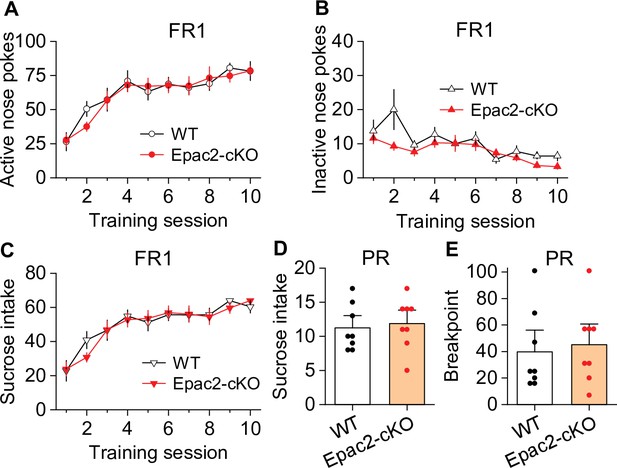
Epac2 conditional knockout (Epac2-cKO) did not affect sucrose self-administration under fixed ratio-1 (FR1) and progressive ratio (PR) reinforcement schedules in mice.
(A–C) Epac2-cKO did not affect the acquisition of sucrose self-administration after 10 days of training under a FR1 schedule (two-way repeated-measures ANOVA: training session on active nose pokes, F9,126 = 20.8, p<0.001; training session on inactive nose pokes, F9,126 = 6.4, p<0.001; training session on sucrose intake, F9,126 = 21.3, p<0.001; genotype on active nose pokes, F1,14 = 0.07, p=0.802; genotype on inactive nose pokes, F1,14 = 3.1, p=0.099; genotype on sucrose rewards, F1,14 = 0.08, p=0.787; training session × genotype interaction in active nose pokes, F9,126 = 0.5, p=0.849; in inactive nose pokes, F9,126 = 1.5, p=0.151; in sucrose intake, F9,126 = 0.6, p=0.8). (D–E) Epac2-cKO did not alter the mean number of sucrose intake (t-test: t14=0.4, p=0.730) or breakpoints (Mann-Whitney rank: U=0.6, p=0.561) under a PR schedule. n=8, 4 male and 4 female per group (see also Figure 3—figure supplement 1).
-
Figure 3—source data 1
Raw data shown in Figure 3, Figure 3—figure supplement 1, and Figure 3—figure supplement 2.
- https://cdn.elifesciences.org/articles/80747/elife-80747-fig3-data1-v2.xlsx
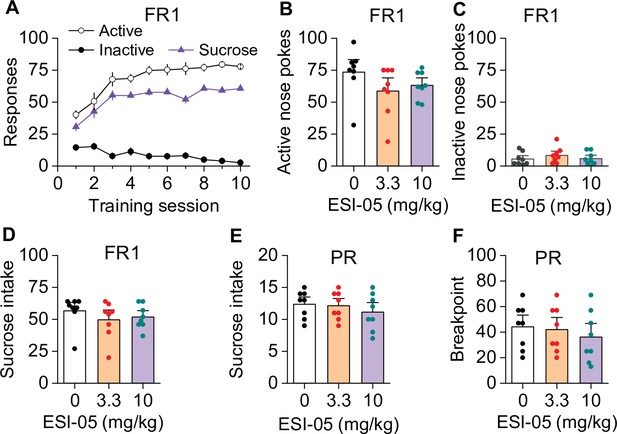
ESI-05 did not affect sucrose self-administration under fixed ratio-1 (FR1) and progressive ratio (PR) reinforcement schedules in mice (associated with Figure 3).
Active and inactive nose pokes and sucrose reinforcers attained during the 10 days of FR1 self-administration training. (B–D) ESI-05 did not affect the mean number of active (one-way ANOVA: F2,21 = 1.65, p=0.217) and inactive nose pokes (one-way ANOVA: F2,21 = 0.67, p=0.525) or sucrose intake (one-way ANOVA: F2,21 = 0.69, p=0.512) under FR1 reinforcement schedule. (E–F) ESI-05 pretreatment did not affect sucrose intake (one-way ANOVA: F2,21 = 0.60, p=0.566) or breakpoint (one-way ANOVA: F2,21 = 0.40, p=0.681) under a PR reinforcement schedule. n=8, 4 male and 4 female per group.
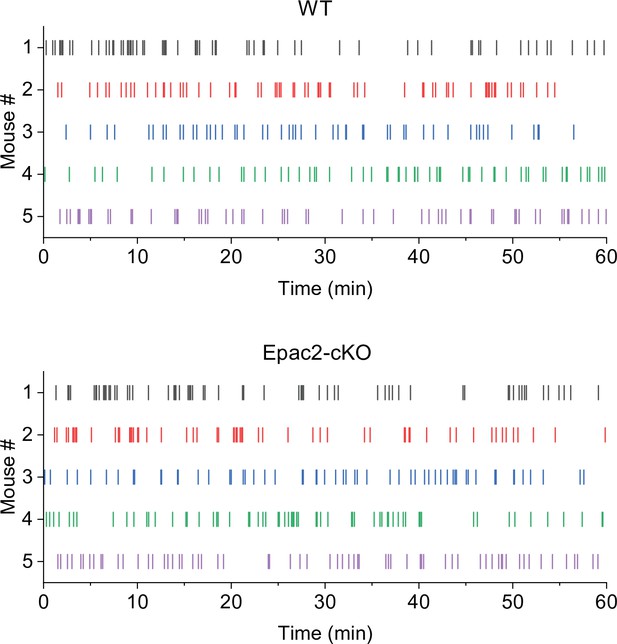
Representative temporal patterns of sucrose intake in wild-type (WT) and Epac2 conditional knockout (Epac2-cKO) mice (associated with Figure 3).
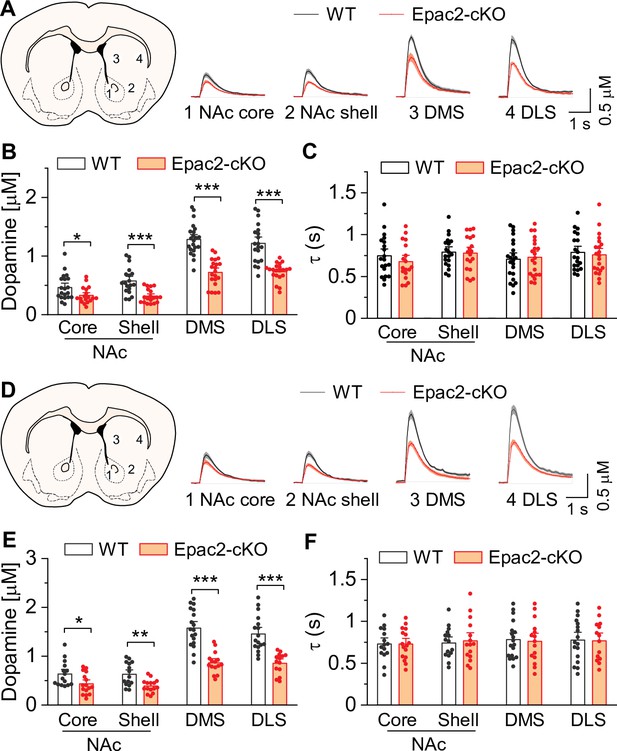
Epac2 knockout decreased evoked dopamine release in the striatum in vitro.
(A) Averaged dopamine concentration vs. time traces from four different striatal subregions (nucleus accumbens [NAc] core, NAc shell, dorsal medial striatum [DMS] and dorsal lateral striatum [DLS]) evoked by a single electrical pulse. (B) Compared to wild-type (WT) mice, Epac2 conditional knockout (Epac2-cKO) mice displayed decreased evoked dopamine release across all striatum subregions in response to single pulse stimulation (t-tests: NAc core, t36=2.3, p=0.028; NAc shell, t36=4.4, p<0.001; DMS, t41=7.4, p<0.001; DLS, t39=6.1, p<0.001; n=18–22 slices from 4 to 5 male and female mice). (C) There were no significant differences in the decay time constants between WT and Epac2-cKO mice with single pulse stimulation across all recording sites (t-tests: NAc core, t36=0.9, p=0.366; NAc shell, t36=0.2, p=0.842; DMS, t41=0.3, p=0.736; DLS, t39=0.4, p=0.676; n=18–22 slices from 4 to 5 male and female mice). (D) Averaged dopamine concentration vs. time traces from four different striatal subregions in response to 100 Hz of 5-pulse electrical stimulation. (E) Compared to WT mice, Epac2-cKO mice displayed decreased evoked dopamine release across all striatum subregions in response to 5-pulse electrical stimulation (t-tests: NAc core, t29=2.4, p=0.025; NAc shell, t28=3.4, p=0.002; DMS, t31=6.3, p<0.001; DLS, t29=5.3, p<0.001; n=14–18 slices from 4 to 5 male and female mice). (F) There were no significant differences in the decay time constants between WT and Epac2-cKO mice with 5-pulse electrical stimulation (t-tests: NAc core, t29=0.006, p=0.999; NAc shell, t28=0.3, p=0.770; DMS, t31=0.3, p=0.801; DLS, t29=0.1, p=0.918; n=14–18 slices from 4 to 5 male and female mice). *p<0.05, **p<0.01, ***p<0.001.
-
Figure 4—source data 1
Raw data shown in Figure 4.
- https://cdn.elifesciences.org/articles/80747/elife-80747-fig4-data1-v2.xlsx
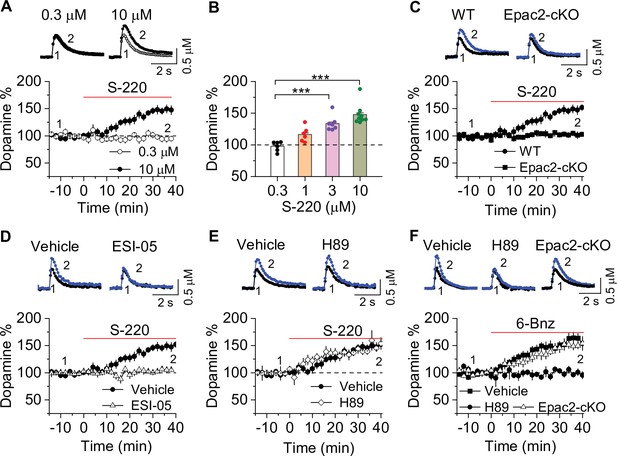
Epac2 activation increased dopamine release in the nucleus accumbens (NAc) in vitro.
(A) Dopamine release was markedly increased by perfusion of S-220 (10 μM) in NAc slices from wild-type (WT) mice (paired t-test; t8=9.1, p<0.001; n=9 slices from 3 to 4 male and female mice). (B) S-220 increased dopamine release in a dose-dependent manner in NAc slices from WT mice (one-way ANOVA: F3,27 = 20.4, p<0.001, n=6–9 slices from 3 to 4 male and female mice). (C) S-220 (10 µM) did not increase dopamine release in NAc slices from Epac2 conditional knockout (Epac2-cKO) mice (paired t-test: t9 = 0.4, p=0.680, p=0.236; n=9 slices from 3 to 4 male and female mice). (D) Preincubation of slices with ESI-05 (20 µM) blocked the S-220-induced increase in dopamine release (t-test: t16=7.4, p<0.001; n=9 slices from 3 to 4 male and female mice). (E) Preincubation of slices with H89 (1 µM) did not alter the S-220-induced increase in dopamine release (t-test: t15 = 1.47, p=0.162; n=8–9 slices from 3 to 4 male and female mice). (F) 6-Bnz (1 µM) increased dopamine release in NAc slices prepared from WT mice (paired t-test: t8=6.5, p<0.001; n=9 slices from 3 to 4 male and female mice). The increase was blocked by H89 (1 µM; t-test: t14 = 5.1, p<0.001; n=7–9 slices from 3 to 4 male and female mice). 6-Bnz also induced an increase in dopamine release in slices from Epac2-cKO mice (paired t-test: t8=6.5, p<0.001; n=8 slices from 3 to 4 male and female mice), which was not significantly different from WT mice (t-test: t15=0.9, p=0.408; n=8–9 slices from 3 to 4 male and female mice). ***p<0.001.
-
Figure 5—source data 1
Raw data shown in Figure 5.
- https://cdn.elifesciences.org/articles/80747/elife-80747-fig5-data1-v2.xlsx
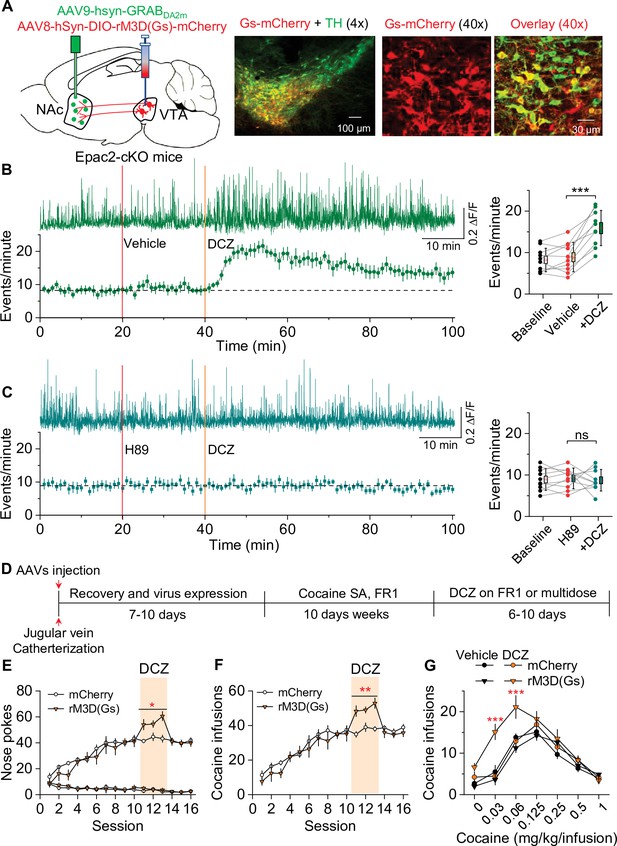
Chemogenetic enhancement of dopamine release increased cocaine self-administration in Epac2 conditional knockout (Epac2-cKO) mice.
(A) AAV8-hSyn-DIO-rM3D(Gs)-mCherry (rM3D(Gs)) or AAV8-hSyn-DIO-mCherry (mCherry) was bilaterally microinjected into the ventral tegmental area (VTA) of Epac2-cKO mice. Immunohistochemistry in VTA sections showed that rM3D(Gs) and mCherry were expressed in the majority of TH+ dopamine neurons but not in TH- neurons. (B) Left, representative dopamine signal and the time course of dopamine transients in the nucleus accumbens (NAc) of Epac2-cKO mice before and after deschloroclozapine (DCZ). Right, DCZ (10 µg/kg, i.p.) significantly increased the frequency of dopamine transients in the NAc shell of Epac2-cKO mice following vehicle pretreatment (paired t-test: t9=7.6, p<0.001; n=10, 5 male and 5 female). (C) DCZ did not affect the dopamine transient frequency in mice which received H89 (1 mg/kg, i.p.) pretreatment (paired t-test: t9=0.1, p=0.889; n=10, 5 male and 5 female). (D) Timeline of AAV injection, cocaine self-administration and DCZ treatment. (E) DCZ pretreatment significantly increased active nose pokes (two-way repeated-measures ANOVA: rM3D(Gs), F1,14 = 12.8, p=0.003; time, F2,28 = 1.9, p=0.164; rM3D(Gs) × time interaction, F2,28 = 1.6, p=0.225) without affecting inactive nose pokes (two-way repeated-measures ANOVA: rM3D(Gs), F1,14 = 0.3, p=0.613; time, F2,28 = 3.0, p=0.065; rM3D(Gs) × time interaction, F2,28 = 1.7, p=0.209) in rM3D(Gs)-expressing Epac2-cKO mice under a fixed ratio-1 (FR1) schedule (n=7–9, 4 male and 3–5 female). (F) DCZ pretreatment significantly increased the number of cocaine infusions (rM3D(Gs), F1,14 = 17.8, p<0.001; time, F2,28 = 3.2, p=0.058; rM3D(Gs) × time interaction, F2,28 = 1.3, p=0.283; n=7–9, 4 male and 3–5 female) under an FR1 schedule in rM3D(Gs)-expressing Epac2-cKO mice. (G) DCZ produced an upward shift in the ascending limb of the cocaine dose-response curve in rM3D(Gs)-expressing Epac2-cKO mice (two-way repeated-measures ANOVA: rM3D(Gs), F3,30 = 8.1, p<0.001; cocaine dose, F6,180 = 79.3, p<0.001; rM3D(Gs) × cocaine dose interaction, F18,180 = 3.6, p<0.001; n=8–9, 4 male and 4–5 female). ns, p>0.05, *p<0.05, **p<0.01, ***p<0.001 (see also Figure 6—figure supplement 1).
-
Figure 6—source data 1
Raw data shown in Figure 6.
- https://cdn.elifesciences.org/articles/80747/elife-80747-fig6-data1-v2.xlsx
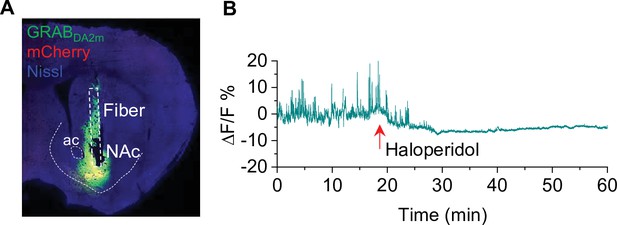
Verification of GRABDA2m injection and activity in the nucleus accumbens (NAc) (associated with Figure 6).
(A) mCherry and GRABDA2m expression in the NAc after viral injection. The cannula implantation was targeted to the virus injection site in the NAc (n = 3 mice). (B) The D2 receptor antagonist haloperidol (0.1 mg/kg, i.p.) abolished GRABDA2m dopamine signals within 10 min of treatment and caused a decrease in baseline GRABDA2m fluorescence. n=3.
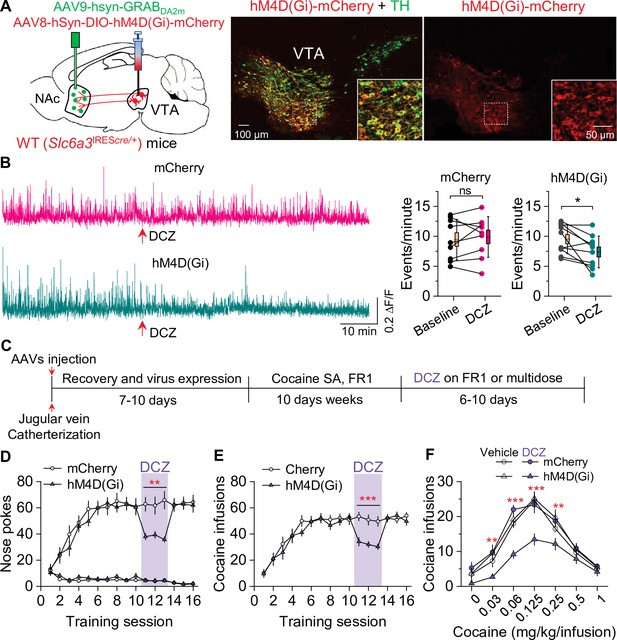
Chemogenetic inhibition of dopamine release decreased cocaine self-administration in wild-type (WT) mice.
(A) AAV8-hSyn-DIO-hM4D(Gi)-mCherry (hM4D(Gi)) or AAV8-hSyn-DIO-mCherry (mCherry) was bilaterally microinjected into the ventral tegmental area (VTA) of WT mice, and the dopamine sensor AAV9-hsyn-DA2m (GRABDA2m) was injected into the NAc shell followed by implantation of a fiber-optic cannula. Immunohistochemistry showed that hM4D(Gi) was expressed in the majority of TH+ dopamine neurons (green) but was not expressed in TH- neurons in the VTA. (B) Left, representative dopamine signal in the nucleus accumbens (NAc) of WT mice before and after deschloroclozapine (DCZ) treatment. Right, DCZ (10 µg/kg, i.p.) did not alter the frequency of dopamine transients in mice with mCherry expression (paired t-test: t8=0.7, p=0.514; n=9, 5 male and 4 female). The frequency of dopamine transients was significantly decreased after DCZ treatment in WT mice expressing hM4D(Gi) in VTA dopamine neurons (paired t-test: t9=2.9, p=0.017; n=10, 5 male and 5 female). (C) Timeline of cocaine self-administration and DCZ treatment. (D–E) DCZ pretreatment significantly decreased active nose pokes (two-way repeated-measures ANOVA: hM4D(Gi), F1,15 = 18.6, p<0.001; time, F2,30 = 0.03, p=0.966; hM4D(Gi) × time interaction, F2,30 = 2.5, p=0.096) and cocaine infusions (two-way repeated-measures ANOVA: hM4D(Gi), F1,15 = 25.7, p<0.001; time, F2,30 = 2.2, p=0.132; hM4D(Gi) × time interaction, F2,30 = 0.02, p=0.986) without altering inactive nose pokes (hM4D(Gi), F1,15 = 0.01, p=0.911; time, F2,30 = 0.5, p=0.627; hM4D(Gi) × time interaction, F2,30 = 0.9, p=0.402) in hM4D(Gi)-expressing WT mice (n=8–9, 4 male and 4–5 female). (F) DCZ produced a significant downward shift of the cocaine dose-response curve (two-way repeated-measures ANOVA: hM4D(Gi), F3,26 = 13.8, p<0.001; cocaine dose, F6,156 = 100.2, p<0.001; hM4D(Gi) × cocaine dose interaction, F18,156 = 2.2, p=0.006; n=7–8, 4 male and 3–4 female) in WT mice that expressed hM4D(Gi) in VTA dopamine neurons. ns, p>0.05, *p<0.05, **p<0.01, ***p<0.001.
-
Figure 7—source data 1
Raw data shown in Figure 7.
- https://cdn.elifesciences.org/articles/80747/elife-80747-fig7-data1-v2.xlsx
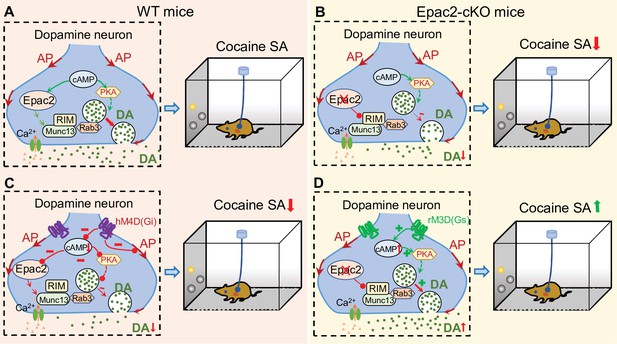
A hypothetical model that explains the relationship between Epac2 disruption, decreased dopamine release, and impairment of cocaine self-administration.
(A) Epac2 is known to interact with the active zone proteins RIM, Munc13, and Rab3. A potential interaction of Epac2 with active zone proteins in the axonal varicosity of dopamine neurons facilitates dopamine exocytosis, which supports cocaine reinforcement during self-administration (SA). (B) Epac2 conditional knockout (Epac2-cKO) mice led to decreased dopamine release and cocaine SA. (C) hM4D(Gi)-mediated inhibition of action potential (AP) firing and dopamine release from axonal terminals of ventral tegmental area (VTA) dopamine neurons in WT mice led to a reduction of cocaine self-administration (mimicking the effect of Epac2-cKO). (D) Enhancing dopamine release via rM3D(Gs) activation in VTA dopamine neurons increases dopamine release via the PKA pathway and enhances cocaine self-administration in Epac2-cKO mice.
Tables
Reagent type (species) or resource | Designation | Source or reference | Identifiers | Additional information |
---|---|---|---|---|
Strain, strain background (Mus musculus) | C57BL/6J mice | The Jackson Laboratory | Stock#: 000664 | |
Genetic reagent (Mus musculus) | Heterozygous Slc6a3IREScre/+ mice | The Jackson Laboratory | Stock#: 006660 | Maintained on the C57BL/6J background |
Strain, strain background (Adeno-associated virus) | AAV9-hSyn-GRABDA2m | WZ Biosciences | ||
Strain, strain background (Adeno-associated virus) | AAV8-hSyn-mCherry | Addgene | Catalog#: 114472-AAV8 | |
Strain, strain background (Adeno-associated virus) | AAV8-hSyn-DIO-rM3D(Gs)-mCherry | Addgene | Catalog#: 50458-AAV8 | |
Strain, strain background (Adeno-associated virus) | AAV8-hSyn-DIO-hM4D(Gi)-mCherry | Addgene | Catalog#: 44362-AAV8 | |
Strain, strain background (Adeno-associated virus) | AAV8-hSyn-DIO-mCherry | Addgene | Catalog#: 50459-AAV8 | |
Antibody | Rabbit polyclonal anti-TH | Santa Cruz Biotechnology | SC-14007, Lot: C2707 | 1:300 |
Antibody | Goat anti-rabbit IgG Alexa Fluor-488 | Cell Signaling | Stock#: 4412 | 1:300 |
Other | NeuroTrace 640/660 Deep-Red Fluorescent Nissl Stain | Thermo Fisher Scientific | N-21483 | 1:150 |
Software, algorithm | Origin 2022b | OriginLab Corporation | ||
Software, algorithm | RStudio | RStudio, PBC | DIO Link (Github): GitHub - xiaojieliu17/Fiber-photometry |