Microstructural and crystallographic evolution of palaeognath (Aves) eggshells
Figures
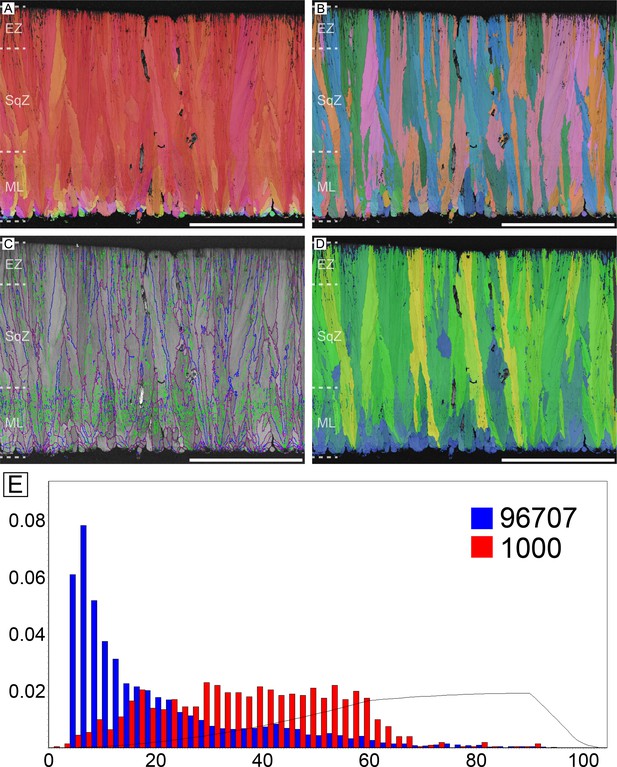
Ostrich eggshell.
(A) IPF, (B) Euler, (C) GB, and (D) AR mappings (see Figures 13—15 for legends). The dashed lines in the maps mark the boundary between the ML and SqZ; SqZ and EZ. Scale bars equal 1000 µm. (E) A misorientation histogram. The numbers in x- and y-axis represent degree between the two selected grains (either adjacent [blue; neighbour-pair method] or random [red; random-pair method]; see also Figure 16) and frequency, respectively. The numbers at the upper right corner mean the number of selected grains in each selection method. The explanation herein is applicable to the Figures 2—12, Appendix 3—figures 1–5, and Appendix 4—figures 1–4.
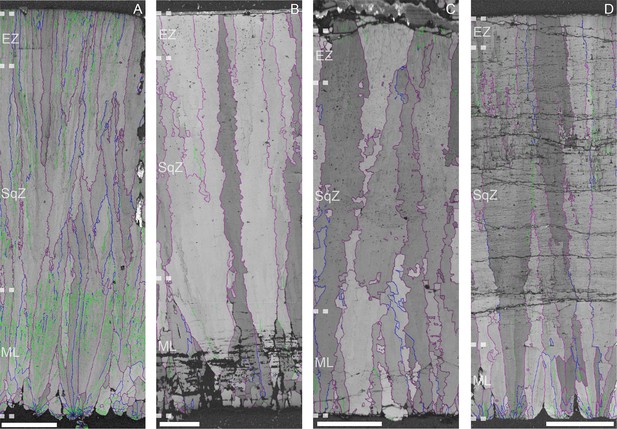
Composite GB mappings of fossil and modern eggshells that have prismatic shell unit structure.
(A) Ostrich, (B) Prismatoolithus levis, (C) Triprismatoolithus stephensi, and (D) thick moa eggshell. In P. levis and T. stephensi (B,C), GB becomes slightly more linear in outer parts of eggshell, which have been suggested as EZ (Varricchio and Jackson, 2004; Jackson and Varricchio, 2010). In ostrich and thick moa eggshell (A,D), the difference in linearity is much more subtle. Scale bars equal 250 µm (A); 100 µm (B–D).
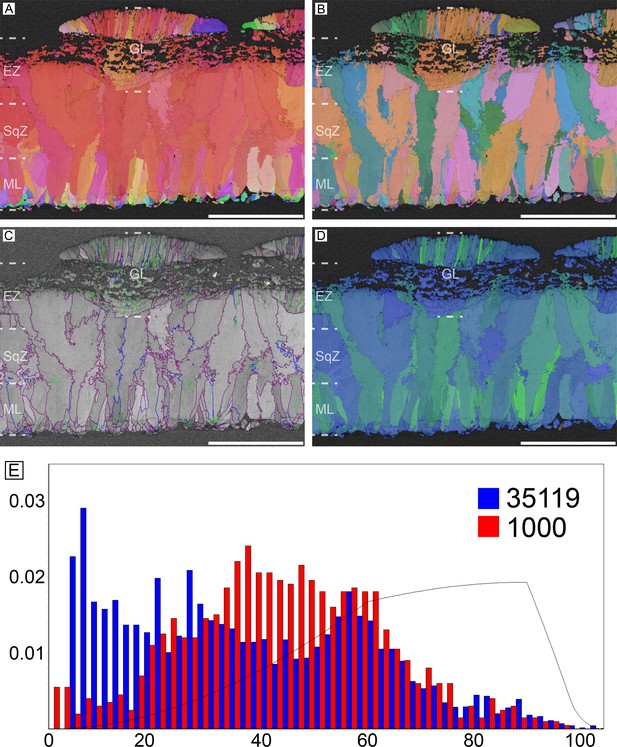
Emu eggshell.
Note that deposition of granular layer (GL) begins in the EZ. Scale bars equal 500 µm.
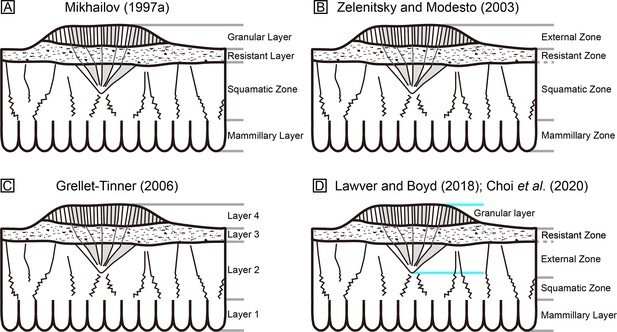
Previous and current interpretations on the microstructure of casuariid eggshells.
(A,C) Mikhailov, 1997a and Grellet-Tinner, 2006 suggested four layers. (B) Zelenitsky and Modesto, 2003 suggested that compact calcified part of the eggshell is composed of SqZ and Resistant Zone is an extension of SqZ. In their view, ornamentation is a homologous structure to EZ that is usually present in all avian eggshell (except for passerine; Mikhailov, 1997a). (D) Lawver and Boyd, 2018 pointed out that ornamentation has its ‘root’ inside compact calcified eggshell. It was confirmed in Choi et al., 2020 and this study. In addition, based on the GB condition of EZ, which is characterized by linear line, Choi et al., 2020 further suggested that outer compact calcified part of the eggshell is EZ, not SqZ as suggested by Zelenitsky and Modesto, 2003. In our view, therefore, ornamentation is not homologous to EZ of most avian eggshells, but is an autapomorphic character of MRCA of emu and cassowary.
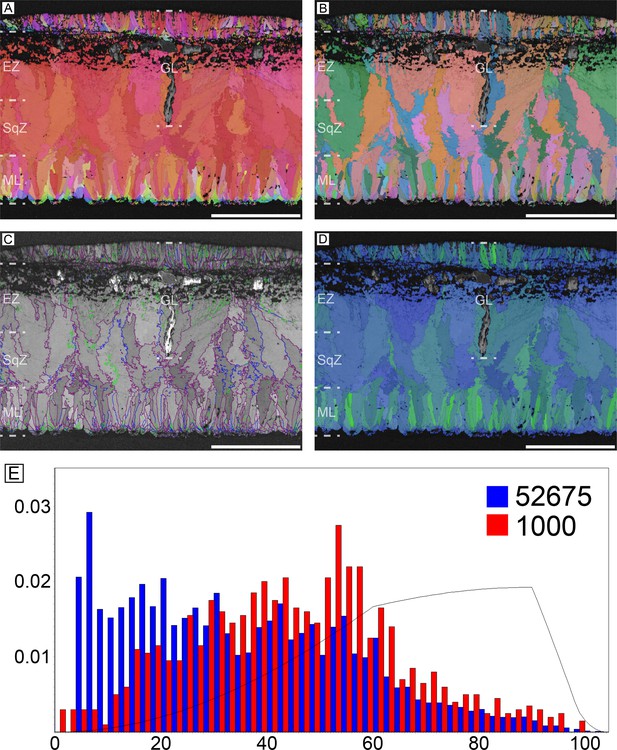
Cassowary eggshell.
Note that deposition of granular layer (GL) begins in the middle of SqZ. Scale bars equal 500 µm.
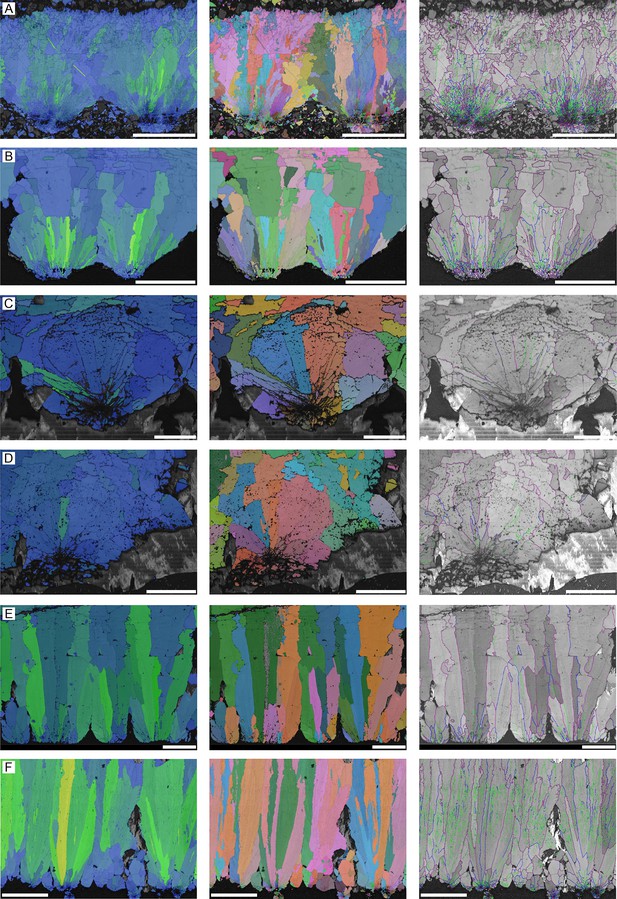
Mammillary layers of (A) Reticuloolithus, (B) Elongatoolithus, (C) tinamou, (D) kiwi, (E) thick moa, and (F) ostrich eggshells presented in AR, Euler, and GB mappings.
When ML is confined to certain region of eggshell (A–D) AR mappings effectively show the needle-like calcite grains, which is a useful character state for fossil eggshell classification. However, when the calcite grain in ML is not limited within the ML, even wedge-like ML can have high AR (E,F). In these cases, measuring the density of calcite grains in the ML can provide a quantitative approach to diagnose needle-like versus wedge-like states in ML. Scale bars equal 250 µm (A,F); 100 µm (B,E); 50 µm (D); 25 µm (C).
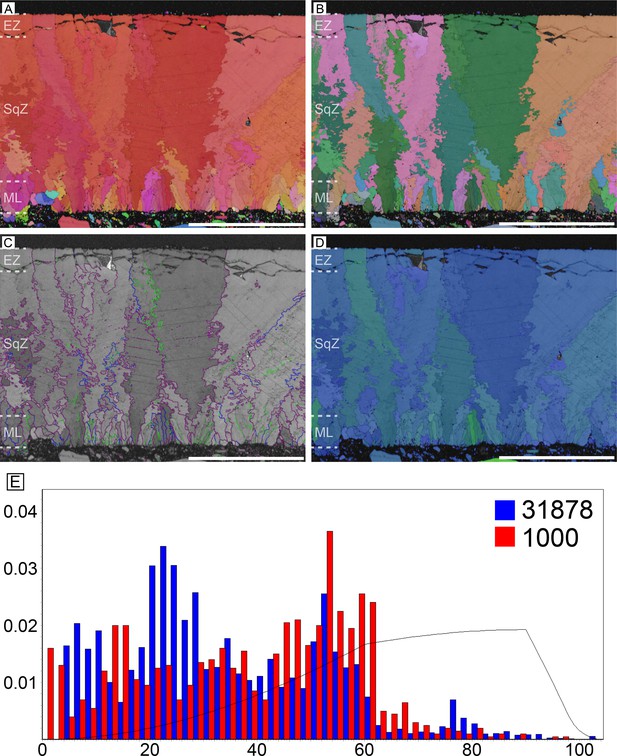
Lithornis eggshell (see also Houde, 1988; Grellet-Tinner and Dyke, 2005).
Scale bars equal 250 µm.
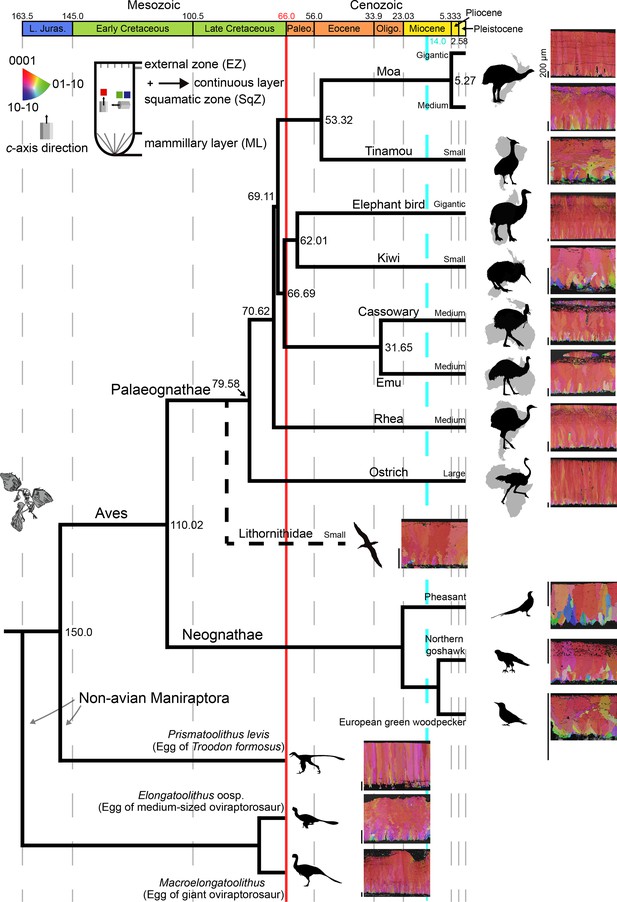
IPF mappings of palaeognath, neognath, and non-avian maniraptoran eggshells.
The phylogeny and speciation timelines of palaeognath are based on Yonezawa et al., 2017 and Bunce et al., 2009; branching in neognath and non-avian Maniraptora are arbitrary, not reflecting specific time. The speciation time of Lithornithidae (marked with a dashed line) is unknown. Silhouettes represent taxa and their habitats. All scale bars left to the IPF mappings is 200 µm. A red solid line marks K/Pg boundary; a dashed skyblue line denotes the initiation of cooling events in Miocene that might have caused gigantism of Palaeognathae (Crouch and Clarke, 2019). Silhouettes are attributable to (http://www.phylopic.org): Emily Willoughby (Citipati); Scott Hartman (Paraves), and Matt Martyniuk (Gigantoraptor). Other artworks are drawn by SC and NHK.
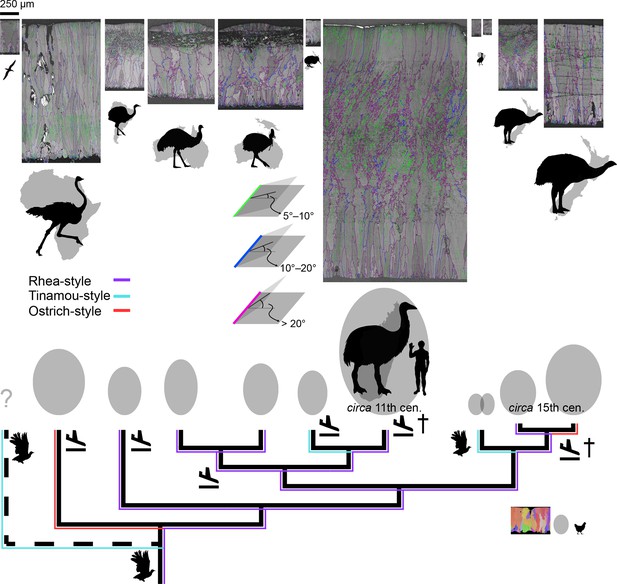
GB mappings, eggshell thickness, and egg size of Palaeognathae.
The green, blue, and purple lines in GB mapping denote the angle range between the calcite grains. All eggshell maps (including IPF mapping of chicken eggshell for comparison) are drawn to scale; note a scale bar at the upper left corner. The silhouettes of palaeognath are drawn to scale (note a human next to elephant bird and a chicken at the lower right corner). Egg shape and size are drawn to scale (Hauber et al., 2014; Stoddard et al., 2017). Two recently extinct lineages are marked by daggers and the extinct Lithorinithidae by a dashed branch. Landing symbols denote potential independent losses of flight (Mitchell et al., 2014; see also Sackton et al., 2019) and flying bird silhouettes denote volant taxa. Sky blue lines show microstructural and crystallographic similarities among tinamou-style eggshells that is attributable to homoplasy. Red lines mean the homoplastic similarities between ostrich-style eggshells. Purple lines represent potential homologies of rhea-style eggshells.
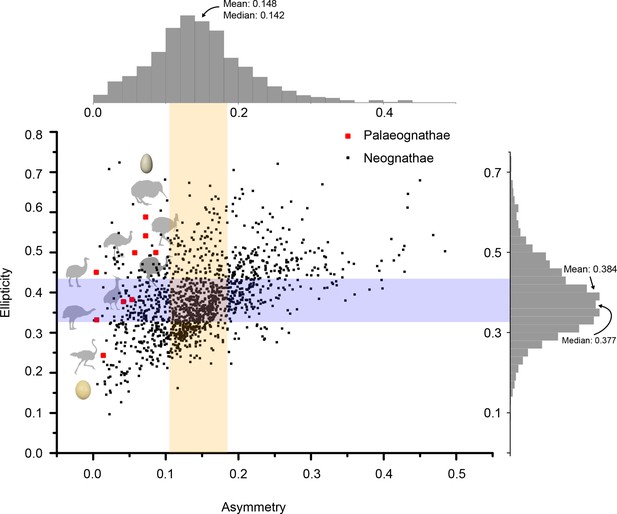
Ellipticity and asymmetry of palaeognath eggs.
Note that ellipticity of palaeognath eggs are not confined to certain range. Kiwi egg has maximum ellipticity and ostrich egg has minimum ellipticity. However, in case of asymmetry, palaeognath eggs have low asymmetry compared to neognath eggs. Coloured ranges mark interquartile ranges of both indices. Modified from Stoddard et al., 2017.
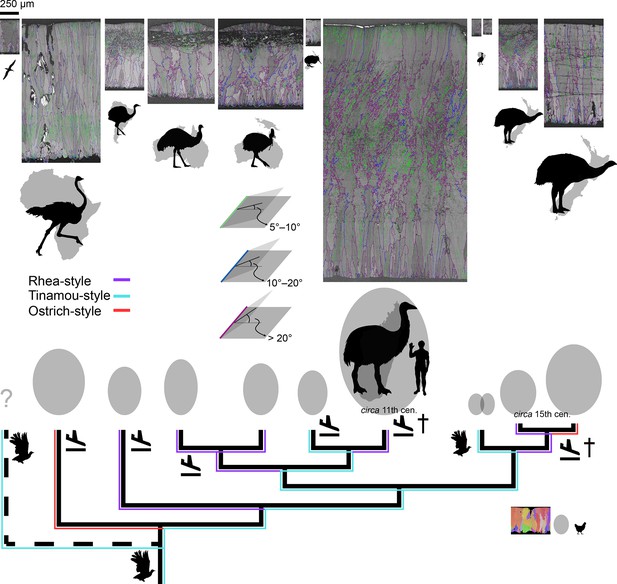
An alternative interpretation of evolution of palaeognath eggshells assuming that all tinamou-style eggshells are homologous to one another.
In this case, rhea-style eggshells might have been independently evolved at least four times.
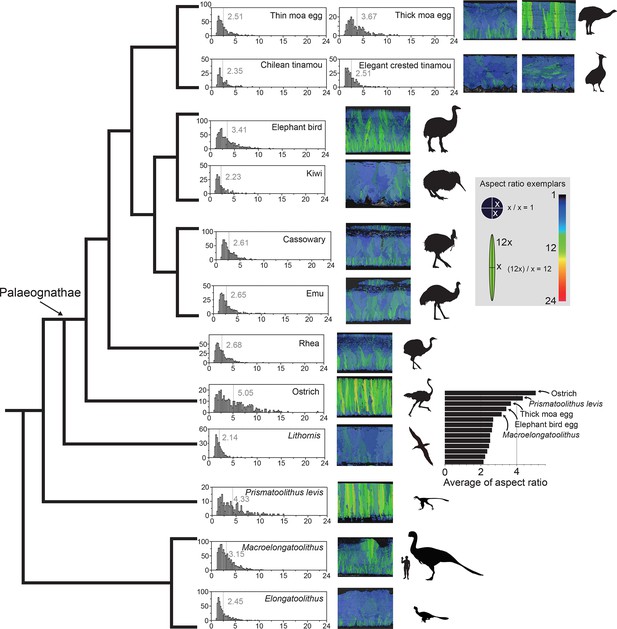
AR mappings and histograms of palaeognath and non-avian maniraptoran eggshells.
Note that ostrich, (Late Cretaceous) Prismatoolithus levis, giant moa (Dinornis), elephant bird, and (Late Cretaceous) Macroelongatoolithus eggshells are characterized by higher AR. The vertical bars and numbers in the histograms mean the average point of AR distribution and its value, respectively. Silhouettes of non-avian dinosaur are drawn to scale.
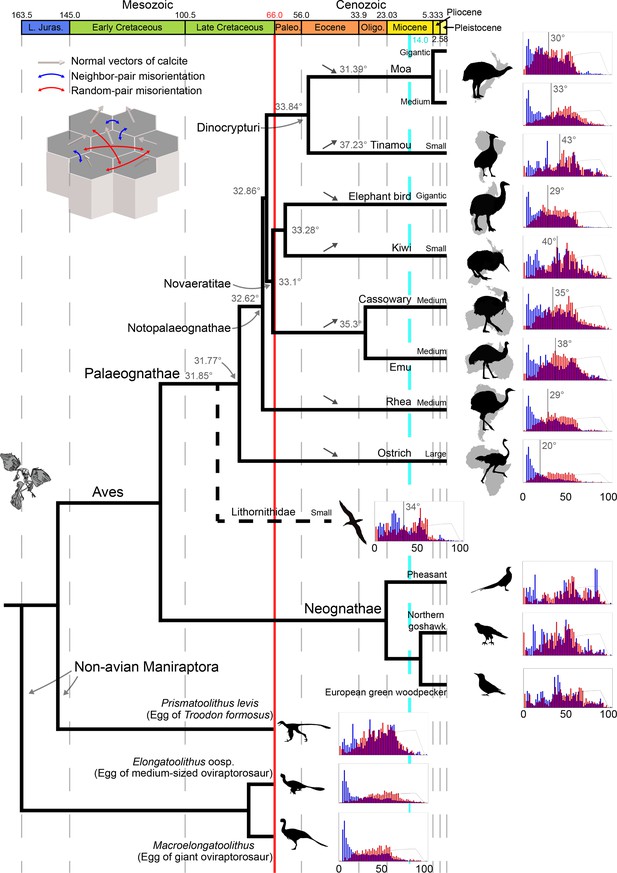
Misorientation distributions of palaeognath, neognath, and non-avian maniraptoran eggshells (adapted from Figure 13).
The vertical bars and numbers in the histograms mean the average point of neighbour-pair misorientation (blue) and its value, respectively. The numbers at the nodes represent the ancestral states for mean of neighbour-pair MD (note that only one MD of tinamou eggshell was shown for brevity). Up and down arrows mark the changing ancestral state trends of each node compared to the nearest ancestral states.
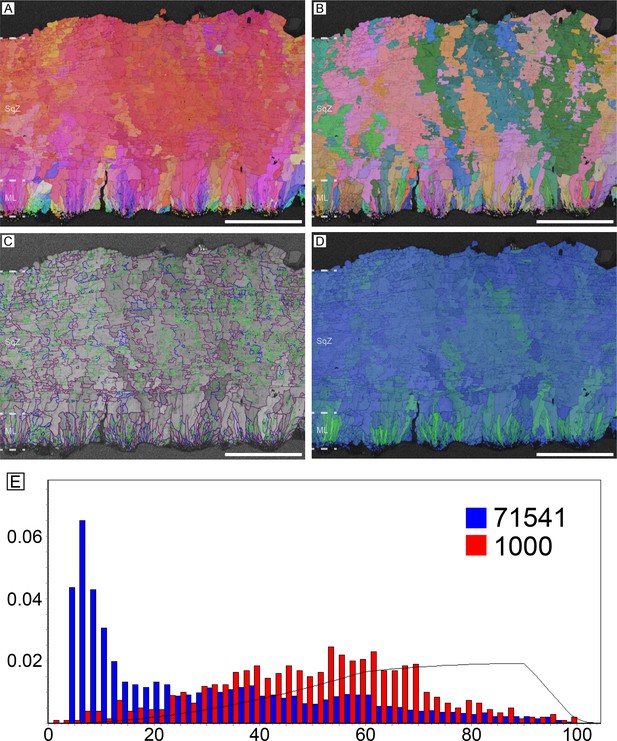
Elongatoolithus oosp.
(see also Choi et al., 2019). Scale bars equal 250 µm.
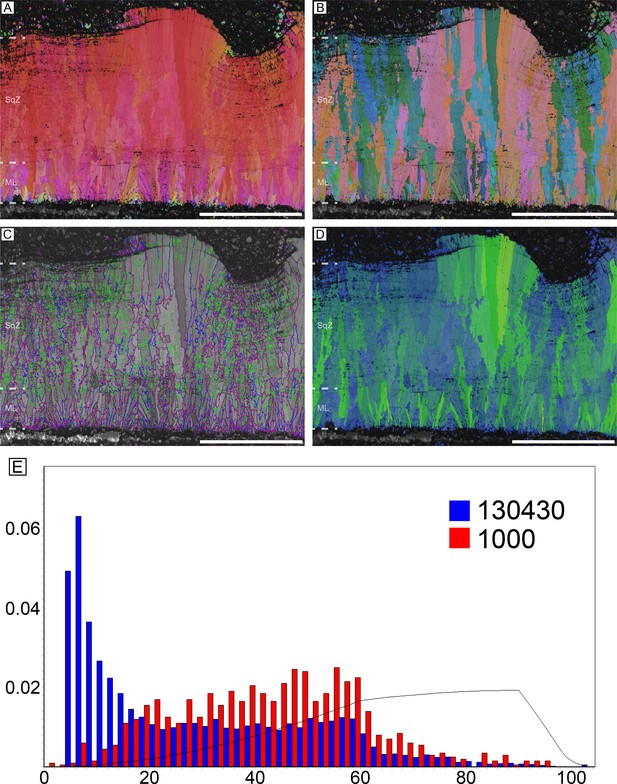
Macroelongatoolithus xixiaensis (or M. carlylei) (see also Huh et al., 2014; Choi et al., 2019).
Scale bars equal 1000 µm.
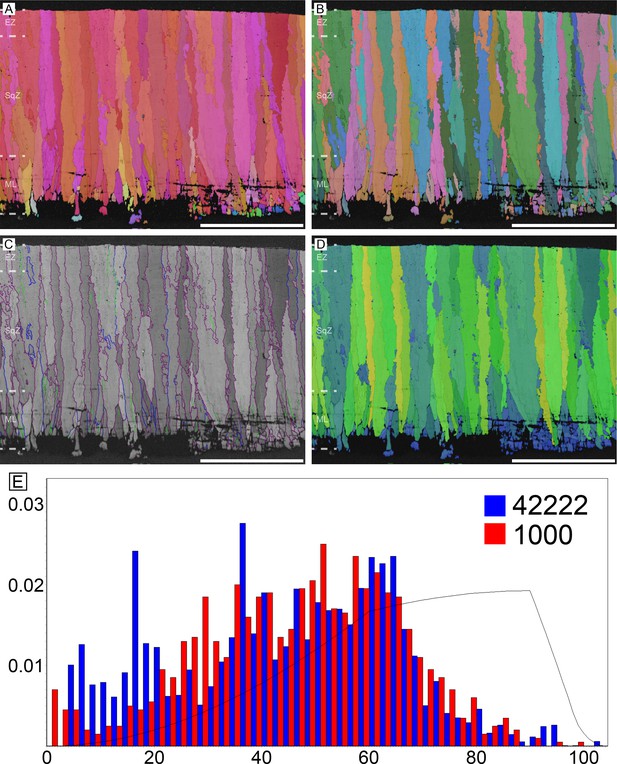
Prismatoolithus levis (see also Varricchio et al., 2002; Varricchio and Jackson, 2004).
Scale bars equal 500 µm.
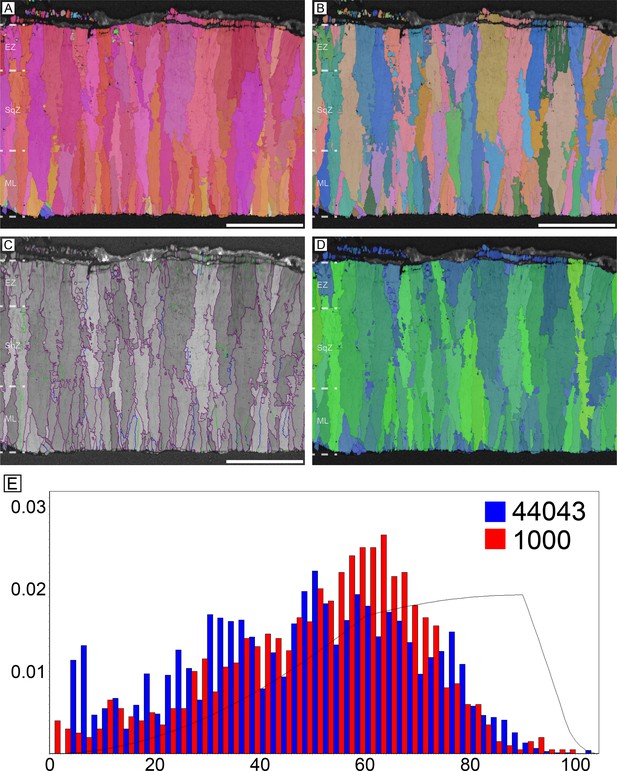
Triprismatoolithus stephensi (see also Jackson and Varricchio, 2010; Yang et al., 2018).
Scale bars equal 250 µm.
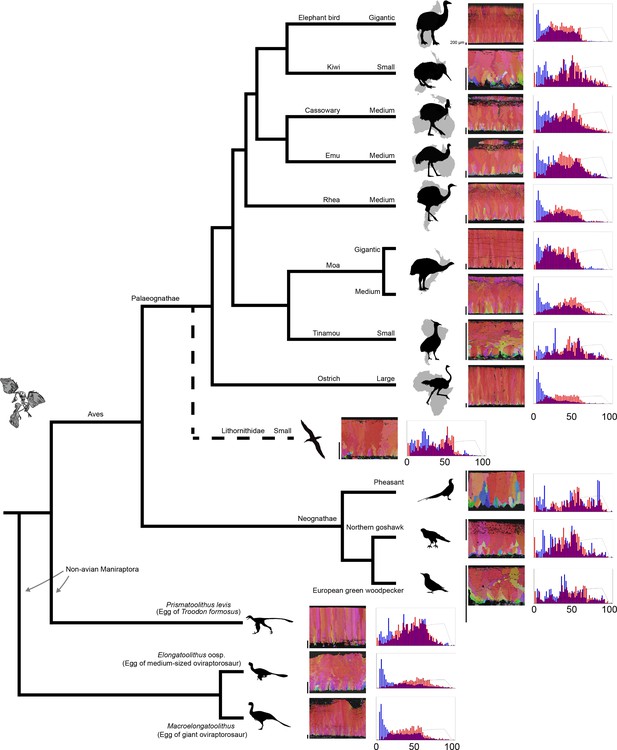
Alternative interpretation of phylogeny of Palaeognathae with IPF mapping and MD.
After Cloutier et al., 2019 and Sackton et al., 2019.
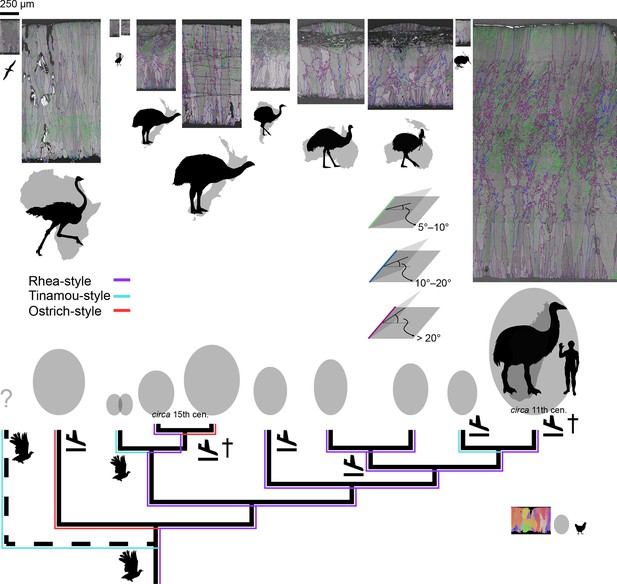
Alternative interpretation of phylogeny of Palaeognathae with GB mapping, egg size, and thickness of eggshells.
After Cloutier et al., 2019 and Sackton et al., 2019.
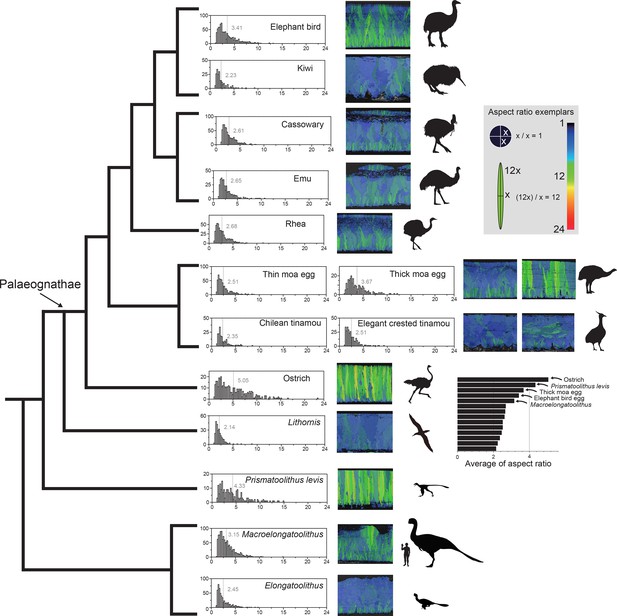
Alternative interpretation of phylogeny of Palaeognathae with AR mapping.
After Cloutier et al., 2019 and Sackton et al., 2019.