The layered costs and benefits of translational redundancy
Figures
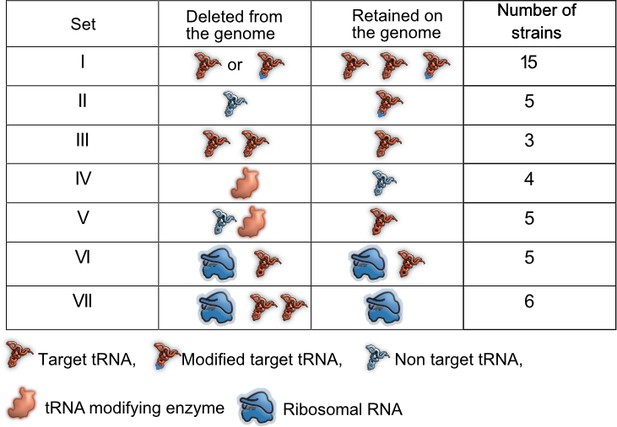
Summary of experimental manipulation of redundancy in translational components.
Target transfer RNAs (tRNAs): tRNA isotypes that are targets of modifying enzymes (MEs) (i.e. are post-transcriptionally modified by a tRNA modifying enzyme), expanding the repertoire of codons they can read. Non-target tRNAs: tRNA isotypes that are not acted upon by MEs. The codons recognized by these tRNAs can also be read by modified target tRNAs, rendering the non-target tRNAs redundant. Symbols represent qualitative differences across sets rather than the exact number or diversity of redundant components. Further details of strains in each set are given in Supplementary file 1A.
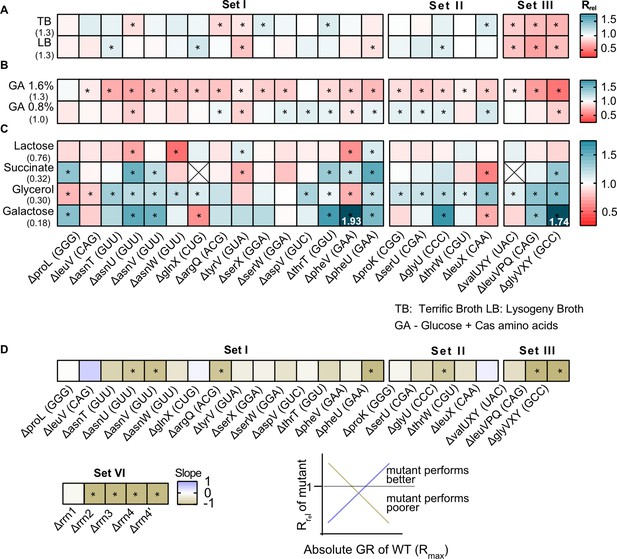
The fitness impact of loss of transfer RNA (tRNA) redundancy varies with nutrient availability.
(A–C) Heat maps show the relative growth rate of tRNA gene deletion strains (‘mutant’) (Rrel = GRmutant/GRWT) in different growth media (see Figure 2—source data 1 file for raw data and Supplementary file 2 for statistics). The anticodon encoded within each deleted tRNA gene is indicated in parentheses on the x-axis. The absolute exponential growth rate (doublings/hour) of WT in each medium is indicated in parentheses on the y-axis. Box colors indicate the impact of each gene deletion (red: Rrel <1, mutant grows more slowly than WT; blue: Rrel >1, mutant grows faster than WT; n=3–4 biological replicates per strain per medium); statistically significant differences from WT are indicated by asterisks (ANOVA with Dunnet’s correction for multiple comparisons). Boxes with an ‘X’ indicate cases where strains failed to grow exponentially. Panels show Rrel in (A) complex rich media (B) permissive rich media (M9 salts supplemented with indicated concentrations of glucose and cas amino acids) (C) poor minimal media (M9 salts) with the indicated carbon source but no cas amino acids. In two cases, we observed very high Rrel values in galactose and these are indicated numerically. (D) For each mutant we estimated Spearman’s rank correlation between the growth rate impact of the gene deletion (Rrel) and the respective maximal wild type (WT) growth rate (Rmax) across eight growth media (Figure 2—figure supplement 5). The heat map shows the slope of this correlation for each mutant. Darker colors indicate a stronger negative relationship, i.e., a higher cost of redundancy in poor media. Statistically significant non-zero slope values are indicated by asterisks. Data for mutants with rRNA operon deletions (across six growth media) are also included in this panel (see Figure 6B).
-
Figure 2—source data 1
Data associated with Figure 2.
- https://cdn.elifesciences.org/articles/81005/elife-81005-fig2-data1-v2.xlsx
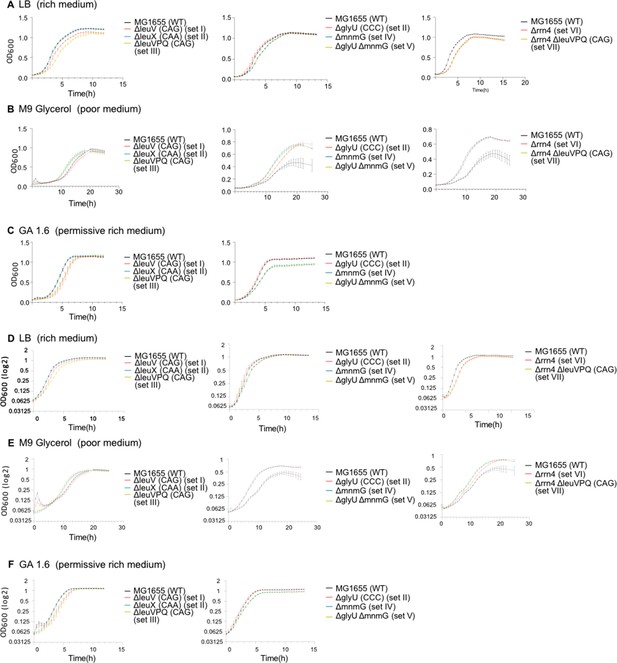
Growth curves (OD600 vs. time) for representative strains in rich and poor media.
Raw growth curves are shown for one strain from each mutant set (see Supplementary file 1A and Figure 1). (A) Rich medium (LB, Lysogeny Broth) (B) permissive rich medium (GA1.6, M9 salts +1.6% glucose +1.6% cas amino acids) (C) poor medium (M9 glycerol, M9 salts +0.3% glycerol). Error bars indicate SE across replicates (n=4 per strain per medium). Same growth curves with OD600 on Log2 scale, are shown in Figure D-F.
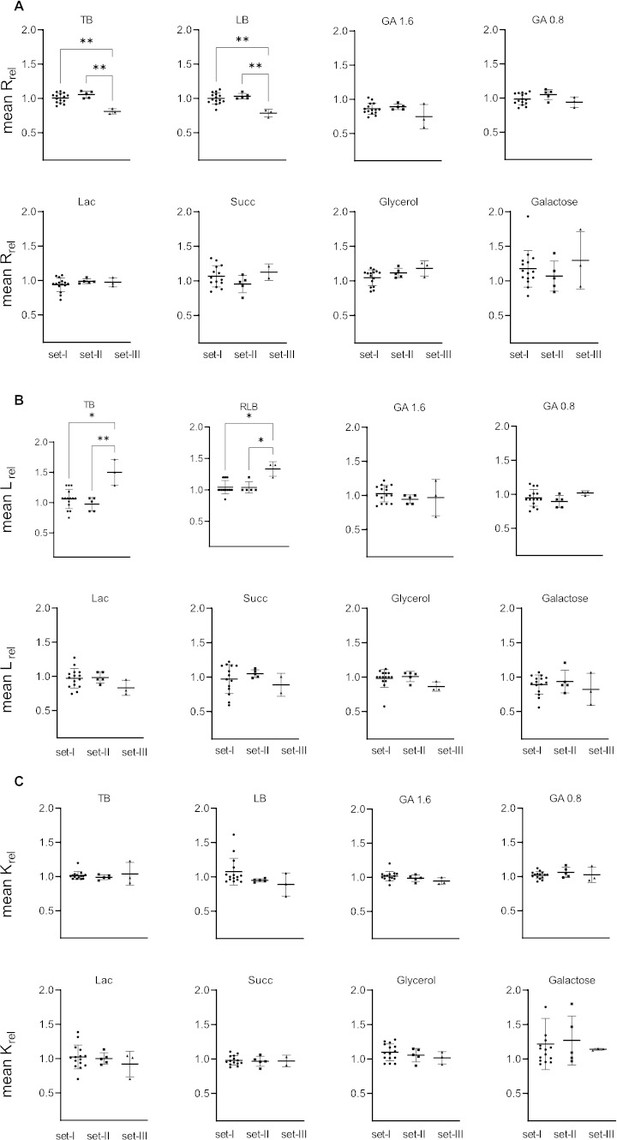
Mean relative growth rate (Rrel), length of lag phase (Lrel), and yield (Krel) of mutant strains across all tested media.
Impact of transfer RNA (tRNA) deletion on growth parameters (y-axis) for all strains from set I, II and III (on x-axis; see Figure 1, Supplementary file 1A), shown as the ratio of mutant:WT for each parameter. Each data point is the mean of four replicates; the horizontal bar indicates the mean of means of that set. (A) Relative growth rate (B) relative length of lag phase (C) relative growth yield. Error bars indicate SE of the mean across strains in each set (n=number of strains as indicated in Figure 1, Supplementary file 1A). Significantly different pairs (Kruskal–Wallis one-way ANOVA) are showed with asterisks.
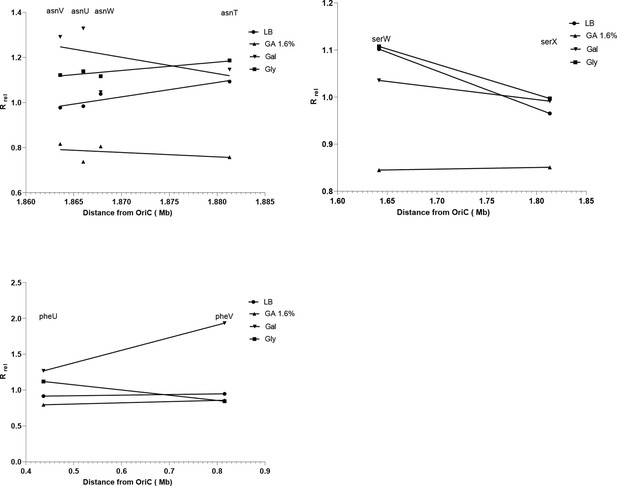
Correlation between distance from oriC and fitness effect of transfer RNA (tRNA) gene deletion.
In our dataset, we had three cases where deleting copies of the same gene (four copies of Asn-tRNAGUU and two copies each of Ser-tRNAGGA) showed slightly different fitness phenotypes. To test whether the differences arose due to their distance from oriC, we tested for a correlation between Rrel of each single gene deletion mutant in the two richest and the two poorest media, and the shortest distance from oriC for each gene on the E. coli MG1655 genome (RefSeq: NC_000913). In each case, we did not find a significant correlation (p>0.05).
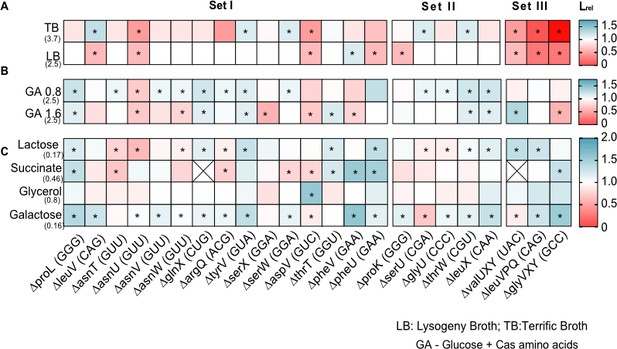
Impact of transfer RNA (tRNA) deletion on length of the lag phase.
Impact of tRNA gene deletion on the length of lag phase (L), in different media, relative to wild type (WT) (Lrel = LdeltRNA/LWT). The anticodon of each deleted tRNA gene is indicated in parentheses on the x-axis, and strains are categorized into sets as described in Supplementary file 1A and Figure 1. Box colos indicate the effect of gene deletion (red: Lrel >1, mutant has longer lag phase than WT; blue: Lrel <1, mutant has shorter lag phase than WT; n=3–4 replicates per strain per medium). Asterisks indicate cases where the mutant has a significantly different lag phase than WT (ANOVA with Dunnett’s correction for multiple comparisons). The absolute lag length (hours) of WT in each medium is indicated in parentheses on the y-axis. From top to bottom, panels show yield in (A) complex rich media (B) permissive rich media, with indicated concentrations of glucose and cas amino acids (C) poor (M9) minimal media with the indicated carbon source but no cas amino acids.
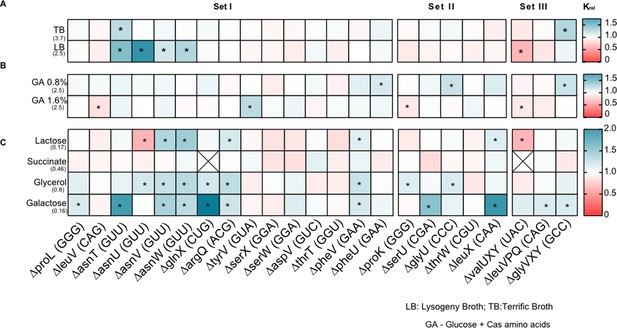
Fitness impact of transfer RNA (tRNA) deletions on growth yield.
Impact of tRNA gene deletion on growth yield (K), in different media, relative to wild type (WT) (Krel = KdeltRNA/KWT). The anticodon of each deleted tRNA gene is indicated in parentheses on the x-axis, and strains are categorized into sets as described in Supplementary file 1A and Figure 1. Box colors indicate the effect of gene deletion (red: Krel >1, mutant has higher yield than WT; blue: Krel <1, mutant has lower yield than WT; n=3–4 replicates per strain per medium). Asterisks indicate cases where the mutant has a significantly different yield than WT (ANOVA with Dunnett’s correction for multiple comparisons). The absolute yield (max OD600) of WT in each medium is indicated in parentheses on the y-axis. From top to bottom, panels show yield in (A) complex rich media (B) permissive rich media, with indicated concentrations of glucose and cas amino acids (C) poor (M9) minimal media with the indicated carbon source but no cas amino acids.
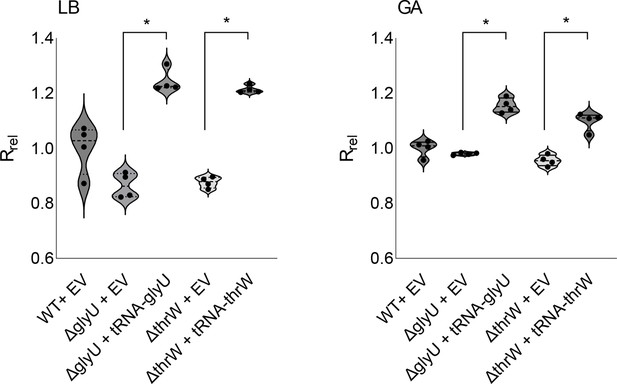
Rescue of select transfer RNA (tRNA) deletion mutants by gene complementation from a plasmid.
Relative growth rate of two tRNA deletion mutants transformed with a plasmid (pUC19) carrying the respective tRNA gene. EV = Empty vector control. Cells were grown in the presence of 100 µg/ml Ampicillin (to ensure plasmid retention) and 0.5 mM IPTG to induce tRNA gene expression from the plasmid. The relative growth rate was measured as described in the methods section, in LB (left panel) and GA (Glucose Cas amino acid) (right panel). Supplementation of tRNA from a plasmid increases the growth rate of both mutants (N=4, Mann-Whitney U test). Horizontal bars indicate the median and quartiles.
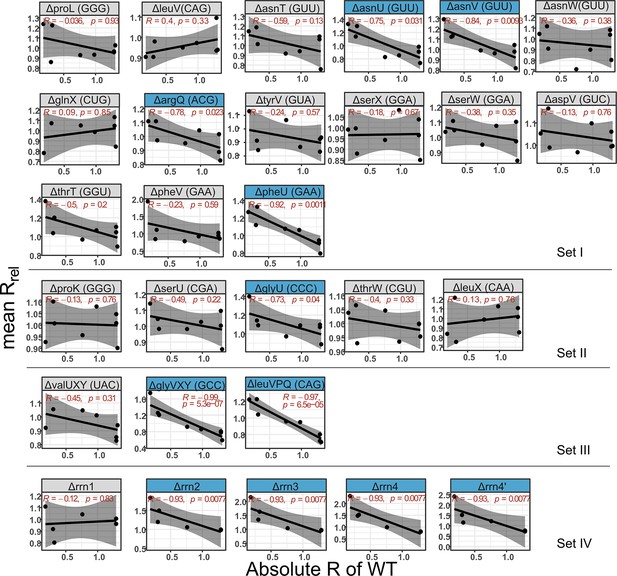
Correlation between absolute growth rate of the wild type (WT) and relative growth rate of mutants.
Plots show Spearman’s rank correlation between the growth rate impact of each gene deletion across different media (Rrel) and the respective maximal WT growth rate (Rmax). Strains with statistically significant non-zero slope values are highlighted in blue. Shaded regions around lines indicate 95% confidence intervals.
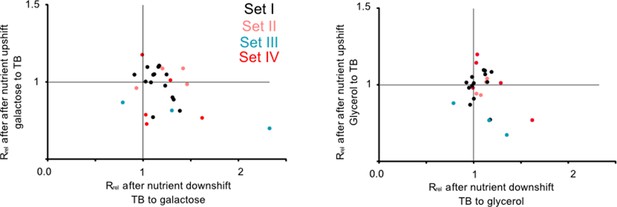
Redundant transfer RNA (tRNA) gene copies are beneficial during nutrient fluctuations.
Relative growth rate of mutants (sets I to IV, Figure 1) upon a nutrient downshift (Rrel on the x-axis) and an upshift (Rrel on the y-axis). Rrel was calculated relative to wild type (WT) (growing in identical conditions), as described in Figure 2 (see Figure 3—source data 1 file for raw data and Supplementary file 2 for statistics). Strains were transferred between a rich medium (TB, terrific broth) and a poor medium (M9 salts +0.6% glycerol or 0.05% galactose). Each data point represents the mean Rrel for a mutant (n=4 biological replicates), with the color indicating the set to which it belongs (Figure 1). Mutants in the bottom right quadrant perform better under a nutrient downshift (i.e. when moving from a rich to poor medium), but more poorly than WT under a nutrient upshift (i.e. moving from a poor to rich medium). Mutants in the top right quadrant perform better than WT in both types of nutrient fluctuations. Statistics are reported in Supplementary file 2.
-
Figure 3—source data 1
Data associated with Figure 3.
- https://cdn.elifesciences.org/articles/81005/elife-81005-fig3-data1-v2.xlsx
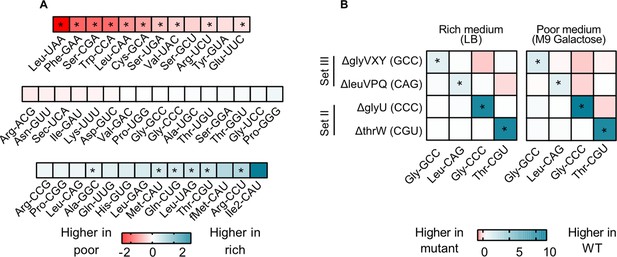
transfer RNA (tRNA) gene copy number changes confer stronger control over the proportional contribution of tRNA isotypes to the mature tRNA pool, than does tRNA gene regulation.
The proportional contribution of all 42 tRNA isotypes in the mature tRNA pool was measured for wild type (WT) and four tRNA deletion mutants in a poor medium (M9 +0.05% galactose) and a rich medium (LB) (n=3 biological replicates per medium per strain) using YAMAT-seq (see Figure 4—source data 1 file for raw data and Supplementary file 2 for statistics). The change in tRNA proportions across strains or across media was then calculated as mean log2 fold change with respect to the ‘reference’ as indicated in each comparison. Significant differences are shown with asterisks (pairwise Wald tests with Benjamini-Hochberg correction for multiple comparisons; Supplementary file 2). (A) The relative proportion of all WT tRNA isotypes in poor medium, compared to rich medium. Isotype is indicated on the x-axis. Blue indicates a higher proportional contribution in rich medium, and red indicates a higher contribution in poor medium. (B) The impact of tRNA gene deletion on the proportions of focal isotype tRNAs (indicated on the x-axis), in WT vs. mutant strains (tRNA gene deletions are indicated on the y-axis), in a rich and a poor medium. Darker blue colors indicate lower proportional contributions in the mutant, and darker red indicates higher proportional contributions compared to WT.
-
Figure 4—source data 1
Data associated with Figure 4.
- https://cdn.elifesciences.org/articles/81005/elife-81005-fig4-data1-v2.xlsx
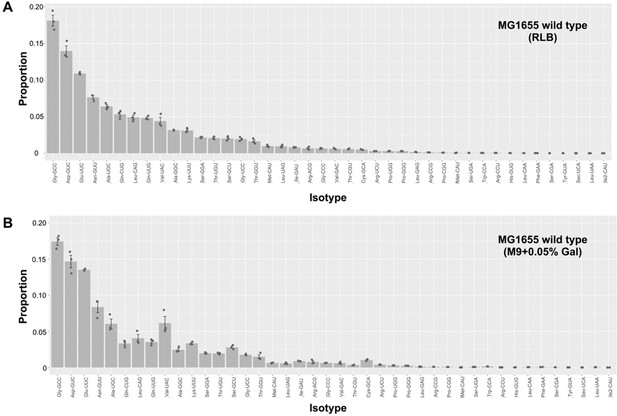
Proportional contribution of transfer RNA (tRNA) isotypes to the total tRNA pool in wild type (WT) E. coli in different media.
Bar plots show the proportions of 42 tRNA isotypes measured in the mature tRNA pool of E. coli K-12 MG1655 (WT) in (A) rich (LB) and (B) poor (M9 +0.05% galactose) media. Isotypes are ordered from highest (tRNA-Gly(GCC)) to lowest (tRNA-Ile2(CAU)) in rich medium; y-axes scales are the same for both figures. Bars are means of three replicates, with individual replicates shown as gray dots. Error bars are +/-1 standard error.
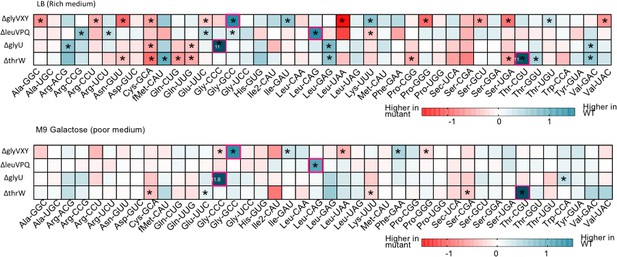
Impact of transfer RNA (tRNA) deletion on the composition of the tRNA pools in rich and poor media.
Proportional abundance of tRNA isotypes (mean log2 fold change, n=3 per medium per strain) in mutant tRNA pools compared to wild type (WT) tRNA pools. Differential expression of focal tRNAs (indicated on the x-axis) in WT vs. each mutant (tRNA deletion strains indicated on the y-axis), in (A) rich (LB) and (B) poor medium (M9 glycerol). Darker blue colors indicate lower proportions in mutant (compared to WT), and darker red indicates relatively higher proportions. Significant differences are indicated by asterisks. Where proportions are more extreme than indicated in the color key, the fold change value is noted in the box. Anticodons carried by the tRNA gene deleted in each deletion strain are indicated by pink borders.

Loss of redundant transfer RNA (tRNA) genes decreases translation output during rapid growth, but increases translational output in poor media.
The heat map shows the translation capacity of tRNA gene deletion mutants in rich (LB), permissive (M9 +0.8% GA) and poor medium (M9 +0.6% glycerol), measured as the relative protein elongation rate (ER, increase in the length of ß-galactosidase protein per unit time after induction; ERrel = ERmutant/ERWT; n=2 biological replicates per strain per medium, see Figure 5—source data 1 file for raw data and Supplementary file 2 for statistics). Red indicates lower reporter protein production in the mutant per unit cell density (i.e. reduced translation capacity), and blue indicates increased translation capacity relative to wild type (WT). Significant differences are indicated with asterisks (ANOVA with Dunnet’s correction for multiple comparisons).
-
Figure 5—source data 1
Data associated with Figure 5.
- https://cdn.elifesciences.org/articles/81005/elife-81005-fig5-data1-v2.xlsx
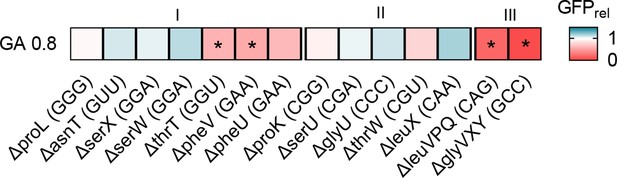
Impact of transfer RNA (tRNA) deletions on translation capacity.
Translation capacity of selected tRNA deletion strains in permissive medium (GA0.8), measured as GFP readout per unit time after induction, relative to WT (n=2–3 per strain; GFPrel = GFPmutant/GFPWT). Red indicates lower production of reporter protein in the deletion strain per unit cell density (i.e. reduced translation capacity), and blue indicates increased translation capacity relative to wild type (WT). Significant differences between mutant and WT are indicated by asterisks.
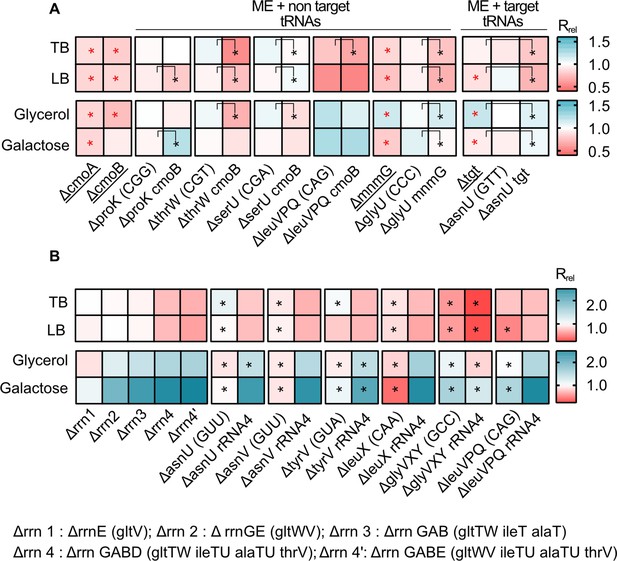
Impacts of manipulating redundancy in multiple translation components are highly variable.
Impact of transfer RNA (tRNA), rRNA and modifying enzyme (ME) gene deletion on growth rate in different media, as described in Figure 2. Box colors indicate the impact of gene deletion on growth rate relative to wild type (WT) (red: Rrel <1, mutant grows more slowly than WT; blue: Rrel >1, mutant grows faster than WT; n=3–4 biological replicates per strain per medium, see Figure 6—source data 1 file for raw data and Supplementary file 2 for statistics). Panels show Rrel for (A) co-deletion of MEs and tRNA genes. The anticodon of each deleted tRNA gene is indicated in parentheses on the x-axis. MEs were either deleted alone (underlined strains) or co-deleted with the respective non-target and target tRNAs. ME deletions strains were compared with the WT to establish statistically significant differences, indicated with a red asterisk. For co-deletions of MEs and non-target tRNAs, comparisons are shown between non-target tRNA deletion and ME +non target tRNA codeletion (as indicated by the asterisks and vertical square brackets). For co-deletions of MEs and target tRNA, comparisons are shown between ME deletions and ME +target tRNA co-deletion (as indicated by the asterisks and vertical square brackets). Other statistical comparisons, not shown in the figures, are in Supplementary file 2. (B) Co-deletion of rRNAs and tRNAs. rRNA operons are abbreviated as shown in the key; tRNA genes that were deleted as part of the operon are indicated in parentheses. Additional tRNAs (outside the rRNA operon) that were deleted in combination with rrn4 are indicated on the x-axis. Asterisks indicate statistically significant impacts ΔrRNA4 vs. ΔrRNA4+tRNA deletion, (ANOVA with Dunnet’s correction for multiple comparisons, Supplementary file 2).
-
Figure 6—source data 1
Data associated with Figure 6.
- https://cdn.elifesciences.org/articles/81005/elife-81005-fig6-data1-v2.xlsx
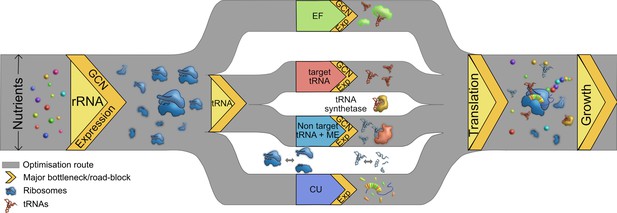
Multiple routes to translational adaptation.
Proposed highway model for the layered, hierarchical organisation of translation components showing multiple potential routes for optimizing translational output, based on the current study and previous work. The width of the routes is constrained primarily by available nutrients, which sets an upper limit to translational output; and the redundancy of various components evolves under this constraint. Within the confines of what is achievable under given nutrient conditions, different components may adapt in different order, and to different degrees. Important components that serve as bottlenecks are shown in yellow, from left to right in the proposed order of importance. rRNA should adapt first (via gene expression change in the short term and gene copy number, GCN, over long term), contributing to ribosome synthesis. Next, transfer RNA (tRNAs) may adapt via two possible parallel routes: by modulating the copy number or expression of target tRNAs, or non-target tRNA +MEs (see Introduction). Elongation factors (EF) can adapt by similar routes. Simultaneously, a stronger match between the tRNA pools and codon usage (CU) of highly expressed genes will enhance translational output. Components that are not directly tested or discussed extensively here but are likely to adapt via the general principles derived here are shown in the white spaces: tRNA synthetases and ribosomal and tRNA disassembly/degradation. Together, all these factors determine translation output, growth, and fitness.
Additional files
-
Supplementary file 1
Detailed information on primers and strains used in this study, and the genomic location of tRNA genes.
- https://cdn.elifesciences.org/articles/81005/elife-81005-supp1-v2.xlsx
-
Supplementary file 2
Summary statistics for analyses associated with main figures.
- https://cdn.elifesciences.org/articles/81005/elife-81005-supp2-v2.xlsx
-
Supplementary file 3
Summary of YAMAT-seq data.
- https://cdn.elifesciences.org/articles/81005/elife-81005-supp3-v2.xlsx
-
MDAR checklist
- https://cdn.elifesciences.org/articles/81005/elife-81005-mdarchecklist1-v2.docx