Novel pathogen introduction triggers rapid evolution in animal social movement strategies
Figures
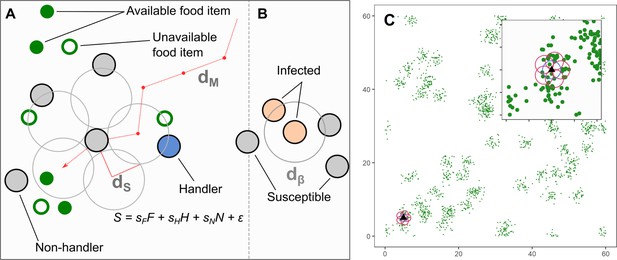
Model implementation of discrete movement steps on a landscape with continuous space, with movement steps selected based on inherited preferences for environmental cues.
(A) Individuals search for clusters of food items (green circles), which may be immediately available (filled green circles; ), or may be available only in the future (open green circles). Individuals can sense only available items, and not unavailable ones. Individuals can sense other foraging individuals, and whether they have successfully found and are handling a food item (handlers; blue circles; ), or whether they are unsuccessful foragers still searching for food (non-handlers; filled grey circles; ). To decide where to move, individuals sample their environment for these cues at five locations around themselves (large open grey circles) and have a sensory range of (default = 1.0 units). Individuals assign each potential direction a suitability, , where the coefficients are inherited preferences for environmental cues, and is a small error term that helps break ties between locations. The sensory distance () and the movement distance () are the same, 1.0 units. (B) An infectious pathogen is transmitted between infected (orange circles) and susceptible (filled grey circles) individuals, with a probability = 0.05, when they are within a distance of each other. In our implementation, is the same as = 1.0 units. (C) An example of the resource landscape used in our simulations, consisting of 60 randomly distributed clusters of food items, with 1800 discrete food items divided among the clusters (30 items per cluster). The landscape is a square of 60 units per side, with wrapped boundaries (i.e. a torus). The food item density is 0.5 food items per unit area. Items are distributed around the centre of each cluster, within a standard deviation of 1.0 unit. Items, once consumed by foragers, are unavailable for a fixed number of timesteps (the regeneration time , expressed in terms of the foragers generation time), after which they regenerate in the same location. While regenerating (i.e. unavailable), items cannot be perceived by foragers. The sensory ranges of individuals () are shown for each potential step (red circles, including the current location: blue circle). Food item clustering means that available items, as well as foragers handling a food item (handlers), are good indicators of the location of a resource cluster.
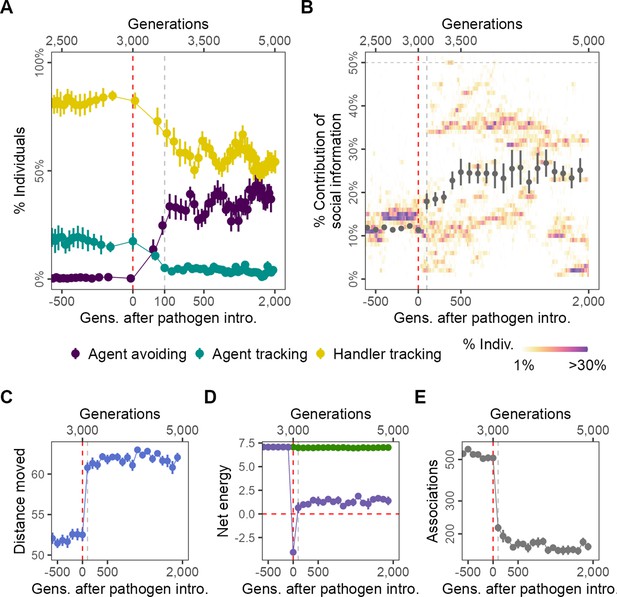
Pathogen introduction leads to rapid evolutionary changes in social information use, with cascading effects on population-level behaviour.
(A) Before pathogen introduction in the default scenario (R = 2, = 0.25), populations rapidly evolve to mostly track handlers and avoid non-handlers (handler-tracking; ); however, the preference for food items () is the major determinant of their fine-scale movement decisions. Pathogen introduction leads to a rapid increase in agent avoidance which stably coexists with the handler-tracking strategy in an eco-evolutionary equilibrium. (B) After pathogen introduction (), the importance of social cues (the presence of other individuals; the sum of the absolute, normalised preferences ) doubles on average (grey points; from 10% to >20%). Additionally, there is significant variation in the importance of social cues to individuals (shaded regions), which is not captured by the mean or standard error. The rapid change in social movement strategies following pathogen introduction has cascading effects on population-level behaviour. Individuals, which have evolved aversions to some kinds of foragers (depending on their strategy), (C) move 15% more on average, (D) have substantially reduced per-capita energy (purple) due to the cost of infection, as mean per-capita intake remains unchanged (green), and (E) also have fivefold fewer associations with other foragers. All panels show data averaged over 10 replicates, with mean and standard error; shaded regions in panel (B) are from a single replicate for clarity. Panels X axes begin at G = 2500,, and panel (A) X-axis is transformed to show the generations after introduction more clearly.
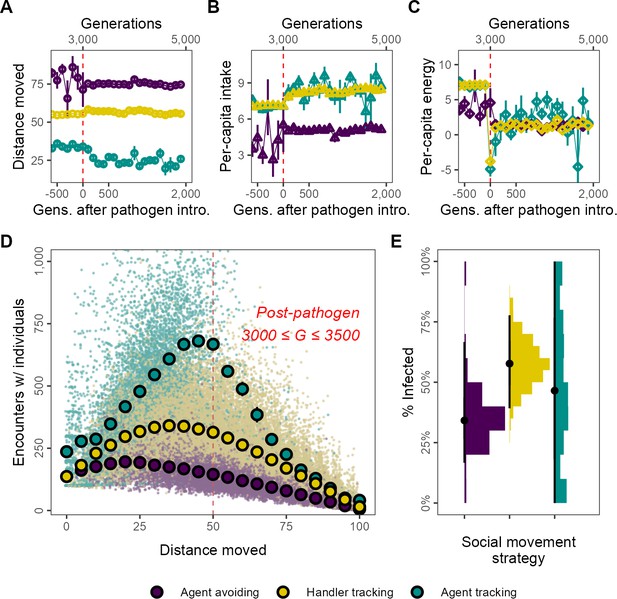
Social movement strategies coexist by trading movement for associations through dynamic social distancing, leading to differences in intake and infection rates.
Population-level outcomes mask substantial variation in strategy-specific behaviour and outcomes. The three main movement strategies differ in the mean distance moved, with the agent-avoiding strategy moving substantially more (A), and having less intake (B) than the other strategies. Nonetheless, all three strategies have similar net energy and hence equivalent fitness (C). In post-introduction populations (3000 ≤ G ≤ 3500), (D) the distance moved by individuals of the three main strategies has a non-linear relationship with the number of associations. Individuals that move either very little (<15) or constantly (>75) have few associations. However, individuals that move intermediate distances have more associations. This curve is influenced by the social movement strategy, with agent-tracking individuals having more associations than the handler-tracking strategy for the same distance moved, while handler-tracking individuals have similarly more associations than agent-avoiding individuals. (E) Avoiding all other foragers leads to lower infection rates than tracking successful foragers (and avoiding unsuccessful ones; handler-tracking). Surprisingly, rare pre-introduction strategies such as following any nearby individuals (agent-tracking) may also have low infection rates, potentially due to their rarity. Panel (D) shows the mean and standard error for movement distance bins of five units (note standard error is very small in some cases); panel (B) shows infection rates; all data represent generation- and replicate-specific means (R = 2, = 0.25).
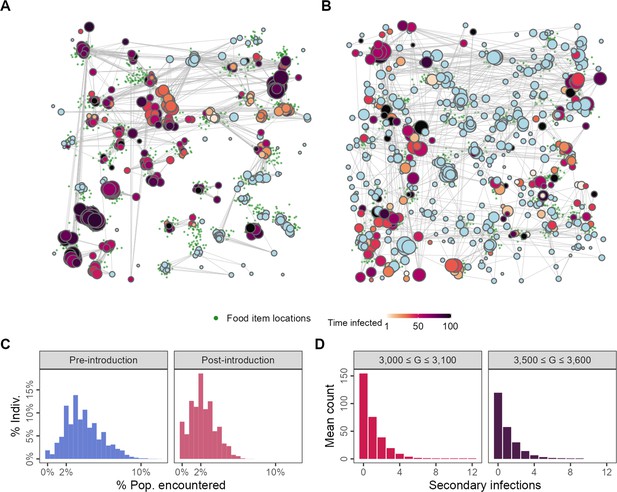
Changes to spatial-social structure in populations adapted to the presence of an infectious pathogen.
Pathogen-risk-naive populations (A; G = 3000) are clustered into modules by the end of their lives, while pathogen-risk-adapted populations (B; G = 3500) are more widely dispersed over the landscape. Pre-introduction individuals encounter somewhat more unique neighbours (C, blue) than pathogen-risk-adapted individuals (C; red). (D) The distribution of the individual reproductive number is left-skewed, with most infections not resulting in any secondary cases, but has a long right-hand tail, suggesting that a small number of infected individuals are responsible for a large number of infections, suggesting that superspreading emerges from the spatial-social dynamics encoded in the model. Panels (A) and (B) show social networks from a single replicate of the default implementation of scenario 1 (R = 2, = 0.25), while all other panels show the average of 10 replicates. Nodes represent individuals positioned at their final location in (A) and (B). Connections represent pairwise encounters (connections with weights <33rd percentile are removed for ease of visualisation), and node size represents individuals’ social associations (larger = more associations). Darker node colours indicate longer infection (light blue = no infection).
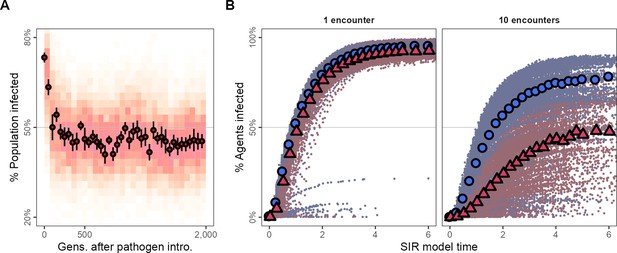
Adapting to moving under the risk of pathogen transmission makes populations more resilient to the spread of some kinds of infections.
(A) In the first generations following pathogen introduction, about 75% the population is infected. However, within 100 generations, tracking the evolutionary shift towards movement strategies that avoid all other individuals, only about 50% of individuals are infected overall. (B) The progression of two hypothetical infections, requiring a single encounter, or 10 encounters for a potential transmission, on the emergent social networks of pre- and post-introduction populations. The transmission of the multiple-encounter infection is reduced in populations with disease-adapted movement strategies (pre-introduction: G = 3,000,, blue circles; post-introduction: G = 35000, red triangles). Subfigures in panel (B) show means of 25 SIR model replicates (transmission rate = 5.0, recovery rate = 1.0), run on emergent social network; both panels represent 10 simulation replicates of the default implementation of scenario 1 ( = 2, = 0.25).
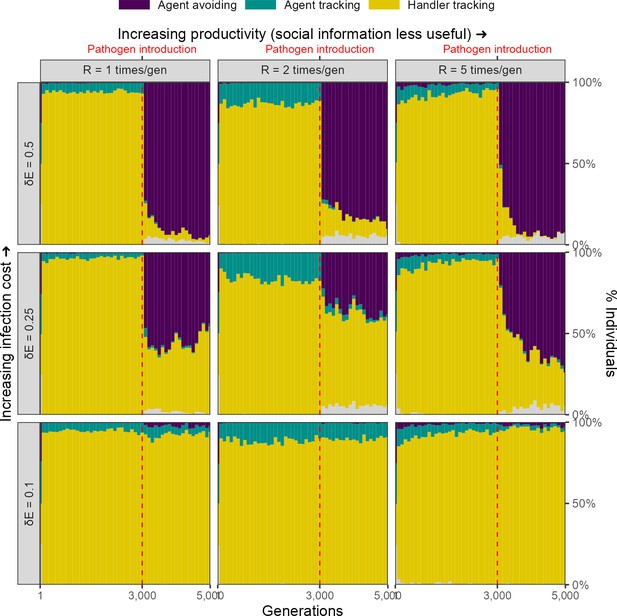
Infection cost, but not the usefulness of social information, shapes the rapid evolutionary change in movement strategies triggered by pathogen introduction.
Pre-introduction (G = 3000; dashed line) populations mostly contain individuals that track successful foragers (handler-tracking), with a small number of individuals that track all foragers (agent-tracking). After pathogen introduction, indiscriminate agent avoidance becomes a common strategy, but only when landscape productivity cannot compensate for infection costs ( 0.25, 0.5). In cases where the infection cost is low, handler-tracking persists as the most common strategy after pathogen introduction. All panels show frequencies over 10 replicate simulations in 100-generation bins; frequencies are stacked. Grey areas show the relatively uncommon non-handler-tracking strategy that sometimes arises due to mutations.
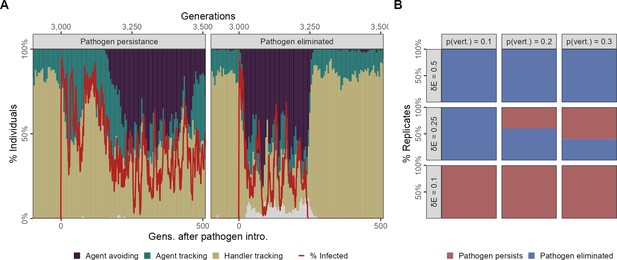
Feedback between evolutionary transitions in social movement strategies and pathogen persistence after a single introduction event with vertical transmission.
In scenario 2 with only a single introduction event (initial infections = 20) but also vertical transmission from parents to offspring at the reproduction stage, simulation replicates show divergent outcomes. (A) In some replicates, the population is slow to transition away from sociality, and the agent-avoiding strategy becomes common only after 200 generations. In such cases, the pathogen persists among social individuals for over 500 generations (panel Pathogen persistence). In contrast, when the population undergoes a rapid evolutionary shift and agent avoidance becomes common within 100 generations, the number of infections falls rapidly. This sets up a feedback between social strategies and the number of infections, with infections tracking the frequency of the more social strategies with a time lag of a few generations (panel Pathogen eliminated). In some cases, infections drop to zero, which drives the pathogen extinct following which there is an extremely rapid recovery in the frequency of the more social handler-tracking strategy, and the near-complete extinction of agent-avoiding foragers. (B) Infection cost and the probability of vertical transmission together influence whether populations undergo evolutionary transitions that lead to pathogen elimination. In general, pathogen elimination is more common when pathogen costs are higher (as infected individuals have fewer offspring), and when the probability of vertical transmission is low. When infection costs are low ( = 0.1), there is no evolutionary transition, and the pathogen persists in the population even when transmission between generations is low (pv = 0.1). At intermediate infection costs ( = 0.25), pathogen persistence increases with the probability of vertical transmission. All panels show the outcomes of 10 replicates with the default landscape spatial structure, and with a landscape productivity = 2. Pathogen persistence or elimination is measured at G = 3500, that is,, 500 generations after the first introduction.
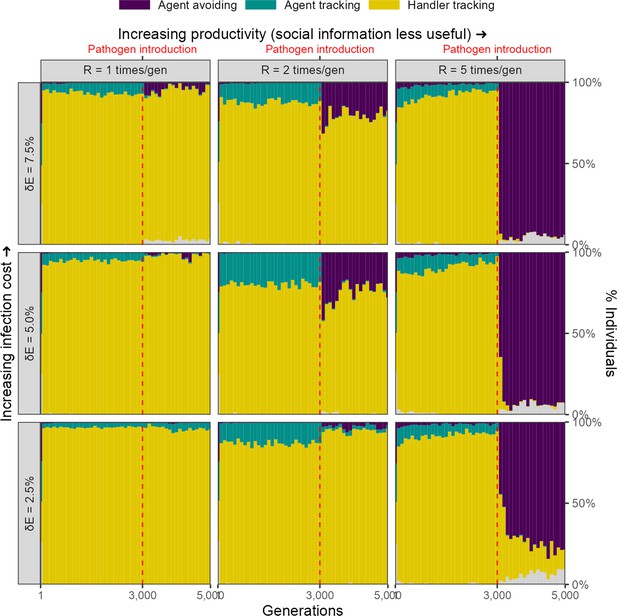
Rapid evolutionary change under some conditions in an alternative implementation of disease costs.
In our alternative, percentage costs implementation of the infectious pathogen, there is a rapid shift in the mix of movement strategies after pathogen introduction, but only when the costs of infection are relatively high (7.5%), and the usefulness of social information is limited by the abundance of food items (R = 5). In these cases, the agent-avoiding strategy is the most common social movement strategy, forming a smaller proportion of the population mixture of social movement strategies when the infection cost is lower, or when the usefulness of social information is greater (lower and lower , respectively).
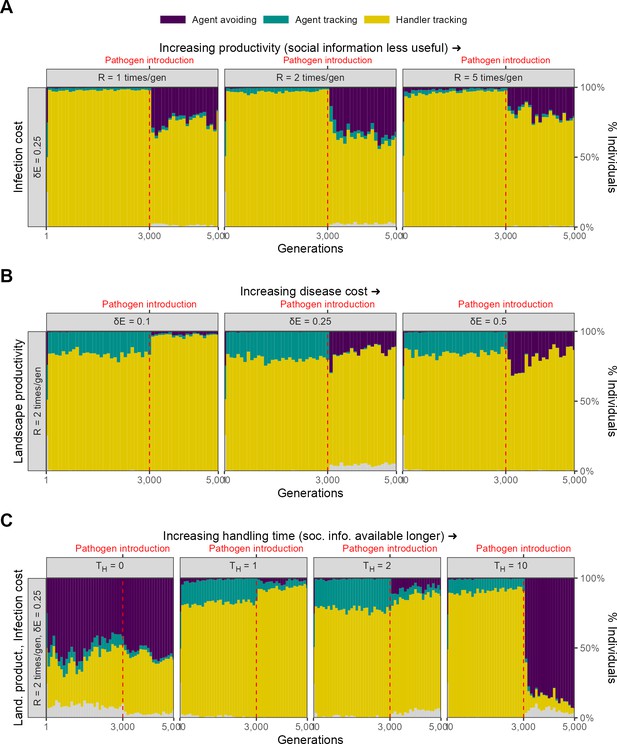
Evolutionary outcomes show the effect of modelling choices in alternative implementations of scenario 1.
(A) Large-scale (or global) natal dispersal leads to evolutionary outcomes similar to the default implementation of small-scale or local natal dispersal ( 1, 2, 5; = 0.25). (B) A threshold on reproduction such that only individuals with a net positive energy balance (lifetime intake > total infection cost) are allowed to reproduce leads to the persistence of the handler-tracking strategy. This is likely because the intake-infection risk trade-off of complete agent avoidance leads to an indirect avoidance of food items, and hence intake; in turn this likely prevents agent-avoiding individuals from reproducing. (C) The availability and indirect costs of using social cues jointly determine how the persistence of inadvertent social information affects the evolution of social movement strategies. When the indirect costs of social information are low ( 1, 2), handler-tracking persists beyond pathogen introduction. When these costs increase, individuals eschew social associations and are agent-avoiding ( = 10). When there is no social information on food items available ( = 0), all individuals are functionally agent-avoiding (as there are no handlers).
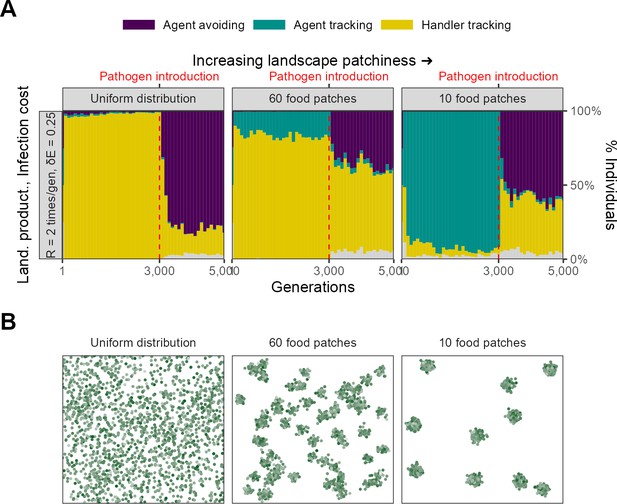
Landscape spatial structure influences the evolution of social movement strategies before, but not after, pathogen introduction.
(A) In two implementations with different spatial structures (=2, = 0.25), pre-pathogen dynamics are actually more different than post-introduction ones. On landscapes with a uniform food distribution (left panel: Uniform distribution), all individuals before pathogen introduction were handler-tracking. On more clustered landscapes (right panel: 10 food patches; default = 60, centre panel), the rare agent-tracking strategy is most common before pathogen introduction. This is likely because the time cost of moving between distant patches on clustered landscapes is higher than that of exploitation competition. After pathogen introduction, agent avoidance rapidly becomes a common strategy. It is more dominant on uniform landscapes (~80%) likely because the usefulness of social information is lower there. (B) Panels show representative landscapes corresponding to the outcomes in (A).
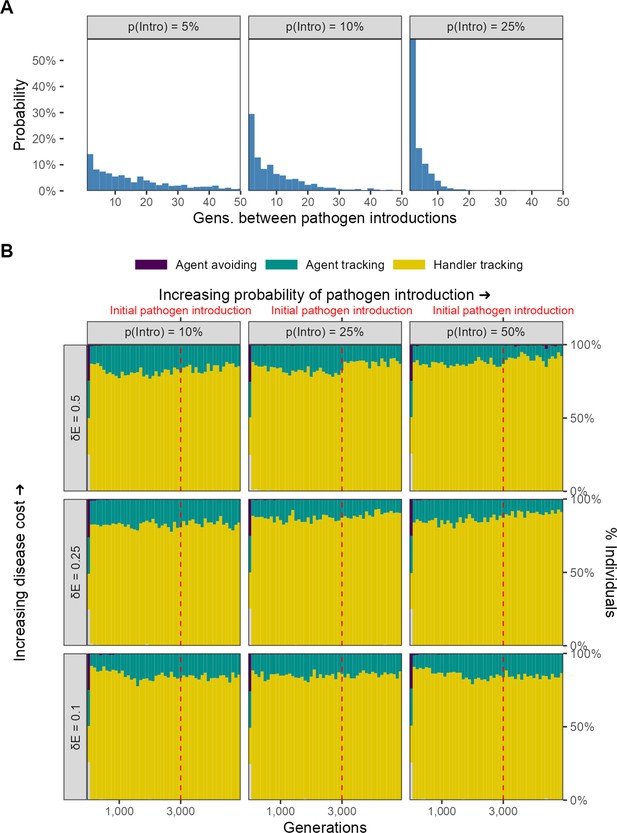
No evolutionary change in social movement strategies when novel pathogen introduction events are relatively uncommon.
(A) In our alternative implementation of scenario 1, the pathogen is only introduced sporadically after the initial introduction (G = 3000; red line in panel B). (B) When introductions are relatively rare and sporadic, there is no shift in the mixture of movement strategies after pathogen introduction. The handler-tracking strategy remains common across parameter combinations. Panels represent combinations of the per-timestep cost of infection and the spillover rate (rows), which is the probability of pathogen introduction in each generation (columns). All panels show the combined outcomes of 10 replicate simulations.