Targeting oncogenic KRasG13C with nucleotide-based covalent inhibitors
Figures
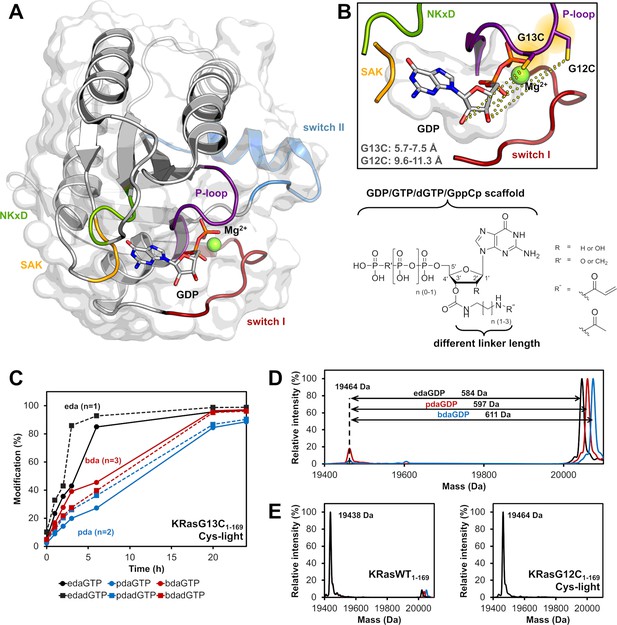
Rational design of nucleotide-based covalent KRasG13C inhibitors.
(A) Structure of KRasWT in the GDP-bound state (grey, P-loop: violet, switch I: red, switch II: blue, NKxD: green, SAK: yellow; PDB 4obe). (B) Model of KRasG13C (model is based on PDB 4obe) showing the distances between the cysteine residue and the OH-groups of the ribose and the general structure of nucleotide derivatives bearing an electrophilic group at the 2’,3’-position of the ribose moiety, showing that position 12 is further remote compared to position 13 and thus explaining the observed specificity of covalent bond formation. (C) Time-dependent analysis of the covalent modification of KRasG13C1-169 (Cys-light) at pH 9.5 using different linker lengths (eda: ethylenediamine, pda: propylenediamine and bda: butylenediamine). (D) Covalent modification of KRasG13C1-169 (Cys-light) proteins at pH 9.5 after 24 hr at room temperature. (E) In contrast, KRasG12C1-169 (Cys-light) mutant was not modified by nucleotide derivatives and for KRasWT1-169 very little unspecific labelling at pH 9.5 was observed compared to the G13C mutant.
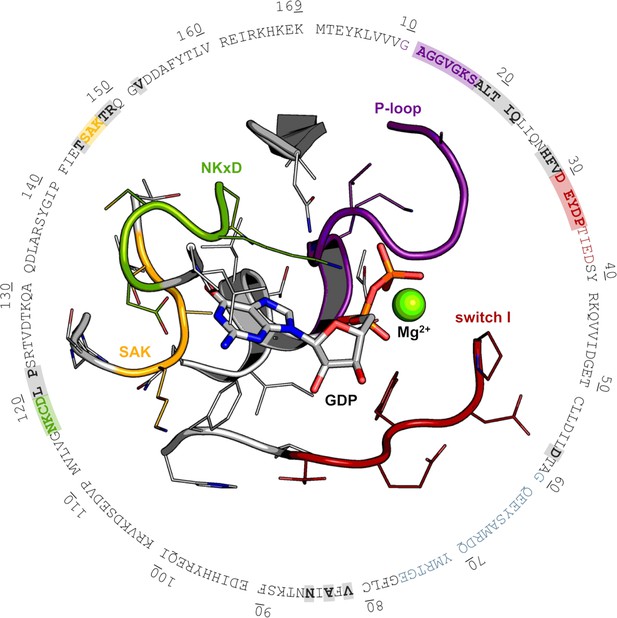
Rational design targeting KRasG13C.
Based on the crystal structure of KRasWT (PDB 4obe), aa residues that are potentially accessible via the linker design (eda:~10 Å, bda:~12 Å) are marked (grey, P-loop: violet, switch I: red, NKxD: green, SAK: yellow) and used for multiple sequence alignment of the Ras small GTPase superfamily (Figure 1—figure supplement 1, Supplementary file 1).
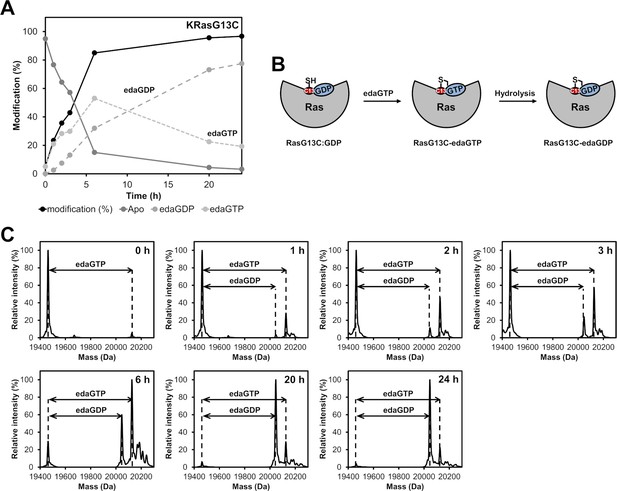
Time-dependent analysis of the covalent modification of KRasG13C.
(A) Time-resolved labelling of Ras proteins with acryl-edaGTP showing that the GTP derivative binds in the active site of Ras where it is subsequently hydrolysed. (B) Schematic representation of the GDP/GTP hydrolysis. (C) Time-dependent analysis of the covalent modification of KRasG13C proteins with edaGTP at pH 9.5 at room temperature.
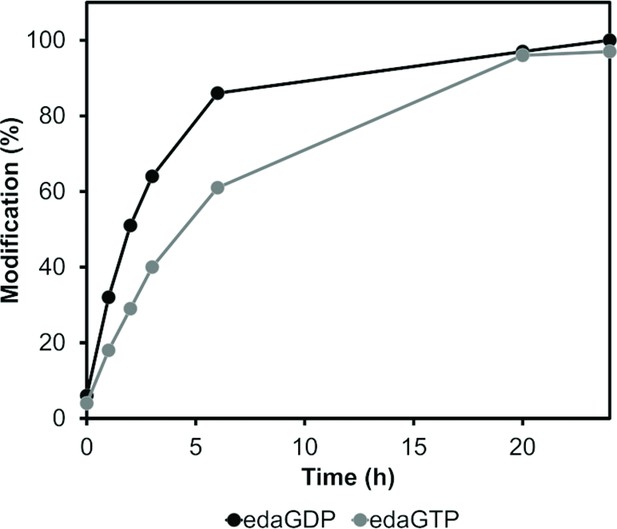
Time-dependent analysis of the covalent protein modification with edaGDP or edaGTP.
Time-resolved labelling of Ras proteins with acryl-edaGDP (black) or acryl-edaGTP (grey).
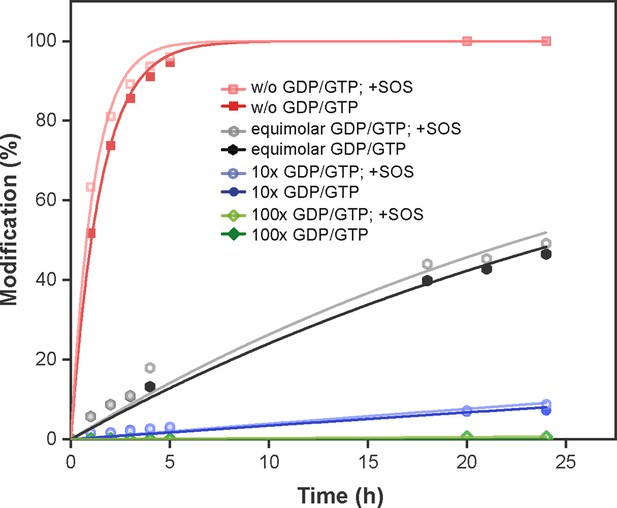
Covalent protein modification with edaGDP in the presence of competing GDP/GTP.
Labelling of KRasG13C1-169 (Cys-light) with acryl-edaGDP at pH 9.5 and room temperature was monitored after different time points by ESI-MS in the presence of equimolar concentrations and 10 x and 100 x excess of GDP/GTP.
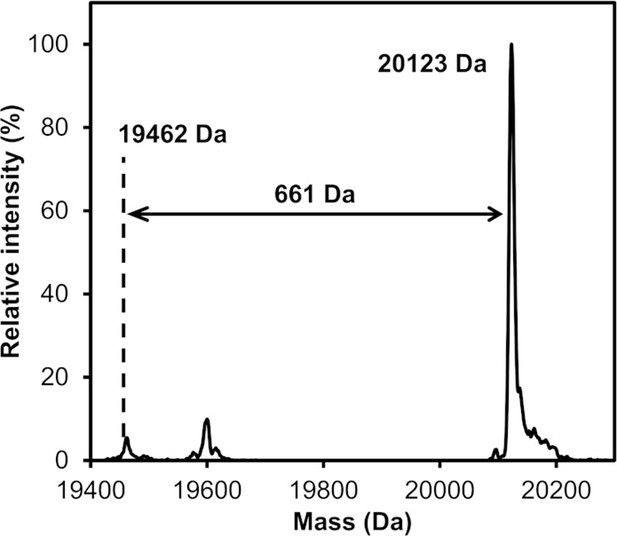
Covalent protein modification with edaGppCp.
Labelling of KRasG13C1-169 (Cys-light) with acryl-edaGppCp at pH 9.5 and room temperature was controlled after 24 hr by ESI-MS.
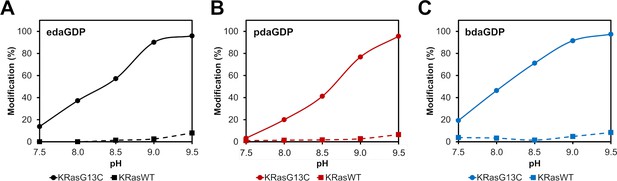
pH-dependent analysis of the covalent protein modification of KRasG13C and KRasWT.
(A, B, C) pH-dependent labelling of Ras proteins with acryl-edaGDP (black), acryl-pdaGDP (red) and acryl-bdaGDP (blue) after 24 hr. To increase the amount of covalently modified protein, the pH value was gradually increased. For KRasG13C1-169 (Cys-light), a slight labelling could already be observed at pH 7.5, which, however, could be increased to almost 100% by increasing the pH to 9.5. For KRasWT1-169 very little unspecific labelling was observed compared to the G13C mutant.
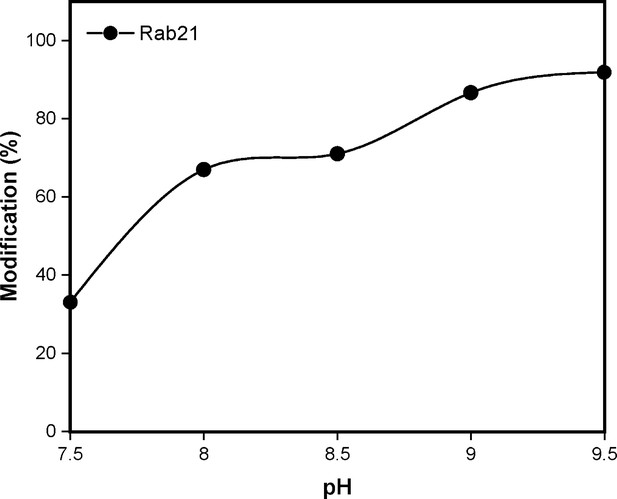
Rab21 naturally contains a cysteine at the position equivalent to KRasG13C and becomes modified by edaGDP to an extent comparable to KRasG13C.
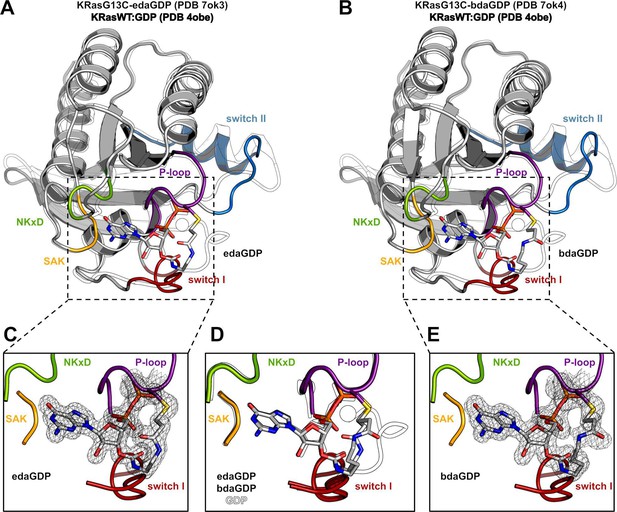
Crystal structure of KRasG13C covalently locked with either edaGDP or bdaGDP.
(A) Comparison of KRasG13C covalently locked with edaGDP (grey, P-loop: violet, switch I: red, switch II: blue, NKxD: green, SAK: yellow, PDB 7ok3) and KRas:GDP (white, PDB 4obe). (B) Comparison of KRasG13C covalently locked with bdaGDP (grey, P-loop: violet, switch I: red, switch II: blue, NKxD: green, SAK: yellow, PDB 7ok4) and KRas:GDP (white, PDB 4obe). (C) Enlarged view of the nucleotide binding pocket in KRasG13C-edaGDP showing the 2Fo-Fc electron density map (countered at 1.0 σ). (D) Structural superposition of KRas:GDP and the covalently locked G13C mutants. (E) Enlarged view of the nucleotide binding pocket in KRasG13C-bdaGDP showing the 2Fo-Fc electron density map (countered at 1.0 σ).
-
Figure 2—source data 1
Data collection and refinement statistics for KRasG13C-edaGDP and KRasG13C-bdaGDP.
- https://cdn.elifesciences.org/articles/82184/elife-82184-fig2-data1-v2.docx
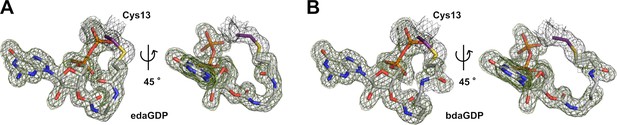
Structural insights into the binding mode of nucleotide-based covalent inhibitors.
(A, B) edaGDP and bdaGDP, respectively, covalently bound to KRasG13C including the FO-FC simulated annealing omit map (green, r.m.s.d.=2.5) and the 2FO-FC map (grey, r.m.s.d.=1.0).
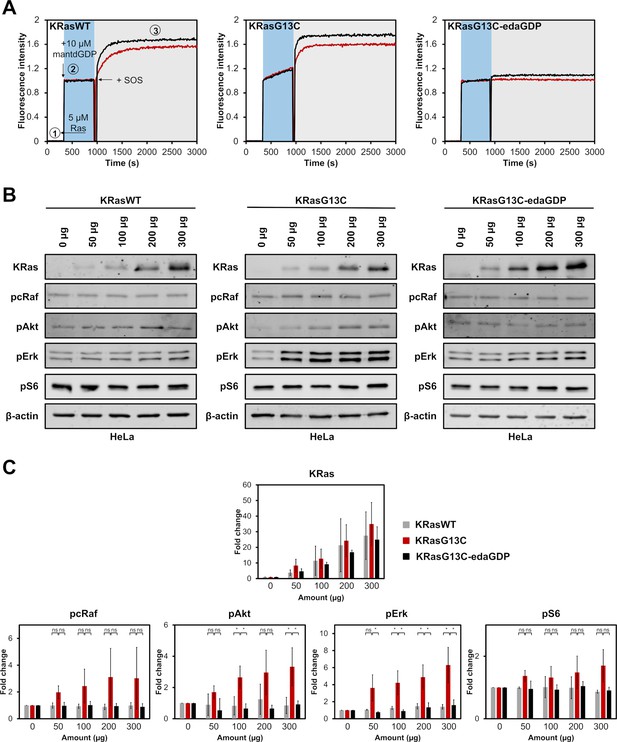
Cellular evaluation of nucleotide-based covalent inhibitors.
(A) GEF-catalysed nucleotide exchange of KRasWT:GDP, KRasG13C:GDP and KRasG13C-edaGDP (step 1) which were mixed with an excess of mantdGDP (step 2) and subsequently with 0.25 µM (red curve) or 0.5 µM (black curve) of SOS (step 3). The intrinsic nucleotide exchange is depicted in the blue box whereas the SOS-catalysed nucleotide exchange is shown in the grey box. (B) Western blot analysis after electroporation of indicated amounts of full-length KRasWT, KRasG13C, and KRasG13C-edaGDP into HeLa cells. (C) Quantification of protein levels from western blots was performed using Empiria Studio (Li-Cor). The mean fold change was plotted against increasing amounts of protein. Error bars indicate the standard deviation for each measurement (n=3). One-way ANOVA was performed using GraphPad Prism. The uncropped western blots are available as source data (Goebel et al_Source_data_file_WB.xlsx).
-
Figure 3—source data 1
Original uncropped Western Blots for data in Figure 3.
- https://cdn.elifesciences.org/articles/82184/elife-82184-fig3-data1-v2.zip
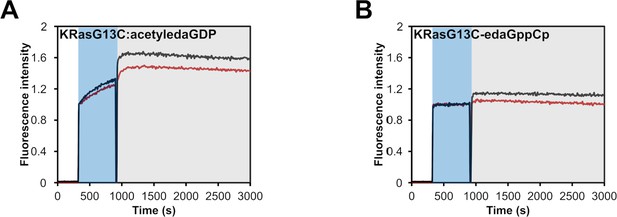
GEF-catalysed nucleotide exchange.
SOS-catalysed nucleotide exchange of (A) KRasG13C:acetyledaGDP and (B) KRasG13C-edaGppCp which were mixed with an excess of mantdGDP and subsequently with 0.25 µM (red curve) or 0.5 µM (black curve) of SOS. The intrinsic nucleotide exchange is depicted in the blue box whereas the SOS-catalysed nucleotide exchange is shown in the grey box.
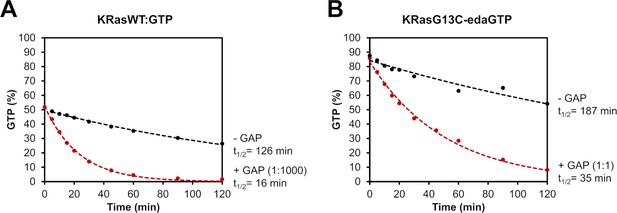
GAP-stimulated GTP hydrolysis.
(A) The intrinsic (black) and the GAP-stimulated (red) GTP hydrolysis of KRasWT:GTP was measured via HPLC. (B) The intrinsic (black) and the GAP-stimulated (red) GTP hydrolysis of KRasG13C-edaGTP was obtained via ESI-MS. While GAP-stimulated GTP hydrolysis can be observed for KRasWT:GTP already at catalytic amounts of GAP (GAP 1:1000, t1/2 = 16 min), KRasG13C-edaGTP requires equivalent amounts of GAP (GAP 1:1, t1/2 = 35 min). However, the intrinsic GTP hydrolysis of KRasG13C-edaGTP (t1/2 = 187 min) is comparable to that of KRasWT:GTP (t1/2 = 126 min).
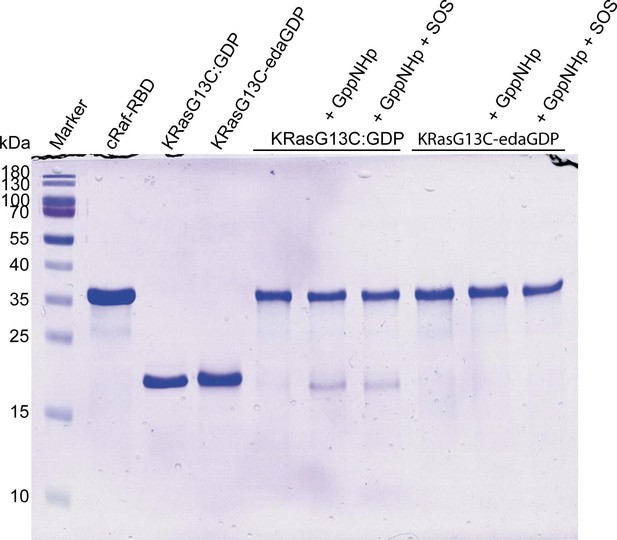
Effector-binding of KRasG13C is precluded upon covalent modification with edaGDP.
Whereas RasG13C:GDP can be pulled down by GST-tagged RafRBD in the presence of GppNHp and GppNHp/SOS, RasG13C-edaGDP cannot become activated and consequently cannot bind to RafRBD, neither in the presence of GppNHp nor GppNHp and SOS.
-
Figure 3—figure supplement 3—source data 1
This is the original uncropped SDS-gel, however since it does not differ from Figure 3—figure supplement 3.
- https://cdn.elifesciences.org/articles/82184/elife-82184-fig3-figsupp3-data1-v2.zip
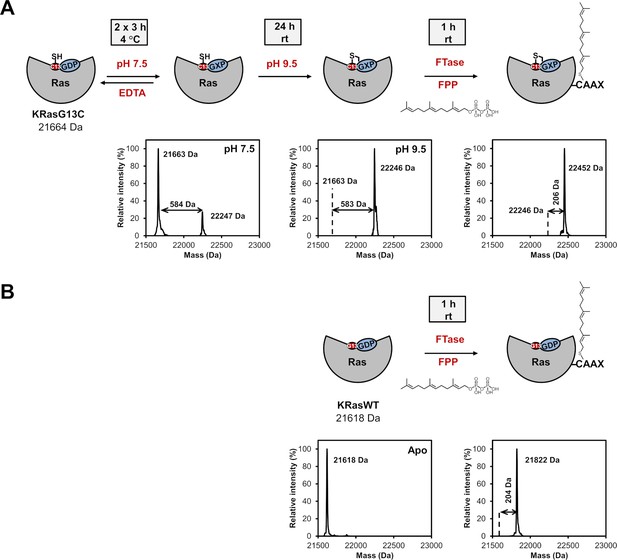
Labelling and in vitro farnesylation experiments with full-length KRas.
(A) Schematic representation of covalent modification of full-length KRasG13C followed by in vitro farnesylation. Nucleotide exchange was performed in the presence of EDTA at pH 7.5 followed by covalent modification of full-length KRasG13C at pH 9.5 to prevent undesired interaction with the cysteine of the CAAX box. To verify the position of the covalent modification, an in vitro farnesylation was afterwards performed, showing complete farnesylation and thus showing that the cysteine of the important CAAX box at the C-terminus of Ras is available for farnesylation and did not react unspecifically with the nucleotide. (B) Schematic representation of in vitro farnesylation of full-length KRasWT as a control experiment.
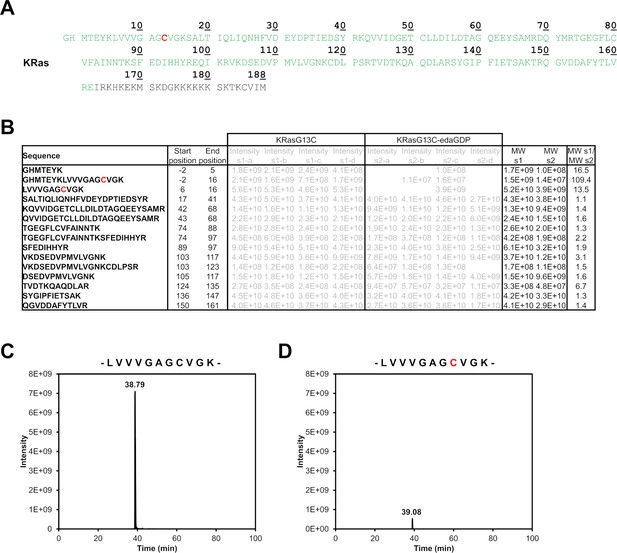
MS/MS analysis to identify the site of modification in KRasG13C.
(A) Overview of the sequence coverage. The identified regions of the protein are marked in green, and only the C-terminus of the protein cannot be identified due to the multiple lysine side chains present in this region. (B) Comparison of the intensities of all peptides of KRas after tryptic digestion, which were detected in all four replicates of the unmodified protein with an intensity of at least 1E8. Peptide intensities of the unmodified protein and the modified one are comparable from position 16 on, whereas the intensities of the peptides containing the targeted cysteine residue were significantly lower. By calculating the mean values of the intensities for the replicates of the unmodified (s1) and modified proteins (s2) followed by calculating the ratio of the mean values (MW s1/MW s2), we observed that the N terminus up to position 16 in the samples s2 could not be identified or was detected with much lower intensity than the other detectable regions of KRas. Thus, MS/MS analysis suggests that the targeted cysteine residue at position 13 is labelled by the nucleotide-based inhibitor edaGDP. (C, D) Comparison of the intensities of peptide LVVVGAGCVGK (m/z: 529.805+–5 ppm, twofold charged) for KRasG13C and KRasG13C-edaGDP. (D) Ion chromatogram of the peptide for KRasG13C. (D) Ion chromatogram of the peptide for KRasG13C-edaGDP.
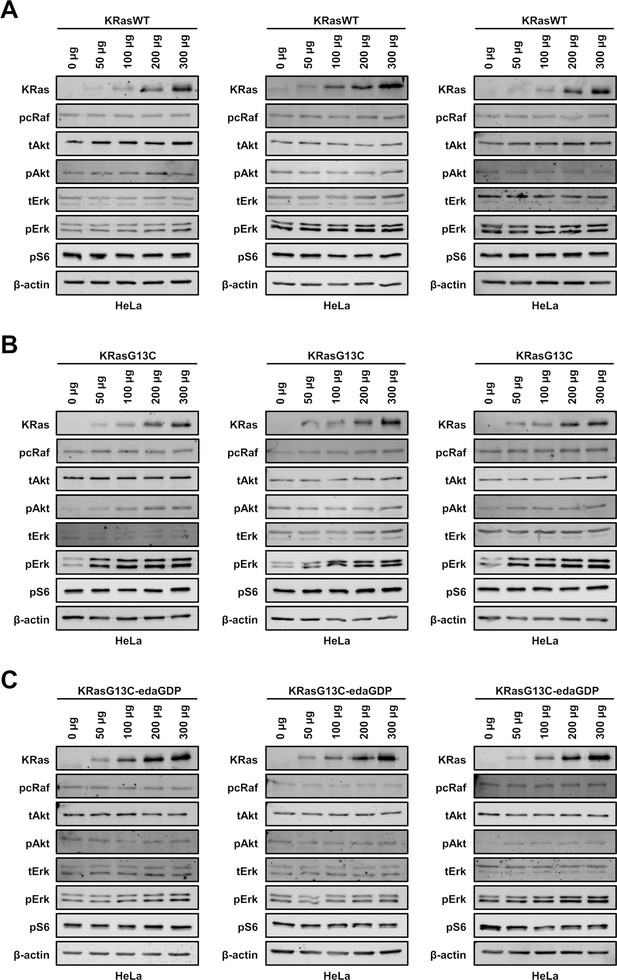
In vitro immunoblotting experiments.
Western blot analysis after electroporation of indicated amounts of full-length (A) KRasWT, (B) KRasG13C and (C) KRasG13C-edaGDP into HeLa cells (n=3 biological replicates). The uncropped western blots are available as source data (Goebel et al_Source_data_file_WB.xlsx).
-
Figure 3—figure supplement 6—source data 1
Original uncropped Western Blots for data in Figure 3—figure supplement 6.
- https://cdn.elifesciences.org/articles/82184/elife-82184-fig3-figsupp6-data1-v2.zip
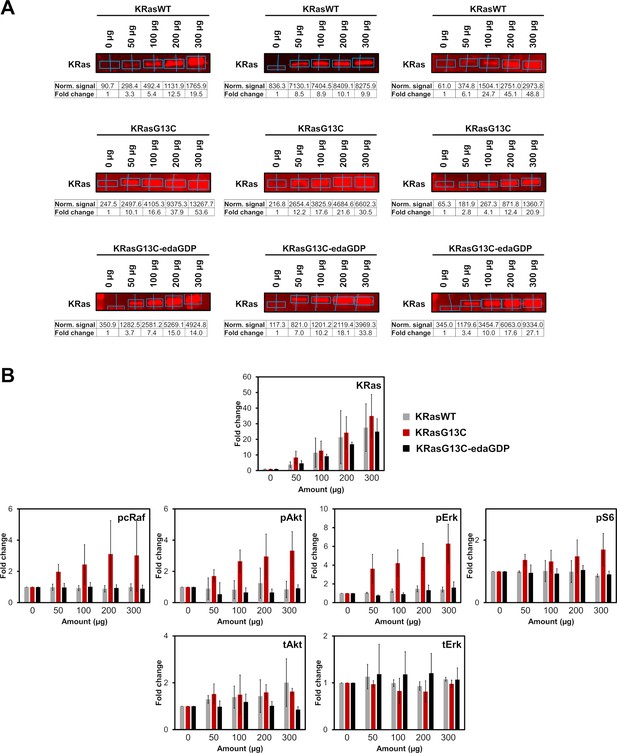
Western blot analysis and quantification.
Quantification of (A) KRas and (B) downstream protein levels from western blots after electroporation of indicated amounts of full-length KRasWT, KRasG13C and KRasG13C-edaGDP into HeLa cells using Empiria Studio (Li-Cor). The mean fold change was plotted against increasing amounts of protein. Error bars indicate the standard deviation for each measurement (n=3). Because of the relatively low expression levels of Ras, the contrast needed to be appropriately increased to enable visualisation of the intrinsically expressed KRas protein. The uncropped western blots are available as source data (Goebel et al_Source_data_file_WB.xlsx).
-
Figure 3—figure supplement 7—source data 1
Original uncropped Western Blots for data in Figure 3—figure supplement 7.
- https://cdn.elifesciences.org/articles/82184/elife-82184-fig3-figsupp7-data1-v2.zip
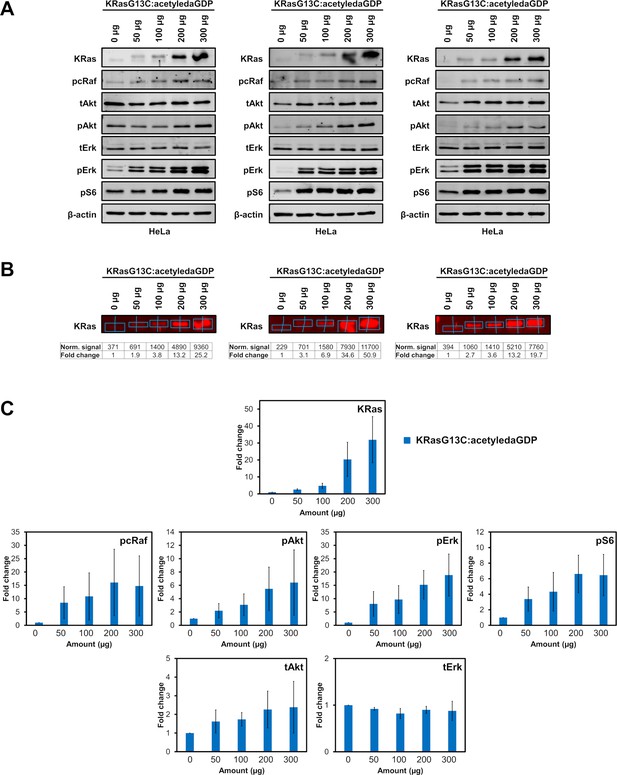
Electroporation of KRasG13C:acetyledaGDP.
(A) Western blot analysis after electroporation of indicated amounts of full-length KRasG13C:acetyledaGDP into HeLa cells (n=3 biological replicates). (B) Quantification of KRas and (C) downstream protein levels from western blots after electroporation of indicated amounts of full-length KRasG13C:acetyledaGDP (blue) into HeLa cells using Empiria Studio (Li-Cor). The mean fold change was plotted against increasing amounts of protein. Error bars indicate the standard deviation for each measurement (n=3). The uncropped western blots are available as source data (Goebel et al_Source_data_file_WB.xlsx).
-
Figure 3—figure supplement 8—source data 1
Original uncropped Western Blots for data in Figure 3—figure supplement 8.
- https://cdn.elifesciences.org/articles/82184/elife-82184-fig3-figsupp8-data1-v2.zip
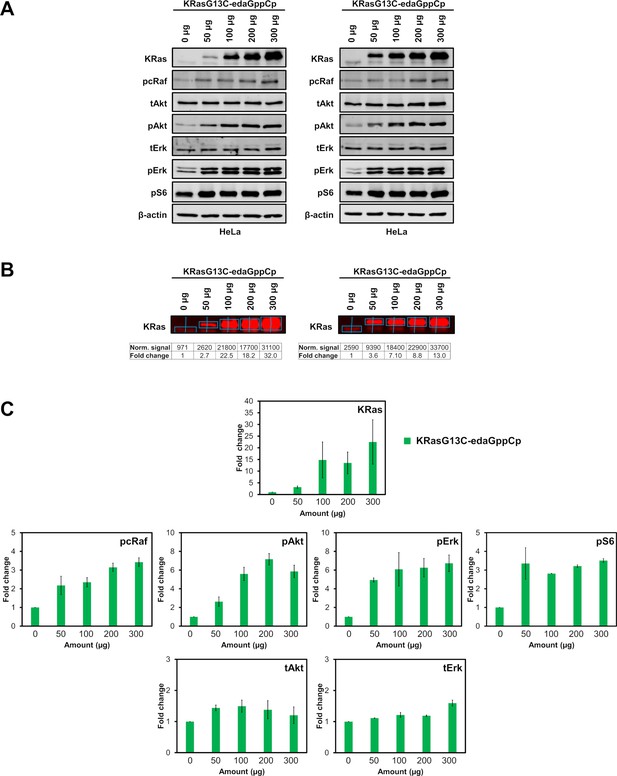
Electroporation of KRasG13C-edaGppCp.
(A) Western blot analysis after electroporation of indicated amounts of full-length KRasG13C-edaGppCp into HeLa cells (n=2 biological replicates). (B) Quantification of KRas and (C) downstream protein levels from western blots after electroporation of indicated amounts of full-length KRasG13C-edaGppCp (green) into HeLa cells using Empiria Studio (Li-Cor). The mean fold change was plotted against increasing amounts of protein. Error bars indicate the standard deviation for each measurement (n=2). The uncropped western blots are available as source data (Goebel et al_Source_data_file_WB.xlsx).
-
Figure 3—figure supplement 9—source data 1
Original uncropped Western Blots for data in Figure 3—figure supplement 9.
- https://cdn.elifesciences.org/articles/82184/elife-82184-fig3-figsupp9-data1-v2.zip
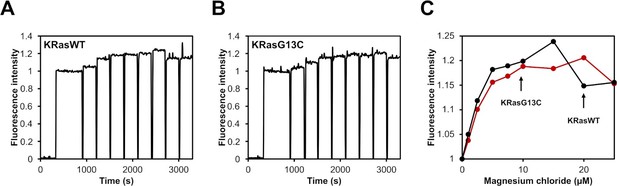
Mg2+ affinity assay.
(A, B) Fluorescence titration of KRasWT:mantdGDP or KRasG13C:mantdGDP with increasing concentrations of Mg2+ (1, 2.5, 5, 7.5, 10, 15, 20, and 25 μM); (C) The resulting curves were analysed by plotting the relative fluorescence intensity vs. MgCl2 concentration.
Tables
Overview of the calculated kinetic parameters (KD, kon and koff).
KD[pM] | kon[µM–1s–1] | koff[s–1] | |
---|---|---|---|
GDP | 2.5 | 4.22 | 1.1x10–5 |
pdaGDP | 8.6±1.3 | 3.34 | 2.9x10–5 |
bdaGDP | 9.6±0.5 | 3.12 | 3.0x10–5 |
SML-8-73-1 | ~140 nM | - | - |
-
Table 1—source data 1
Kinetics of the nucleotide association (kon).
(A) Competitive binding experiments of GDP and mantdGDP; 1 μM KRas and 2 μM mantdGDP was used in the absence (black curve) or in the presence of 1 µM (red), 2 µM (blue) or 6 µM (green) competing nucleotides in a stopped-flow instrument. (B) The binding curves were globally fit to the indicated model using KinTek Explorer to obtain the corresponding association rates (kon). (C, D, E) Competitive binding experiments between mantdGDP and GDP, GTP, or dGTP analogues. Calculated kon values are shown in Table 1—source data 2.
- https://cdn.elifesciences.org/articles/82184/elife-82184-table1-data1-v2.zip
-
Table 1—source data 2
kon calculation.
Overview of kon rate constants obtained from competitive binding experiments with mantdGDP (Table 1—source data 1).
- https://cdn.elifesciences.org/articles/82184/elife-82184-table1-data2-v2.zip
-
Table 1—source data 3
HPLC-based approach for determination of affinities relative to GDP.
(A, B, C) Competitive binding experiments of GDP and the acetyl-derivatives of GDP; 50 μM KRasWT:GDP was mixed with 50 µM of edaGDP, pdaGDP or bdaGDP and incubated for 7 days at room temperature in the absence of EDTA, for 24 hr at 4 °C in the presence of 10 mM EDTA or for 1 hr in the presence of SOS (0.5 µM). After buffer exchange for removal of any unbound nucleotides, the resulting mixtures were analyzed by isocratic HPLC runs. (D) Relative amounts of the nucleotides and GDP bound to KRas were compared. It should be noted that the relative affinities mentioned in the method section and shown in Table 1—source data 4 are calculated from these experiments and represent an average affinity of the 2’ isomers, and that of the 3’-isomer seen to be bound in the X-ray structure is presumably actually higher than this, since it can be seen from Figure 2—figure supplement 1 that there is a preference for one of the isomers, presumably the 3’-isomer, so that this species and the derivatives of dGDP/dGTP must have a very similar affinity to that of GDP/GTP.
- https://cdn.elifesciences.org/articles/82184/elife-82184-table1-data3-v2.zip
-
Table 1—source data 4
KD calculations.
Overview of the calculated KD values of pdaGDP and edaGDP obtained from an HPLC-based approach (Table 1—source data 3); For reference, the KD values of GDP and the nucleotide analogue SML-8-73-1 are also listed.
- https://cdn.elifesciences.org/articles/82184/elife-82184-table1-data4-v2.zip
Additional files
-
Supplementary file 1
Sequence alignment of Ras small GTPase superfamily.
Multiple sequence alignment of the G domain Ras superfamily members using Uniprot (the uniport accession codes can be taken from this figure). Based on the marked areas that are shown in Figure 1—figure supplement 1, cysteines that are within the reach of the warhead of the eda linker are highlighted in gray. In contrast, regions that are also within reach of the bda linker are highlighted in blue. The cysteines located within these regions are marked in red. Only ~7% of the GTPases have cysteines within the P-loop and are therefore potential off-targets of the designed nucleotide derivatives.
- https://cdn.elifesciences.org/articles/82184/elife-82184-supp1-v2.docx
-
Supplementary file 2
pKa calculations.
Overview of calculated pKa values of the KRasG12C and G13C mutants in the presence (+) and absence (-) of GDP. The linker in case of the G13C mutants was removed so that all featured structures had GDP and a free cysteine in its active center. The pKa calculations showed that both cysteines at position 12 and 13 have similar pKa values indicating that also position 13 should generally be addressable by covalent warheads.
- https://cdn.elifesciences.org/articles/82184/elife-82184-supp2-v2.docx
-
MDAR checklist
- https://cdn.elifesciences.org/articles/82184/elife-82184-mdarchecklist1-v2.pdf
-
Source data 1
Summary of all uncropped western blots used in Figure 3 and the Figure supplements.
- https://cdn.elifesciences.org/articles/82184/elife-82184-data1-v2.xlsx