Hypoxia truncates and constitutively activates the key cholesterol synthesis enzyme squalene monooxygenase
Figures
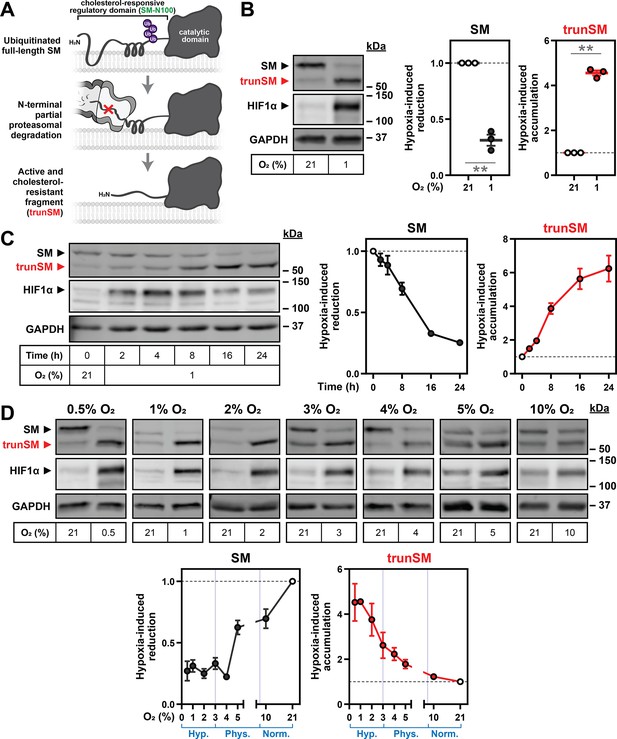
Oxygen availability regulates SM truncation.
(A) Simplified overview of SM truncation. Full-length SM contains an N-terminal domain mediating feedback regulation by cholesterol. Ubiquitinated SM is targeted to the proteasome, where proteolysis is prematurely halted within the regulatory domain. This liberates a truncated protein (trunSM) that no longer responds to cholesterol and is therefore constitutively active. (B) HEK293T cells were incubated under normoxic (21% O2) or hypoxic (1% O2) conditions for 24 hr. (C) HEK293T cells were incubated under normoxic or hypoxic conditions for the indicated times. Changes in HIF1α levels over time are consistent with other reports (Jantsch et al., 2011; Bartoszewski et al., 2019). (D) HEK293T cells were incubated under the indicated oxygen concentrations for 24 hr. Each set of immunoblots was obtained in a separate experiment. (B–D) Immunoblotting was performed for SM and trunSM (red). Graphs depict densitometric quantification of SM and trunSM protein levels normalized to the normoxic condition, which was set to 1 (dotted line). In (D), oxygen concentrations considered hypoxic (hyp.), ‘physoxic’ (phys.) or normoxic (norm.) (McKeown, 2014) are indicated in blue. Data presented as mean ± SEM from n=3–4 independent experiments (**, p≤0.01; two-tailed one-sample t-test vs. hypothetical mean of 1).
-
Figure 1—source data 1
Uncropped immunoblots for Figure 1.
- https://cdn.elifesciences.org/articles/82843/elife-82843-fig1-data1-v1.zip
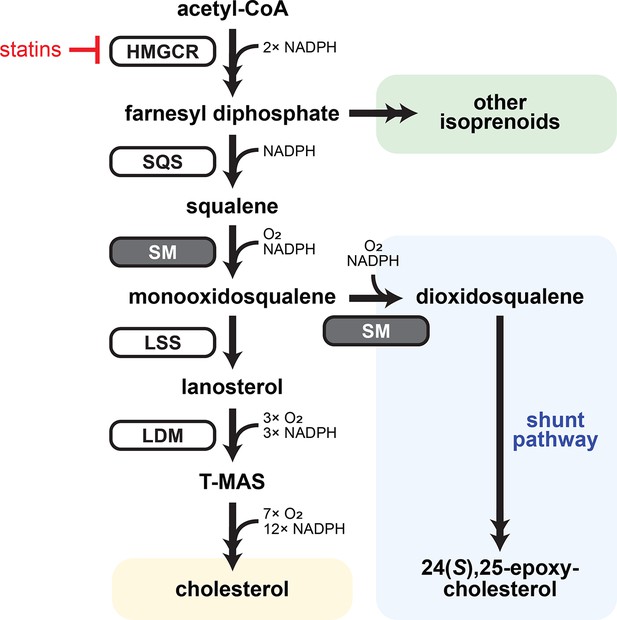
Simplified schematic of the cholesterol synthesis pathway.
Early cholesterol synthesis overlaps with the mevalonate pathway, which produces farnesyl diphosphate and other isoprenoid precursors. Its rate-limiting enzyme is HMG-CoA reductase (HMGCR), the target of statins. The late cholesterol synthesis pathway is committed to sterol production and begins with squalene synthase (SQS). Squalene is converted to monooxidosqualene by the rate-limiting enzyme squalene monooxygenase (SM, grey) and cyclized by lanosterol synthase (LSS). Lanosterol is then converted by lanosterol 14α-demethylase (LDM) to testis meiosis-activating sterol (T-MAS), which is ultimately converted to cholesterol. A second round of SM-catalyzed epoxidation converts monooxidosqualene to dioxidosqualene, the precursor for a parallel ‘shunt’ pathway producing the regulatory oxysterol 24(S),25-epoxycholesterol. Cholesterol synthesis is energy intensive and consumes a total of 11 O2 molecules: one by SM, three by LDM, and seven by downstream enzymes. Double-headed arrows indicate multiple enzymatic steps.
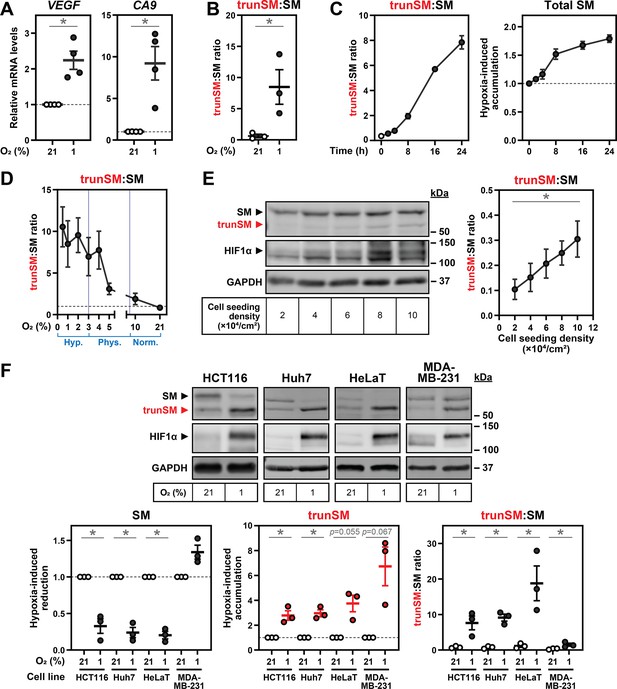
Hypoxia-induced truncation of SM is generalizable.
(A) HEK293T cells were incubated under normoxic (21% O2) or hypoxic (1% O2) conditions for 24 hr. Levels of the indicated HIF1α target gene transcripts were quantified, normalized to the levels of RPL11, GAPDH and ACTB housekeeping transcripts and adjusted relative to the normoxic condition, which was set to 1 (dotted line). (B) Quantification of trunSM:SM ratio in Figure 1B. (C) Quantification of trunSM:SM ratio and total SM levels (expressed as the sum of SM and trunSM levels) in Figure 1C. (D) Quantification of trunSM:SM ratio in Figure 1D. (E) HEK293T cells were seeded at the indicated densities and incubated for 48 hr. Graph depicts densitometric quantification of trunSM:SM ratio. (F) The indicated cell lines were incubated under normoxic or hypoxic conditions for 24 hr. (C, F) Graphs depict densitometric quantification of protein levels normalized to the respective normoxic conditions for each cell line, which were set to 1 (dotted line). (A–F) Data presented as mean ± SEM from n=3–4 independent experiments (*, p≤0.05; [A, F] two-tailed one-sample t-test vs. hypothetical mean of 1; [B, E, F] two-tailed ratio paired t-test).
-
Figure 1—figure supplement 2—source data 1
Uncropped immunoblots for Figure 1—figure supplement 2.
- https://cdn.elifesciences.org/articles/82843/elife-82843-fig1-figsupp2-data1-v1.zip
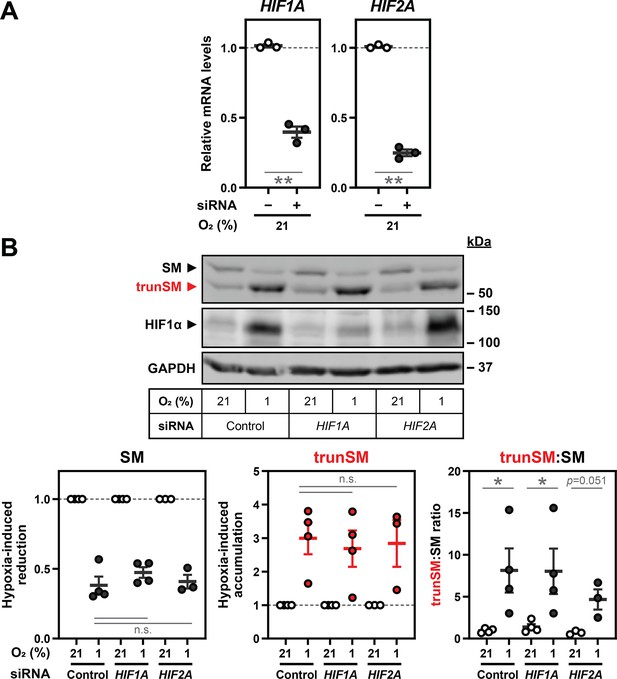
Hypoxia-induced truncation of SM is independent of hypoxia-inducible factors.
HEK293T cells were transfected with the indicated siRNAs for 24 hr and incubated under normoxic or hypoxic conditions for 24 hr. (A) Levels of siRNA target transcripts in normoxic cells were quantified, normalized to the levels of PBGD housekeeping transcripts and adjusted relative to the control siRNA condition, which was set to 1 (dotted line). (B) Graphs depict densitometric quantification of protein levels normalized to the respective normoxic conditions for each siRNA, which were set to 1 (dotted line). (A, B) Data presented as mean ± SEM from n=3–4 independent experiments (*, p≤0.05; **, p≤0.01; [A] two-tailed one-sample t-test vs. hypothetical mean of 1; [B] two-tailed ratio paired t-test).
-
Figure 1—figure supplement 3—source data 1
Uncropped immunoblots for Figure 1—figure supplement 3.
- https://cdn.elifesciences.org/articles/82843/elife-82843-fig1-figsupp3-data1-v1.zip
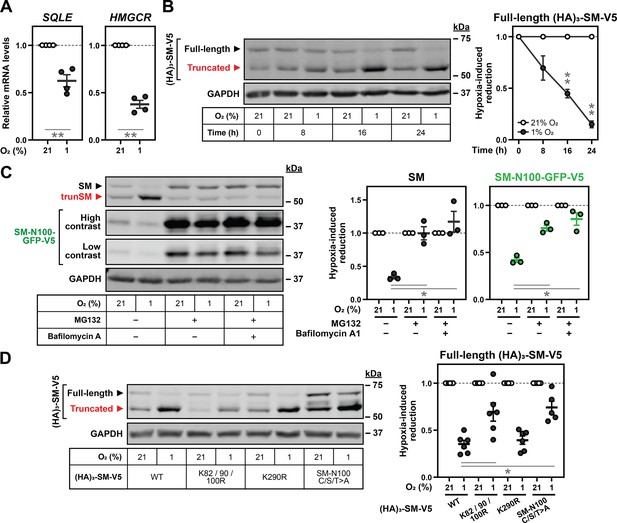
Hypoxia transcriptionally and post-translationally reduces full-length SM levels.
(A) HEK293T cells were incubated under normoxic or hypoxic conditions for 24 hr. Levels of the indicated transcripts were quantified, normalized to the levels of RPL11, GAPDH and ACTB housekeeping transcripts and adjusted relative to the normoxic condition, which was set to 1 (dotted line). (B) HEK293T cells were transfected with (HA)3-SM-V5 for 24 hr and incubated under normoxic or hypoxic conditions for the indicated times. (C) HEK SM-N100-GFP-V5 cells were treated with or without 20 µM MG132 and 20 nM bafilomycin A1 under normoxic or hypoxic conditions for 16 hr. (D) HEK293T cells were transfected with the indicated constructs for 24 hr and incubated under normoxic or hypoxic conditions for 16 hr. (B–D) Graphs depict densitometric quantification of protein levels normalized to the respective normoxic conditions for each timepoint, treatment or construct, which were set to 1 (dotted line). (A–D) Data presented as mean ± SEM from n=3–6 independent experiments (*, p≤0.05; **, p≤0.01; [A, B] two-tailed one-sample t-test vs. hypothetical mean of 1; [C, D] two-tailed ratio paired t-test).
-
Figure 2—source data 1
Uncropped immunoblots for Figure 2.
- https://cdn.elifesciences.org/articles/82843/elife-82843-fig2-data1-v1.zip
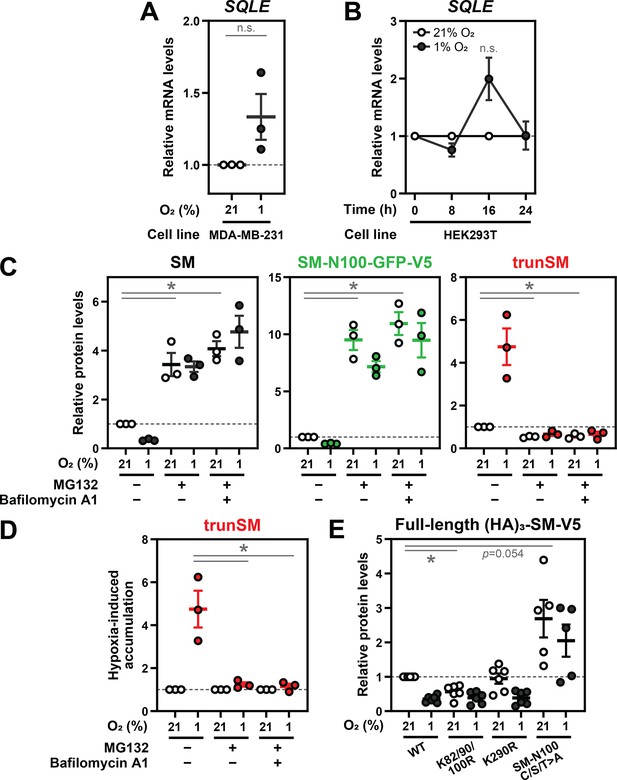
Hypoxia-induced degradation of full-length SM is via the ubiquitin-proteasome system.
(A) MDA-MB-231 cells were incubated under normoxic or hypoxic conditions for 24 hr. (B) HEK293T cells were treated as described in Figure 2B. (A, B) Levels of SQLE transcripts were quantified, normalized to the levels of RPL11, GAPDH and ACTB housekeeping transcripts and adjusted relative to the normoxic condition, which was set to 1 (dotted line). (C) Quantification of SM, SM-N100-GFP-V5 and trunSM levels in Figure 2C normalized to the normoxic vehicle condition, which was set to 1 (dotted line). (D) Quantification of trunSM levels in Figure 2C normalized to the respective normoxic conditions for each treatment, which were set to 1 (dotted line). (E) Quantification of full-length (HA)3-SM-V5 SM levels in Figure 2D normalized to the normoxic wild-type condition, which was set to 1 (dotted line). (A–E) Data presented as mean ± SEM from n=3–6 independent experiments (*, p≤0.05; [A–C, E] two-tailed one-sample t-test vs. hypothetical mean of 1; [D] two-tailed ratio paired t-test).
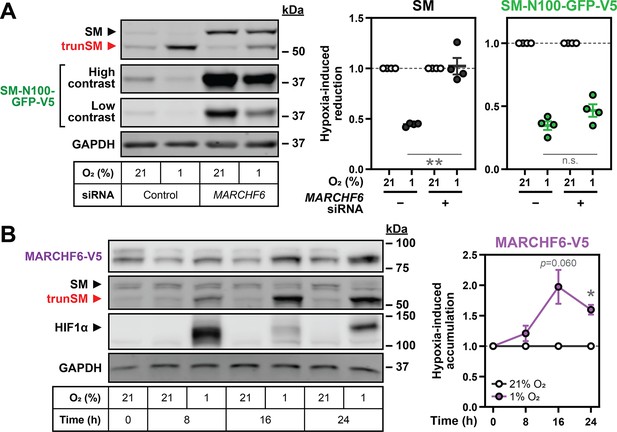
Hypoxia-induced degradation of full-length SM requires the E3 ubiquitin ligase MARCHF6.
(A) HEK SM-N100-GFP-V5 cells were transfected with control or MARCHF6 siRNA for 24 hr and incubated under normoxic or hypoxic conditions for 16 hr. (B) HEK MARCHF6-V5 cells were incubated under normoxic or hypoxic conditions for the indicated times. MARCHF6-V5 appears as two bands that were quantified collectively, as we have done previously (Sharpe et al., 2019). (A, B) Graphs depict densitometric quantification of protein levels normalized to the respective normoxic condition for each siRNA or timepoint, which were set to 1 (dotted line). Data presented as mean ± SEM from n=3–4 independent experiments (*, p≤0.05; **, p≤0.01; [A] two-tailed ratio paired t-test; [B] two-tailed one-sample t-test vs. hypothetical mean of 1).
-
Figure 3—source data 1
Uncropped immunoblots for Figure 3.
- https://cdn.elifesciences.org/articles/82843/elife-82843-fig3-data1-v1.zip
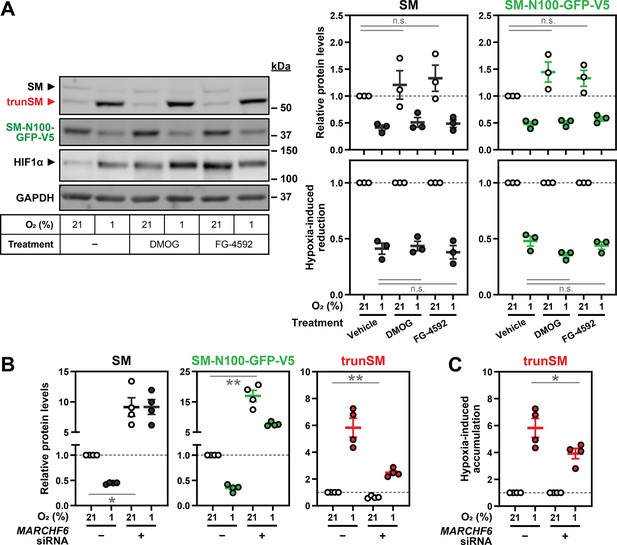
Hypoxia-induced degradation of full-length SM is independent of proline hydroxylation.
(A) HEK SM-N100-GFP-V5 cells were treated with or without 1 mM DMOG or 25 µM FG-4592 under normoxic or hypoxic conditions for 16 hr. Graphs depict densitometric quantification of protein levels normalized to the normoxic vehicle condition (top row) or respective normoxic conditions for each treatment (bottom row), which were set to 1 (dotted line). (B) Quantification of SM, SM-N100-GFP-V5 and trunSM levels in Figure 3A normalized to the normoxic vehicle condition, which was set to 1 (dotted line). (C) Quantification of trunSM levels in Figure 3A normalized to the respective normoxic conditions for each siRNA, which were set to 1 (dotted line). (A–C) Data presented as mean ± SEM from n=3–4 independent experiments (*, p≤0.05; **, p≤0.01; [A top row, B] two-tailed one-sample t-test vs. hypothetical mean of 1; [A bottom row, C] two-tailed ratio paired t-test).
-
Figure 3—figure supplement 1—source data 1
Uncropped immunoblots for Figure 3—figure supplement 1.
- https://cdn.elifesciences.org/articles/82843/elife-82843-fig3-figsupp1-data1-v1.zip
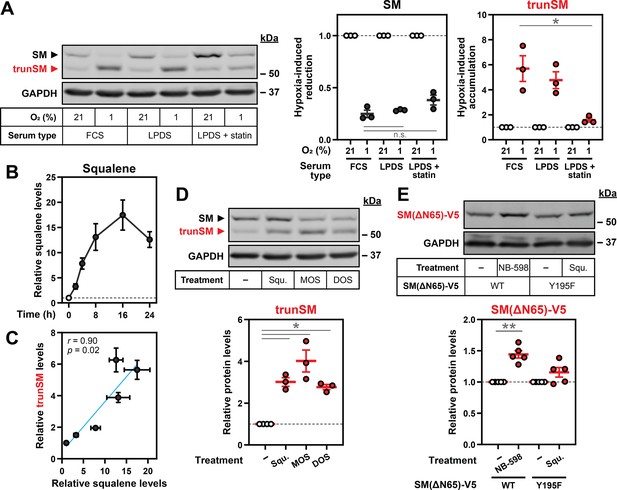
Hypoxia-induced squalene accumulation promotes partial degradation of SM.
(A) HEK293T cells were incubated in medium containing fetal calf serum (FCS), lipoprotein-deficient FCS (LPDS) or LPDS containing 5 µM mevastatin and 50 µM mevalonolactone (LPDS +statin) for 8 hr, refreshed in their respective medium and incubated under normoxic or hypoxic conditions for 16 hr. (B) HEK293T cells were incubated under normoxic or hypoxic conditions for the indicated times. Non-saponifiable lipids were extracted, and squalene levels were determined using gas chromatography-mass spectrometry and adjusted relative to the normoxic condition, which was set to 1 (dotted line). The maximal squalene level detected was 0.66±0.12 ng per µg of total protein. (C) Pearson correlation between squalene levels in (B) and trunSM levels in Figure 1C. Blue line indicates linear regression. (D) HEK293T cells were treated with or without 300 µM squalene (squ.), monooxidosqualene (MOS) or dioxidosqualene (DOS) for 16 hr. (E) HEK293T SQLE-knockout (SQLE-KO) clone 10 (c10) cells were transfected with the indicated constructs for 24 hr, then treated with or without 1 µM NB-598 or 300 µM squalene for 16 hr. (A, D, E) Graphs depict densitometric quantification of trunSM or truncated protein levels normalized to the (A) respective normoxic conditions for each serum type or (D, E) vehicle conditions, which were set to 1 (dotted line). (A–E) Data presented as mean ± SEM from n=3–5 independent experiments (*, p≤0.05; **, p≤0.01; [A] two-tailed ratio paired t-test; [D, E] two-tailed one-sample t-test vs. hypothetical mean of 1).
-
Figure 4—source data 1
Uncropped immunoblots for Figure 4.
- https://cdn.elifesciences.org/articles/82843/elife-82843-fig4-data1-v1.zip
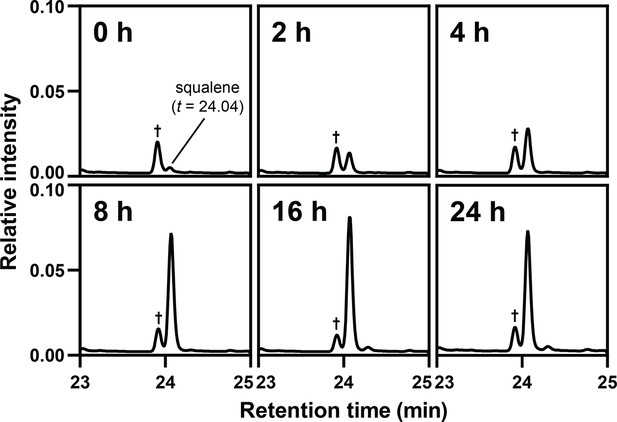
Hypoxia induces squalene accumulation.
Representative selective ion monitoring chromatogram traces from gas chromatography-mass spectrometry analysis of squalene levels in Figure 4B. Abundance was normalized to the 5α-cholestane internal standard, which was set to 1. Dagger indicates a non-specific analyte.
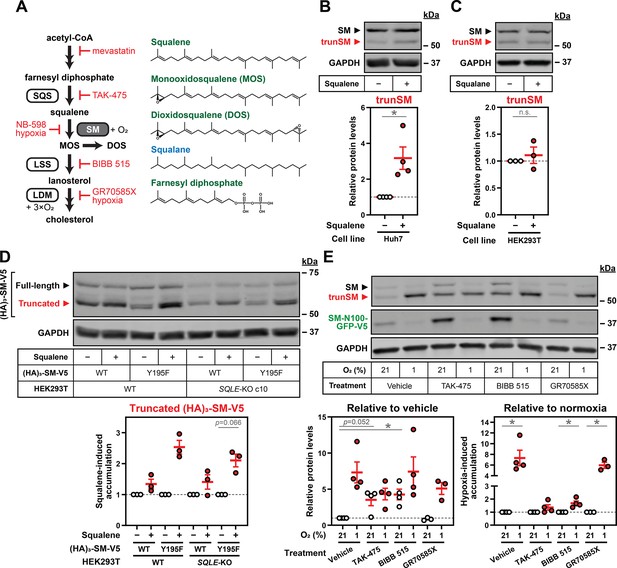
Farnesyl-containing cholesterol synthesis intermediates specifically promote partial degradation of SM.
(A) Simplified schematic of the cholesterol synthesis pathway depicting activities of squalene synthase (SQS), SM, lanosterol synthase (LSS) and lanosterol 14α-demethylase (LDM), alongside chemical structures of squalene, monooxidosqualene (MOS), dioxidosqualene (DOS), squalane, and farnesyl diphosphate. Double-headed arrows indicate multiple enzymatic steps, red labels indicate cholesterol synthesis inhibitors, and green and blue labels indicate molecules found to promote or have no effect on trunSM accumulation, respectively. (B) Huh7 cells were treated with or without 300 µM squalene for 16 hr. (C) HEK293T cells were treated with or without 300 µM squalane for 16 hr. (D) Parental (WT) or HEK293T SQLE-knockout (SQLE-KO) clone 10 (c10) cells were transfected with the indicated constructs for 24 hr, then treated with or without 300 µM squalene for 16 hr. (E) HEK SM-N100-GFP-V5 cells were treated with the indicated compounds under normoxic or hypoxic conditions for 16 hr. (B–E) Graphs depict densitometric quantification of protein levels normalized to the (B–D) respective vehicle conditions for each treatment or construct, (E left) normoxic vehicle condition, or (E right) respective normoxic conditions for each treatment, which were set to 1 (dotted line). Data presented as mean ± SEM from n=3–4 independent experiments (*, p≤0.05); two-tailed one-sample t-test vs. hypothetical mean of 1.
-
Figure 4—figure supplement 2—source data 1
Uncropped immunoblots for Figure 4—figure supplement 2.
- https://cdn.elifesciences.org/articles/82843/elife-82843-fig4-figsupp2-data1-v1.zip
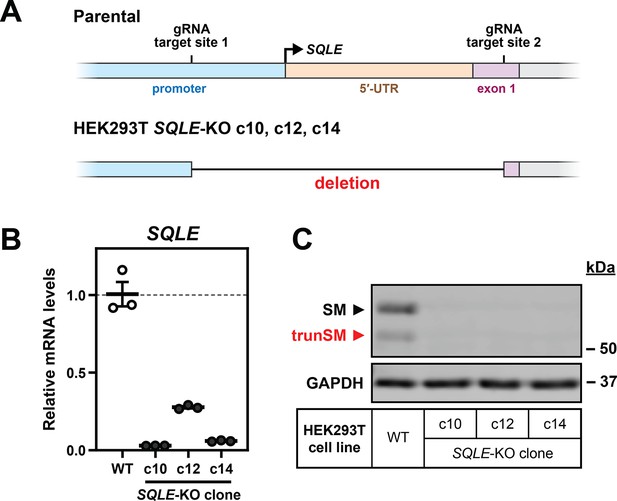
Generation of SQLE-knockout HEK293T cells.
(A) HEK293T cells were transfected with CRISPR/Cas9 guide RNAs (gRNAs) targeting the proximal promoter and first exon of the SQLE gene, and three clonal populations of SQLE-KO cells were isolated and expanded for each parental cell line. The target region was amplified from genomic DNA and sequenced, which found that all three clones contain a genomic deletion between the gRNA target sites. (B) SQLE transcript levels were quantified in HEK293T SQLE-KO clones, normalized to the PBGD housekeeping transcript, and adjusted relative to the parental (WT) cell line, which was set to 1 (dotted line). Data presented as mean ± SEM for technical triplicates in a single experiment. (C) Protein lysates from the HEK293T WT cell line and SQLE-KO clones were immunoblotted for SM and trunSM.
-
Figure 4—figure supplement 3—source data 1
Uncropped immunoblots for Figure 4—figure supplement 3.
- https://cdn.elifesciences.org/articles/82843/elife-82843-fig4-figsupp3-data1-v1.zip
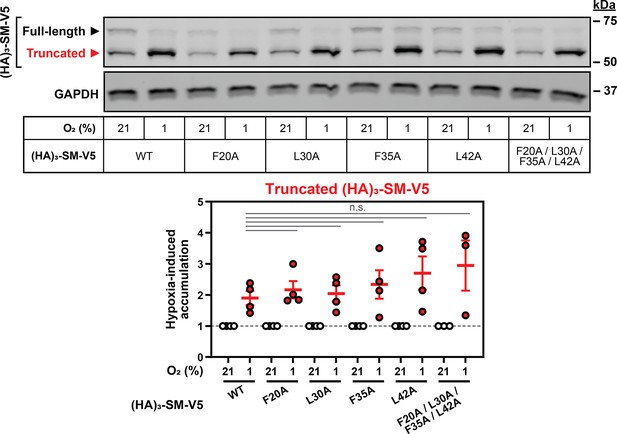
Putative squalene-binding residues are not required for hypoxia-induced partial degradation of SM.
HEK293T cells were transfected with the indicated constructs for 24 hr and incubated under normoxic or hypoxic conditions for 16 hr. Graph depicts densitometric quantification of protein levels normalized to the respective normoxic conditions for each construct, which were set to 1 (dotted line). Data presented as mean ± SEM from n=3–4 independent experiments (n.s., not significant; two-tailed ratio paired t-test).
-
Figure 4—figure supplement 4—source data 1
Uncropped immunoblots for Figure 4—figure supplement 4.
- https://cdn.elifesciences.org/articles/82843/elife-82843-fig4-figsupp4-data1-v1.zip
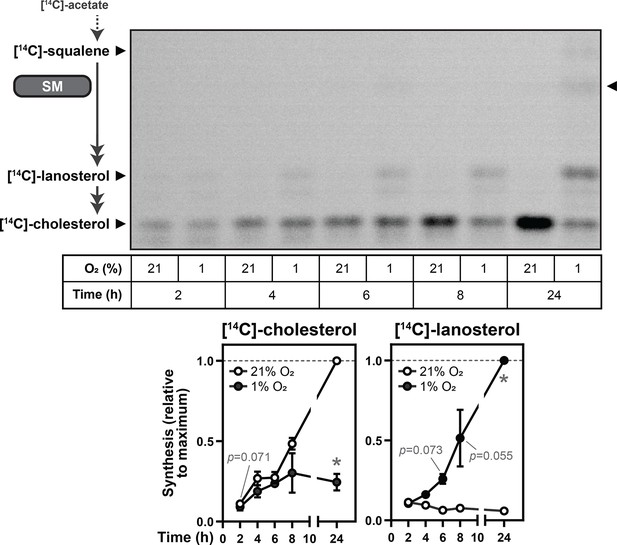
SM activity is preserved during hypoxia.
Huh7 cells were labelled with 2 µCi [14C]-acetate and incubated under normoxic or hypoxic conditions for the indicated times. Synthesis of [14C]-squalene, [14C]-lanosterol, and [14C]-cholesterol was determined using thin-layer chromatography. Double-headed arrows indicate multiple enzymatic steps, and arrowhead on right of image indicates band corresponding to the SM product [14C]-monooxidosqualene (Capell-Hattam et al., 2022). Graphs depict densitometric quantification of [14C]-cholesterol and [14C]-lanosterol levels normalized to the conditions with the greatest abundance of each analyte, which were set to 1 (dotted line). Data presented as mean ± SEM from n=3 independent experiments (*, p≤0.05; two-tailed ratio paired t-test vs. respective normoxic condition for each timepoint).
-
Figure 5—source data 1
Uncropped thin-layer chromatography image for Figure 5.
- https://cdn.elifesciences.org/articles/82843/elife-82843-fig5-data1-v1.zip
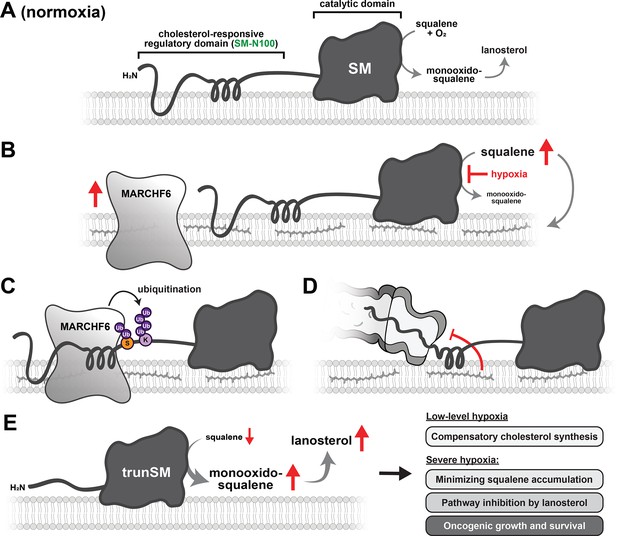
Model of hypoxia-induced SM truncation.
(A, B) Hypoxic conditions stabilize the E3 ubiquitin ligase MARCHF6 and inhibit SM-catalyzed conversion of squalene to monooxidosqualene, leading to squalene accumulation. (C) Increased MARCHF6 activity promotes ubiquitination of SM and its targeting to the proteasome. (D) Squalene impedes the complete proteasomal degradation of SM via a mechanism involving the SM-N100 domain, (E) yielding the constitutively active trunSM. During transient or low-level hypoxia, trunSM activity may facilitate continued cholesterol synthesis to compensate for the oxygen shortfall. During long-term or severe hypoxia, trunSM activity may reduce squalene-induced toxicity and promote downstream synthesis of lanosterol, which suppresses an early step of the cholesterol synthesis pathway. During pathophysiological hypoxia, cholesterol synthesis enabled by trunSM may contribute to oncogenic cell growth and survival.
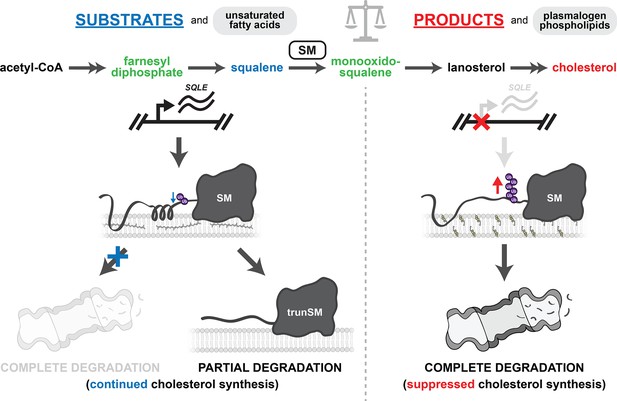
Summary of metabolic regulation of SM.
Squalene (blue), other farnesyl-containing cholesterol synthesis intermediates (green), and unsaturated fatty acids stabilize SM by impeding its ubiquitination and complete proteasomal degradation. When SM reaches the proteasome, squalene and farnesyl-containing intermediates can also promote its partial degradation to yield the constitutively active trunSM. In both cases, SM activity is preserved and continued cholesterol synthesis is favored. Excess cholesterol suppresses SQLE gene expression and accelerates the ubiquitination and complete proteasomal degradation of SM. Plasmalogen phospholipids also promote SM ubiquitination and degradation. This suppresses SM activity and downstream cholesterol synthesis. Balance of these feedforward and feedback loops ensures cholesterol synthesis is tightly coupled with supply and demand.
Tables
Reagent type (species) or resource | Designation | Source or reference | Identifiers | Additional information |
---|---|---|---|---|
Gene (Homo sapiens) | SQLE | RefSeq | 6713, NM_003129.4 | |
Cell line (H. sapiens) | HEK293T | Gift from UNSW School of Medical Sciences (UNSW Sydney, Australia) | Highly transfectable human embryonic kidney cells | |
Cell line (H. sapiens) | HCT116 | Gift from Dr Ewa Goldys (UNSW Sydney, Australia) | Epithelial colorectal carcinoma cells | |
Cell line (H. sapiens) | Huh7 | Gift from Centre for Cardiovascular Research (UNSW Sydney, Australia) | Epithelial-like hepatocellular carcinoma cells | |
Cell line (H. sapiens) | HeLaT | Gift from Drs Louise Lutze- Mann and Noel Whitaker (UNSW Sydney, Australia) | Highly transfectable cervical adenocarcinoma cells | |
Cell line (H. sapiens) | MDA-MB-231 | Gift from Drs Louise Lutze-Mann and Noel Whitaker (UNSW Sydney, Australia) | Epithelial breast adenocarcinoma cells | |
Cell line (H. sapiens) | HEK SM-N100-GFP-V5 | Generated previously using the Flp-In T-REx system (Zelcer et al., 2014) | HEK293 cells stably expressing the N-terminal 100 amino acids of SM (SM-N100) fused with green fluorescent protein and a V5 epitope tag. Controlled by a cytomegalovirus (CMV) promoter. | |
Cell line (H. sapiens) | HEK MARCHF6-V5 | Generated previously using the Flp-In T-REx system (Sharpe et al., 2019) | HEK293 cells stably expressing MARCHF6 fused with a V5 epitope tag. Controlled by a CMV promoter. | |
Cell line (H. sapiens) | HEK293T SQLE-knockout clone 10 (c10) | This study | See Materials and Methods, Generation of SM knockout cells | |
Cell line (H. sapiens) | HEK293T SQLE-knockout clone 12 (c12) | This study | See Materials and Methods, Generation of SM knockout cells | |
Cell line (H. sapiens) | HEK293T SQLE-knockout clone 14 (c14) | This study | See Materials and Methods, Generation of SM knockout cells | |
Transfected construct (H. sapiens) | MISSION universal negative control #1 siRNA | Sigma-Aldrich | SIC001 | |
Transfected construct (H. sapiens) | MARCHF6 siRNA | Sigma-Aldrich | SASI_Hs01_00105239 | |
Transfected construct (H. sapiens) | HIF1A siRNA | Sigma-Aldrich | SASI_Hs01_00332063 | |
Transfected construct (H. sapiens) | EPAS1 (HIF2A) siRNA | Sigma-Aldrich | SASI_Hs01_00019152 | |
Antibody | Anti-SM(SQLE) (rabbit polyclonal) | Proteintech | 12544-1-AP | 4 °C for 16 hr (1:2500) |
Antibody | Anti-HIF1α (rabbit polyclonal) | Proteintech | 20960-1-AP | Room temperature for 1 hr (1:1000) |
Antibody | Anti-GAPDH (rabbit monoclonal) | Cell Signaling Technology | 2118 | 4 °C for 16 hr (1:2000) |
Antibody | Anti-V5 (mouse monoclonal) | Thermo Fisher Scientific | R960-25 | Room temperature for 1 hr (1:5000) |
Antibody | IRDye 680RD anti- rabbit IgG (donkey polyclonal) | LI-COR Biosciences | LCR-926-68073 | Room temperature for 1 hr (1:5000) |
Antibody | IRDye 800CW anti- mouse IgG (donkey polyclonal) | LI-COR Biosciences | LCR-926-32212 | Room temperature for 1 hr (1:10,000) |
Antibody | Peroxidase-conjugated AffiniPure anti-rabbit IgG (donkey polyclonal) | Jackson ImmunoResearch Laboratories | 711-035-152 | Room temperature for 1 hr (1:10,000) |
Antibody | Peroxidase-conjugated AffiniPure anti-mouse IgG (donkey polyclonal) | Jackson ImmunoResearch Laboratories | 715-035-150 | Room temperature for 1 hr (1:10,000) |
Commercial assay or kit | Lipofectamine RNAiMAX transfection reagent | Thermo Fisher Scientific | 13778150 | |
Commercial assay or kit | Lipofectamine 3000 transfection reagent | Thermo Fisher Scientific | L3000001 | |
Commercial assay or kit | Bicinchoninic acid assay kit | Thermo Fisher Scientific | 23225 | |
Commercial assay or kit | TRI reagent | Thermo Fisher Scientific | AM9738 | |
Commercial assay or kit | SuperScript III First- Strand Synthesis kit | Thermo Fisher Scientific | 18080051 | |
Commercial assay or kit | PureLink Genomic DNA Mini kit | Thermo Fisher Scientific | K182001 | |
Commercial assay or kit | QuantiNova SYBR Green PCR kit | Qiagen | 208052 | |
Commercial assay or kit | Immobilon Western chemiluminescent HRP substrate | Millipore | WBKLS0500 | |
Chemical compound, drug | [14C]-Acetic acid sodium salt | PerkinElmer | NEC084H001MC | |
Chemical compound, drug | 2,3,22,23-Dioxidosqualene | Echelon Biosciences | S-0302 | |
Chemical compound, drug | 2,3-Oxidosqualene (mono-oxidosqualene) | Echelon Biosciences | S-0301 | |
Chemical compound, drug | 5α-Cholestane | Sigma-Aldrich | C8003 | |
Chemical compound, drug | Bafilomycin A1 | Sigma-Aldrich | B1793 | |
Chemical compound, drug | BIBB 515 | Cayman Chemical Company | 10010517 | |
Chemical compound, drug | DMOG | Sigma-Aldrich | D3695 | |
Chemical compound, drug | FG-4592 | Cayman Chemical Company | 15294 | |
Chemical compound, drug | GR70585X | GlaxoSmithKlein | N/A | |
Chemical compound, drug | Mevalonolactone | Sigma-Aldrich | M4667 | |
Chemical compound, drug | Mevastatin | Sigma-Aldrich | M2537 | |
Chemical compound, drug | MG132 | Sigma-Aldrich | C2211 | |
Chemical compound, drug | NB-598 | Chemscene | CS-1274 | |
Chemical compound, drug | Squalane | Sigma-Aldrich | 234311 | |
Chemical compound, drug | Squalene | Sigma-Aldrich | S3626 | |
Chemical compound, drug | TAK-475 | Sigma-Aldrich | SML2168 | |
Chemical compound, drug | N,O-Bis-(trimethylsilyl) trifluoroacetamide | Supelco | T6381 | |
Software, algorithm | Thermo Xcalibur software | Thermo Fisher Scientific | v2.2 SP1.48 | |
Software, algorithm | Image Studio Lite software | LI-COR Biosciences | v5.2.5 | |
Software, algorithm | GraphPad Prism software | GraphPad Software Inc | v9.0 | |
Other | Opti-MEM I reduced serum medium | Thermo Fisher Scientific | 31985062 | Used to deliver transfection complexes. See Materials and Methods, siRNA and Plasmid Transfection |
Plasmids used for transfection.
Plasmid | Description |
---|---|
pCMV-(HA)3-SM-V5 | pcDNA3.1/V5-His TOPO vector containing the coding sequence of human squalene monooxygenase (SM; NM_003129.4) fused with three N-terminal HA epitope tags and C-terminal V5 and 6×His epitope tags, under the transcriptional control of a constitutive cytomegalovirus (CMV) promoter. Generated previously by the Brown laboratory. |
pCMV-(HA)3-SM-V5 K82 / 90 / 100R | pCMV-(HA)3-SM-V5 containing SM K82R, K90R and K100R substitutions, which blunt SM truncation (Coates et al., 2021). Generated previously (Coates et al., 2021). |
pCMV-(HA)3-SM-V5 K290R | pCMV-(HA)3-SM-V5 containing a K290R substitution, which disrupts a known ubiquitination site (Hornbeck et al., 2015). Generated in this study. |
pCMV-(HA)3-SM-V5 SM-N100 C/S/T>A | pCMV-(HA)3-SM-V5 containing SM T3A, T9A, T11A, S43A, C46A, S59A, S61A, S67A, S71A, S83A and S87A substitutions, which blunt cholesterol-induced degradation of SM (Chua et al., 2019). Generated previously (Coates et al., 2021). |
pCMV-(HA)3-SM-V5 Y195F | pCMV-(HA)3-SM-V5 containing an SM Y195F substitution, which causes loss of catalytic activity (Padyana et al., 2019). Generated previously (Coates et al., 2021). |
pCMV-SM(ΔN65)-V5 | pCMV-(HA)3-SM-V5 containing a deletion of the N-terminal (HA)3 tag and first 65 residues of SM, which are lost upon truncation (Coates et al., 2021). Generated previously (Coates et al., 2021). |
pCMV-SM(ΔN65)-V5 Y195F | pCMV-SM(ΔN65)-V5 containing an SM Y195F substitution, which causes loss of catalytic activity (Padyana et al., 2019). Generated in this study. |
pCMV-(HA)3-SM-V5 F20A | pCMV-(HA)3-SM-V5 containing a F20A substitution within the N-terminal re-entrant loop (Howe et al., 2015). Generated in this study. |
pCMV-(HA)3-SM-V5 L30A | pCMV-(HA)3-SM-V5 containing a L30A substitution within the N-terminal re-entrant loop (Howe et al., 2015). Generated in this study. |
pCMV-(HA)3-SM-V5 F35A | pCMV-(HA)3-SM-V5 containing a F35A substitution within the N-terminal re-entrant loop (Howe et al., 2015). Generated in this study. |
pCMV-(HA)3-SM-V5 L42A | pCMV-(HA)3-SM-V5 containing a L42A substitution within the N-terminal re-entrant loop (Howe et al., 2015). Generated in this study. |
pCMV-(HA)3-SM-V5 F20A / L30A / F35A / L42A | pCMV-(HA)3-SM-V5 containing F20A, L30A, F35A and L42A substitutions within the N-terminal re-entrant loop (Howe et al., 2015). Generated in this study. |
PX458 SQLE-1 (promoter) | PX458 containing a guide RNA sequence targeting the SQLE proximal promoter (hg38 chr8:124998048–124998067). Generated in this study. |
PX458 SQLE-2 (exon 1) | PX458 containing a guide RNA sequence targeting SQLE exon 1 (hg38 chr8:124999588–124999607). Generated in this study. |
Primers used for qRT-PCR and DNA cloning.
Non-annealing nucleotides for restriction enzyme cloning and DNA substitution are indicated in lowercase.
qRT-PCR primer pair | Primer sequence (5′‒3′) | Source | |
---|---|---|---|
VEGF | Forward Reverse | CCTGGTGGACATCTTCCAGG CTGTAGGAAGCTCATCTCTCC | Coates et al., 2019 |
CA9 | Forward Reverse | GTGCCTATGAGCAGTTGCTGTC AAGTAGCGGCTGAAGTCAGAGG | Origene |
RPL11 | Forward Reverse | AGAGTGGAGACAGACTGACGCG CGGATGCCAAAGGATCTGACAG | Origene |
GAPDH | Forward Reverse | GTCTCCTCTGACTTCAACAGCG ACCACCCTGTTGCTGTAGCCAA | Origene |
ACTB | Forward Reverse | CACCATTGGCAATGAGCGGTTC AGGTCTTTGCGGATGTCCACGT | Origene |
HIF1A | Forward Reverse | CAGTAACCAACCTCAGTGTGG CAGATGATCAGAGTCCAAAGC | Dr Laura Sharpe (Brown laboratory) |
EPAS1 (HIF2A) | Forward Reverse | CTGTGTCTGAGAAGAGTAACTTCC TTGCCATAGGCTGAGGACTCCT | Origene |
SQLE | Forward Reverse | GCTTCCTTCCTCCTTCATCAGTG GCAACAGTCATTCCTCCACCA | Coates et al., 2021 |
HMGCR | Forward Reverse | TTGGTGATGGGAGCTTGCTGTG AGTCACAAGCACGTGGAAGACG | Wong et al., 2007 |
DNA cloning primer pair | Primer sequence (5′‒3′) | Method | |
SQLE gRNA 1 (promoter) | Forward Reverse | caccgAATGGAAACGTTCCGACCCG aaacCGGGTCGGAACGTTTCCATTc | BbsI cloning into PX598 vector (as in Ran et al., 2013) |
SQLE gRNA 2 (exon 1) | Forward Reverse | aaacAGTTCGCCCTCTTCTCGGATc caccgATCCGAGAAGAGGGCGAACT | |
SM K290R | Forward Reverse | GATGGGCTTTTCTCCAgGTTCAGGAAAAGCCTG GCGATGCAATTTCCTCATTT | Overlap extension cloning (as in Stevenson et al., 2013) |
SM Y195F | Forward Reverse | CCAGGTTGTAAATGGTTtCATGATTCATGATCAGG GCGATGCAATTTCCTCATTT | |
SM F20A | Forward Reverse | GTTCGGGGACgcCATCACTTTGG GCGATGCAATTTCCTCATTT | |
SM L30A | Forward Reverse | GGAGGTCCTGgcGTGCGTGCTGGTG GCGATGCAATTTCCTCATTT | |
SM F35A | Forward Reverse | GTGCTGGTGgcCCTCTCGCTG GCGATGCAATTTCCTCATTT | |
SM L42A | Forward Reverse | CTGGGCCTGGTGgcCTCCTACCGCTG GCGATGCAATTTCCTCATTT | |
SM F20A / L30A / F35A / L42A | Forward Reverse | Combination of above mutagenic primers GCGATGCAATTTCCTCATTT |