Sublytic gasdermin-D pores captured in atomistic molecular simulations
Figures
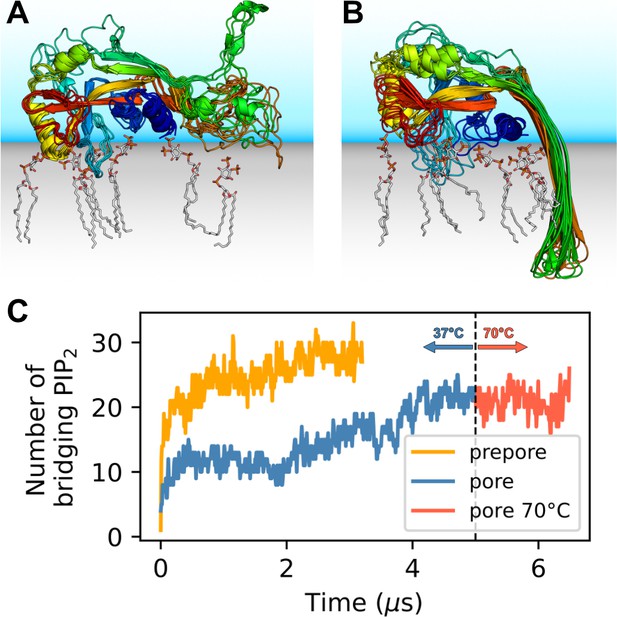
GSDMDNT interacts tightly with anionic lipids.
Overlay of six representative PI(4,5)P2 bound poses of the prepore monomer (A) and the 33-mer GSDMDNT ring in pore conformation (B). GSDMDNT is shown in cartoon representation and colored using a rainbow spectrum from blue (N-terminus) to red (C-terminus). The β1–β2 loop is colored in cyan, the α1 helix in dark blue, the α3 helix in yellow, and the C-terminus in red. PI(4,5)P2 is shown in grey licorice representation with orange phosphorus and red oxygen atoms. Hydrogen atoms are not shown for clarity. The membrane and solvent are schematically shown with gray and blue shades, respectively. (C) Number of PI(4,5)P2 molecules that interact simultaneously with two subunits of the prepore (orange) and pore (blue) 33-mer rings. After 5 µs at 37°C, the pore simulation was continued for 1.5 µs at 70 °C (red).
-
Figure 1—source data 1
Source data for Figure 1C.
- https://cdn.elifesciences.org/articles/81432/elife-81432-fig1-data1-v2.xlsx
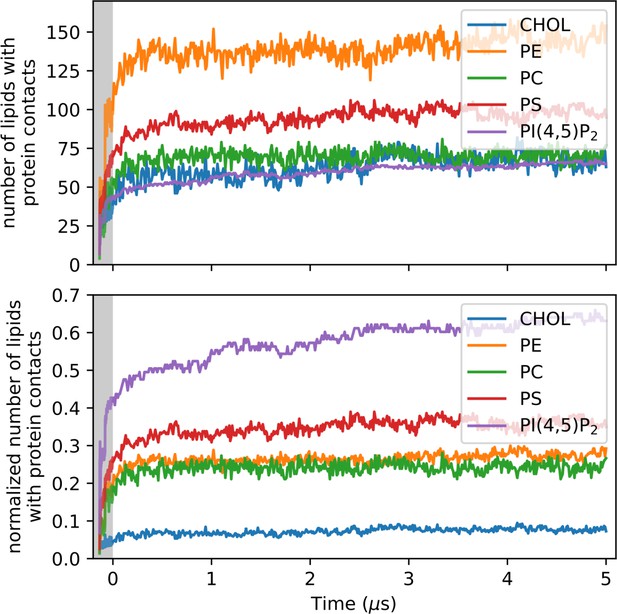
Change in the number of inner leaflet lipids, whose headgroups interact with at least one heavy atom of the 33-mer pore, in absolute counts (top) and normalized by the number of lipids of each lipid species in the inner leaflet (bottom).
Lipids are grouped by their headgroup identity. The gray-shaded area represents the equilibration phase of the MD simulations.
-
Figure 1—figure supplement 1—source data 1
Source data for Figure 1—figure supplement 1.
- https://cdn.elifesciences.org/articles/81432/elife-81432-fig1-figsupp1-data1-v2.xlsx
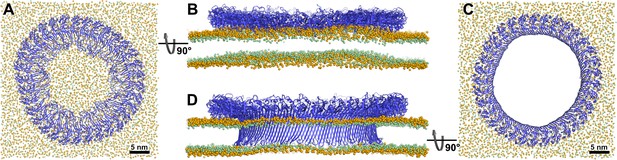
Atomistic MD simulations of GSDMDNT 33-mer rings in prepore and pore conformation.
Prepore (A,B; after 3.5 µs of MD) and pore rings (C,D; after 5 µs) are viewed from the top (A,C) and side (B,D). GSDMDNT is shown in blue cartoon representation, lipid headgroup phosphates and glycerol oxygens are shown as orange and green spheres, respectively. Water, ions, and lipid tails are not shown for clarity. The membrane under the prepore ring (A,B) is continuous but visibly bent upwards into the ring (B). In the pore conformation (C,D), lipids are absent from the central pore, which is lined by a continuous, membrane spanning β-barrel.
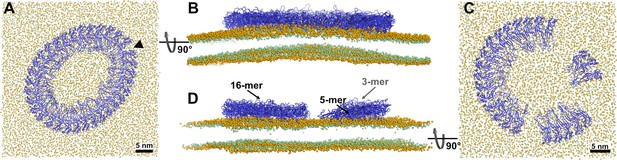
Snapshots of prepore 33-mer on a larger membrane patch and of prepore 3, 5, and 16-mer on one membrane patch.
Snapshots of 33-mer GSDMDNT prepore ring on 46×46 nm2 membrane after 2.2 µs of MD simulation, viewed from the top (A) and side (B). Snapshots of the system with 3, 5, and 16-mer GSDMDNT in prepore conformation after 1.5 µs of MD simulation, viewed from the top (C) and side (D). The GSDMDNT backbones are shown in blue cartoon representation. Lipid headgroup phosphates and glycerol oxygen atoms are shown as orange and green spheres, respectively. Water, ions, and lipid tails are not shown for clarity. (A) The black triangle indicates where the contacts of the globular domains of two neighboring subunits broke up.
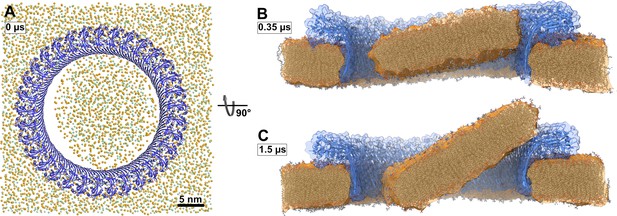
Atomistic MD simulations of GSDMDNT 33-mer rings in pore conformation and filled initially with lipids.
(A) Top view after equilibration. (B,C) Side views after 0.35 µs (B) and after 1.5 µs (C) of MD simulation shown as section through the pore center. The GSDMDNT backbones are shown in blue cartoon representation. In (A), lipid headgroup phosphates and glycerol oxygen atoms are shown as orange and green spheres, respectively. Water, ions, and lipid tails are not shown for clarity. In the sections (B,C), lipid tails are shown in full licorice representation. The outlines of the protein and membrane are represented as transparent surfaces.
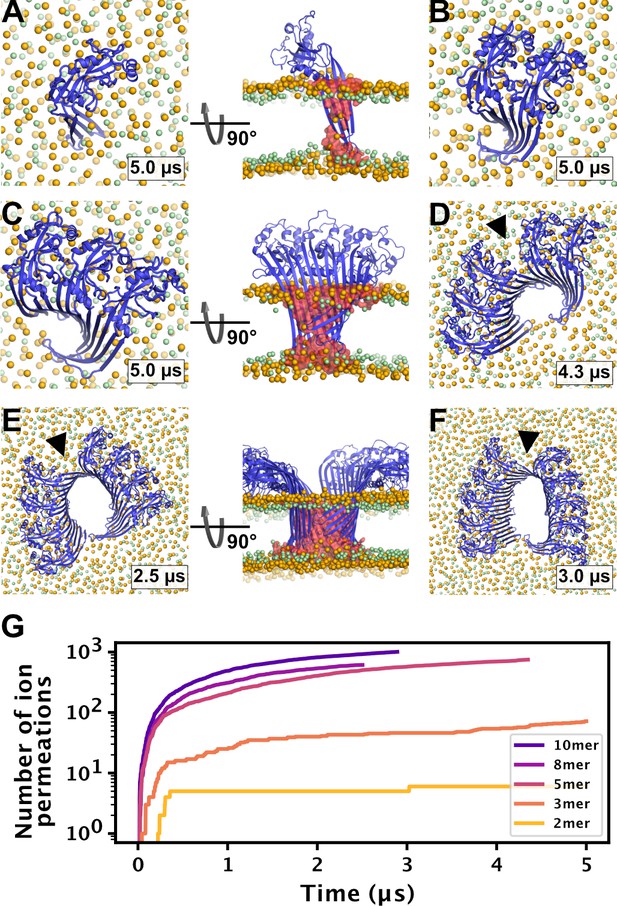
MD simulations of small GSDMDNT oligomers.
GSDMDNT monomer (A), dimer (B), trimer (C), pentamer (D), octamer (E) and decamer (F) remain membrane inserted for the full duration of the respective MD simulations. The β-sheets of 2, 3, 5, 8, and 10-mers coil up into small membrane pores filled with water (water inside the pore shown as red volume in the right panels of A,C,E). The GSDMDNT backbones are shown in blue cartoon representation. Lipid headgroup phosphates and glycerol oxygens are shown as orange and green spheres, respectively. Water, ions, and lipid tails are not shown for clarity except in the right panels of A,C,E. Black triangles indicate sites where the arc had cracked. (G) Cumulative sodium and chloride ion permeation events during the simulations. No ions permeated the membrane in the monomer simulation.
-
Figure 4—source data 1
Source data for Figure 4G.
- https://cdn.elifesciences.org/articles/81432/elife-81432-fig4-data1-v2.xlsx
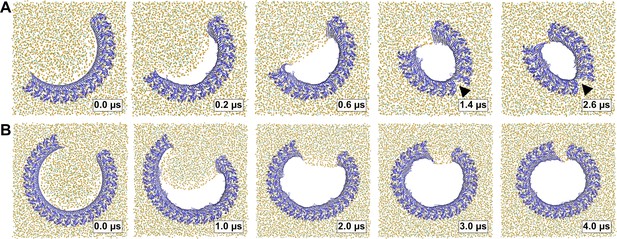
Arc-shaped GSDMDNT oligomers transition into slit or ring-shaped membrane pores.
Top views of GSDMDNT arcs comprising 16 (A) and 27 (B) subunits in pore conformation along MD simulation trajectories (time points indicated) show phospholipid headgroups (orange spheres) and cholesterol oxygens (green spheres) receding from the inserted β-sheet, before the open protein edges approach each other and close into slit-shaped (A) or ring-shaped (B) pores. Water, ions, and lipid tails are not shown for clarity.
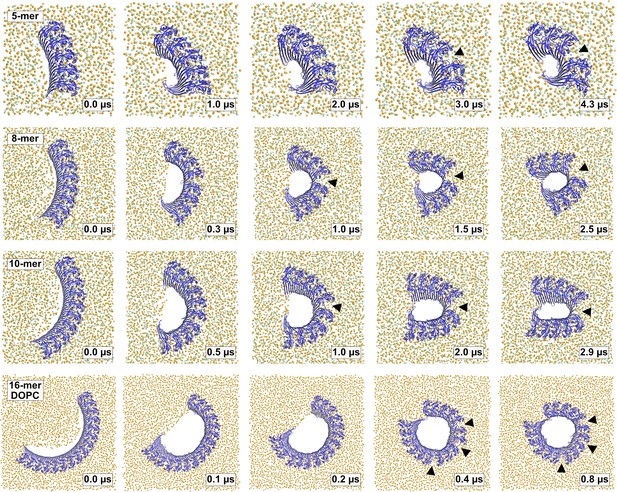
Time lapse images of pore formation from 5–10-meric arcs in the plasma membrane and a 16-meric arc in a pure DOPC membrane.
Top view snapshots of GSDMDNT arcs comprising 5–16 subunits in pore conformation along MD simulation trajectories show lipids (orange spheres) and cholesterol (green spheres) receding from the inserted β-sheet, before the open protein edges come closer to each other. In the case of the 16-mer in a fluid DOPC membrane, the arc closes and forms a ring shaped pore. In all cases, the interfaces between globular domains broke in one to three positions, as indicated with black triangles. Water, ions, and lipid tails are not shown for clarity.
Formation of a slit-like pore from a membrane inserted 16-meric arc.
Trajectory from 2.0 µs of simulation, showing the plasma membrane edge receding from the protein and then shortening. This draws the ends of the multimer together, causes breakage of the contacts of two neighboring globular domains, and ultimately the slit-shaped pore of Figure 5A (2.6 µs) develops. Lipid headgroup phosphates are shown as yellow spheres, cholesterol oxygen atoms as light green spheres, and the protein is shown in blue cartoon representation. Water and ions are not shown for clarity.
Formation of a ring-like pore from a membrane inserted 27-meric arc.
Trajectory from 4.0 µs of simulation, showing the plasma membrane edge receding from the protein. Lipid efflux first increases the distance between the arc ends, before the open membrane edge then draws the ends together to form the ring-shaped pore of Figure 5B (4.0 µs). Lipid headgroup phosphates are shown as yellow spheres, cholesterol oxygen atoms as light green spheres, and the protein is shown in blue cartoon representation. Water and ions are not shown for clarity.
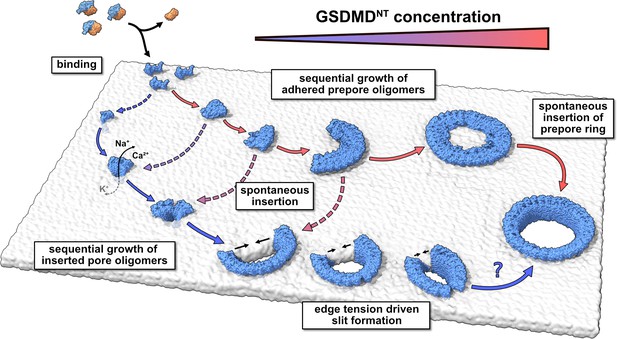
Model of membrane pore formation by GSDMDNT.
After proteolytic cleavage, GSDMDNT monomers bind the inner leaflet of the plasma membrane. Aided by specific lipid interactions they multimerize and, at a critical size, spontaneously insert into the membrane. Depending on the concentration of membrane adhered GSDMDNT, the insertion may proceed either from a fully formed prepore ring or from small oligomeric assemblies. Dotted arrows indicate that the mechanism of β-sheet insertion so far remains unresolved. Pores formed by small oligomers cause early nonspecific ion flux and can combine with one another or grow sequentially by the attachment of uninserted monomers. Depending on the edge tension in the cellular milieu, arcs would continue to grow or crack to form slit-shaped pores (bottom). Whether slit-shaped pores can grow to circular pores by subsequent mono- or oligomer attachment is unclear.
Tables
Summary of arc cracking events.
System size | Cracked subunit interfaces | Time of cracking [μs] |
---|---|---|
5 | 2,3 | 2.91 |
8 | 4,5 | 0.23 |
10 | 5,6 | 0.74 |
16 | 8,9 | 0.89 |
*16 | 6,7; 9,10; 11,12 | 0.4; 0.26; 0.27 |
-
*
Simulated in pure DOPC membrane.
Eisenberg hydrophobicity scores (Eisenberg et al., 1982) of the pore facing residues of human GSDMD and mouse GSDMA3 in kcal mol-1.
Structural element | human GSDMD | mouse GSDMA3 | ||
---|---|---|---|---|
Pore facing residues | ΣEisenberg hydrophobicity | Pore facing residues | ΣEisenberg hydrophobicity | |
β3 | ADQQSE | –2.73 | MDQQLE | –1.93 |
β5 | KAGASS | –0.96 | TKKTGS | –2.66 |
β7 | TKESRS | –4.18 | TNNISP | –1.06 |
β8 | QEQHSK | –3.76 | LGQSNN | –1.54 |
Σfull sheet | –11.63 | –7.19 |
Asymmetric plasma membrane composition.
Fatty acid tails abbreviated as FA.
Lipid | FA | Full name | Charge | Inner leaflet [mol %] | Outer leaflet [mol %] |
---|---|---|---|---|---|
CHOL | Cholesterol | 0 | 40.4 | 40.0 | |
PSM | 18:1/16:0 | N-palmitoyl-D-erythro-sphingosylphosphorylcholine | 0 | 1.0 | 12.0 |
NSM | 18:1/24:1 | N-nervonoyl-D-oleoyl-sphingosylphosphorylcholine | 0 | - | 9.0 |
LSM | 18:1/24:0 | N-lignoceroyl-D-oleoyl-sphingosylphosphorylcholine | 0 | - | 8.0 |
PLPC | 16:0/18:2 | 1-palmitoyl-2-linoleoyl-sn-glycero-3-phosphocholine | 0 | 8.1 | 15.0 |
SOPC | 18:0/18:1 | 1-stearoyl-2-oleoylphosphocholine | 0 | - | 7.0 |
PAPC | 16:0/20:4 | 1-palmitoyl-2-arachidonoyl-glycero-3-phosphocholine | 0 | - | 5.0 |
POPC | 16:0/18:1 | 1-palmitoyl-2-oleoyl-glycero-3-phosphocholine | 0 | 3.0 | - |
DPPC | 16:0/16:0 | 1,2-dipalmitoyl-glycero-3-phosphocholine | 0 | 2.0 | - |
PLA20(PE) | 18:0/20:4 | 1-O-stearoyl-2-O-arachidonoyl-glycero-3-phosphoethanolamine | 0 | 11.1 | 3.0 |
PDoPE | 16:0/22:6 | 1-palmitoyl-2-docosahexaenoyl-glycero-3-phosphoethanolamine | 0 | 8.1 | - |
SAPE | 18:0/20:4 | 1-stearoyl-2-arachidonoyl-glycero-3-phosphoethanolamine | 0 | 4.0 | - |
POPE | 16:0/18:1 | 1-palmitoyl-2-oleoyl-glycero-3-phosphoethanolamine | 0 | 3.0 | - |
PAPS | 16:0/20:4 | 1-palmitoyl-2-arachidonoyl-glycero-3-phosphoserine | -1 | 13.1 | - |
SAPS | 18:0/20:4 | 1-stearoyl-2-arachidonoyl-glycero-3-phosphoserine | -1 | 1.0 | 1.0 |
PI(4,5)P2 | 16:0/18:2 | 1-palmitoyl-2-linoleoyl-sn-glycero-3-phosphoinositol-4,5-bisphosphate | -4 | 5.1 | - |
Restraints used during energy minimization (EM) and stepwise equilibration (steps 1–6) of the asymmetric plasma membrane mimetic in kJ mol-1nm-2.
Step | Time [ns] | Timestep [fs] | Ensemble | Lipids | Dihedrals |
---|---|---|---|---|---|
EM | 1000 | 1000 | |||
1 | 0.125 | 1 | NVT | 1000 | 1000 |
2 | 0.125 | 1 | NVT | 400 | 400 |
3 | 0.125 | 1 | NPT | 400 | 200 |
4 | 0.5 | 2 | NPT | 200 | 200 |
5 | 0.5 | 2 | NPT | 40 | 100 |
6 | 0.5 | 2 | NPT | 0 | 0 |
Restraints used during energy minimization (EM) and stepwise equilibration (1–3) of GSDMDNT pore conformation systems in kJ mol-1nm-2.
Step | Time [ns] | Timestep [fs] | Backbone | Sidechains | Lipid headgroup () | Water () |
---|---|---|---|---|---|---|
EM | 4000 | 2000 | 1000 | 1000 | ||
1 | 5* | 2 | 2000 | 1000 | 10 | 50* |
2 | 50 | 2 | 2000 | 1000 | 50 | 0 |
3 | 80 | 2 | 500 | 100 | 0 | 0 |
-
*
For the 27-mer system with an initially lipid filled pore this was extended to 10 ns with a force constant of 1000 kJ mol−1 nm−2 for restraining the z-position of water molecules.
Atomistic GSDMDNT simulations.
Conformation | Subunits | Simulated time [µs] | Membrane size [nm2] | No. of atoms |
---|---|---|---|---|
prepore | 1 | 7.0 | 13.4×13.4 | 222740 |
prepore | 33 | 3.5 | 38.9×38.9 | 1950552 |
*prepore | 3, 5, 16 | 1.5 | 38.9×38.9 | 1916829 |
prepore | 33 | 2.2 | 45.8×45.8 | 3113594 |
pore | 1 | 5.0 | 12.7×12.7 | 216375 |
pore | 2 | 5.0 | 12.5×12.5 | 213665 |
pore | 3 | 5.0 | 12.2×12.2 | 208656 |
pore | 5 | 4.3 | 18.8×18.8 | 481242 |
pore | 8 | 2.5 | 25.5×31.9 | 1079514 |
pore | 10 | 3.0 | 25.4×31.7 | 1071088 |
pore | 16 | 3.5 | 31.3×31.3 | 1329398 |
†pore | 16 | 1.4 | 34.9×34.9 | 1497819 |
pore | 27 | 4.1 | 37.2×37.2 | 2226909 |
pore | 33 | 5.0 | 37.0×37.0 | 2119785 |
‡pore | 33 | 1.5 | 37.0×37.0 | 2119785 |
§pore | 33 | 1.5 | 37.0×37.0 | 1885031 |
-
*
simulated cut out oligomers of the full 33-mer prepore ring after 200 ns.
-
†
simulated in pure DOPC membrane.
-
‡
70°C continuation of the 37°C simulation after 5 µs.
-
§
simulated with lipid plug inside pore.