Structures of ferroportin in complex with its specific inhibitor vamifeport
Figures
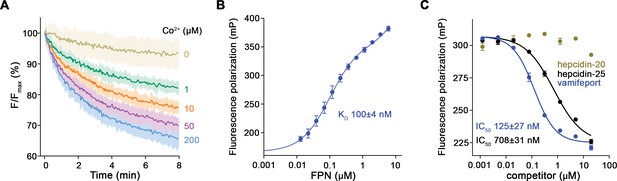
Functional characterization of hsFPN.
(A) Fluorescence-based uptake of Co2+ into proteoliposomes containing reconstituted hsFPN. Metal ion influx is monitored with the fluorophore calcein that is trapped inside the liposomes (six measurements for 0 μM Co2+, 1 μM Co2+, and 10 μM Co2+ and eight measurements for 50 μM Co2+ and 200 μM Co2+ from four independent reconstitutions). (B) Binding of fluorescently labeled hepcidin-25 (TMR-hepcidin) to increasing concentrations of hsFPN as monitored by the change in the fluorescence polarization of the peptide (six measurements from two independent experiments). (C) Competition of bound TMR-hepcidin with vamifeport and unlabeled hepcidin-20 and hepcidin-25 (six measurements for vamifeport and hepcidin-25 from three independent experiments and four measurements for hepcidin-20 from two independent experiments). (A–C) Data show mean of the indicated number of measurements, and errors are SEM.
-
Figure 1—source data 1
Proteoliposome-based in-vitro transport data of hsFPN, TMR-hepcidin fluorescence polarization direct binding and displacement data of hsFPN.
- https://cdn.elifesciences.org/articles/83053/elife-83053-fig1-data1-v1.zip
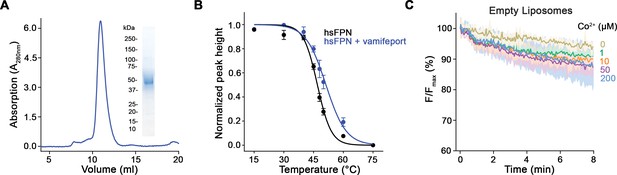
Biochemical properties of purified hsFPN.
(A) Size-exclusion chromatography (SEC) profile of the purified protein on a Superdex 200 column. Inset (right) shows SDS-PAGE of peak fractions with indicated molecular weight of marker proteins (kDa). (B) Thermal stability of hsFPN in absence and presence of vamifeport determined by fluorescence-detection SEC (4–5 measurements from four independent experiments in absence of vamifeport and three measurements from two independent experiments in presence of vamifeport). (C) Unspecific leak of Co2+ into empty proteoliposomes (three measurements from three independent reconstitutions). (B and C) Data show mean of the indicated number of experiments, and errors are SEM.
-
Figure 1—figure supplement 1—source data 1
Size-exclusion chromatography data of hsFPN and corresponding SDS-PAGE gels, thermal stability data, and proteoliposome-based in-vitro transport data of empty liposomes.
- https://cdn.elifesciences.org/articles/83053/elife-83053-fig1-figsupp1-data1-v1.zip
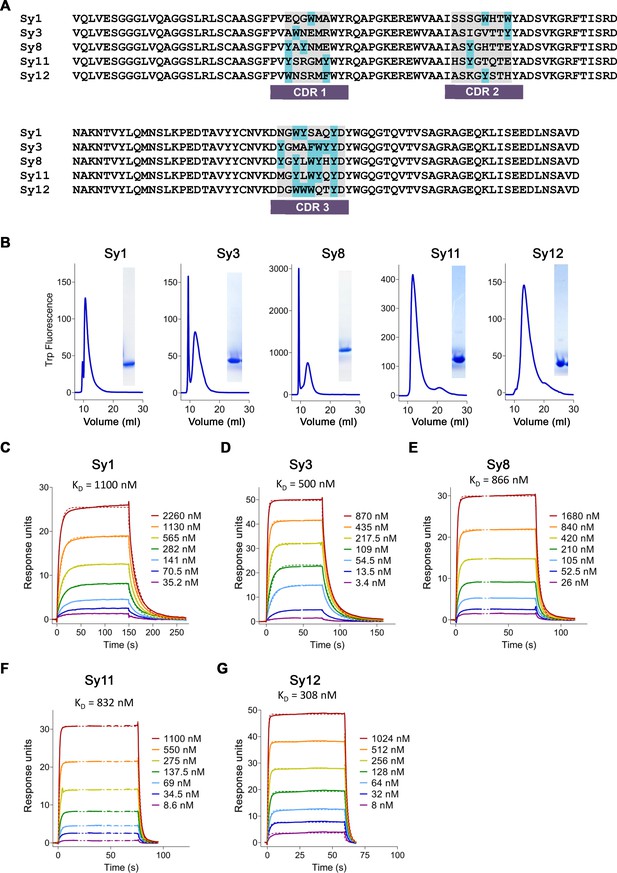
Characterization of sybodies binding hsFPN.
(A) Alignment of sybody sequences. Complementary determining regions (CDRs) are indicated, and aromatic residues are labeled in light blue. (B) Size-exclusion chromatography (SEC) profiles of the purified sybodies on an SRT-100 column and SDS-PAGE of peak fractions (right). (C–G) Affinity determination by surface plasmon resonance (SPR) experiments using immobilized hsFPN and varying concentrations of Sy1 (C), Sy3 (D), Sy8 (E), Sy11 (F), and Sy12 (G). Individual traces of the association and dissociation of sybodies and dashed lines representing the fit to a 1:1 binding model are shown in distinct colors. KD values are indicated.
-
Figure 1—figure supplement 2—source data 1
Size-exclusion chromatography data of all sybodies and corresponding SDS-PAGE gels, surface plasmon resonance (SPR) binding data of all sybodies.
- https://cdn.elifesciences.org/articles/83053/elife-83053-fig1-figsupp2-data1-v1.zip
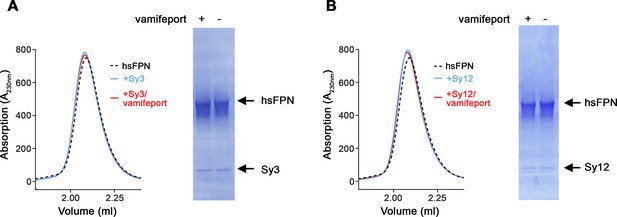
Biochemical properties of sybody-hsFPN complexes.
Size-exclusion chromatography (SEC) profiles of hsFPN in complex with Sy3 (A) and Sy12 (B). A sample of hsFPN was incubated with five times molar excess of the respective sybody in the absence and presence of a large excess of vamifeport (1 mM) and analyzed on a Superdex 200 column. For comparison, the SEC profile of hsFPN without binders is displayed as a dashed black line. The peak fractions were analyzed on SDS-PAGE (right) to detect the individual components (hsFPN and the respective sybodies).
-
Figure 1—figure supplement 3—source data 1
Size-exclusion chromatography data of sybody-hsFPN complexes and corresponding SDS-PAGE gels.
- https://cdn.elifesciences.org/articles/83053/elife-83053-fig1-figsupp3-data1-v1.zip
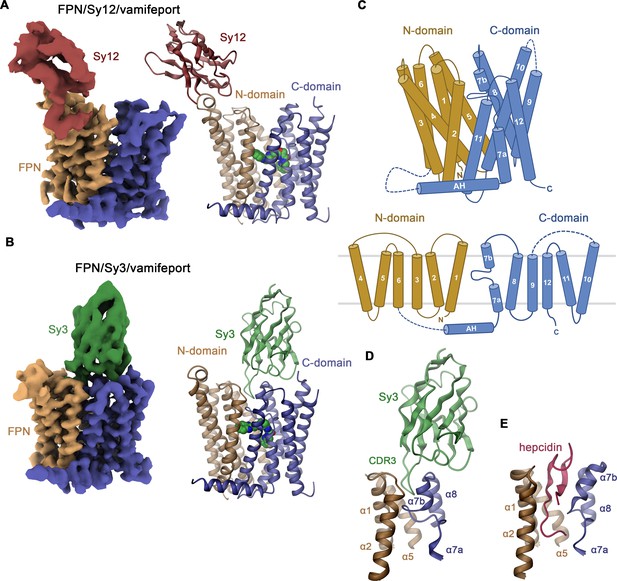
Structure of hsFPN/sybody complexes.
Cryo-electron microscopy (cryo-EM) density (left) and ribbon model (right) of (A), the hsFPN/Sy12/vamifeport and (B), the hsFPN/Sy3/vamifeport complex. N- and C-domains of hsFPN and the respective sybodies are labeled and shown in unique colors. Bound vamifeport is displayed in space-filling representation. (C) Schematic depiction of membrane-spanning helices (top) and topology of hsFPN (bottom). Ribbon representation of (D), the binding region of Sy3 and (E), hepcidin (obtained from PDBID: 6WBV). Secondary structure elements are labeled.
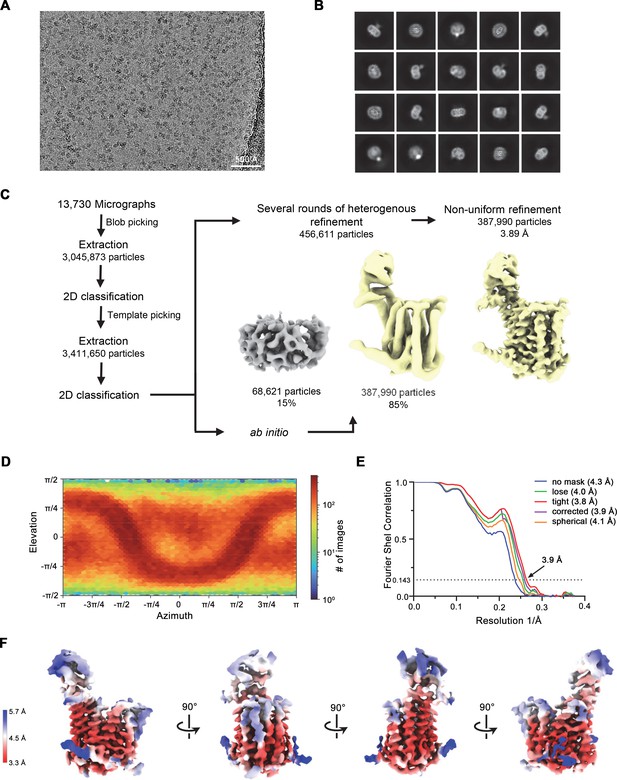
Cryo-electron microscopy (cryo-EM) reconstruction of the hsFPN/Sy12/vamifeport complex.
(A) Representative micrograph (of a total of 13,730) of the hsFPN/Sy12/vamifeport dataset. (B) 2D class averages of the hsFPN/Sy12/vamifeport complex. (C) Data-processing workflow. Particles were extracted and subjected to 2D classification, and generated 2D class averages were subsequently used as templates for particle picking. After extraction, the novel set of particles was subjected to a second round of 2D classification. Based on 2D class averages, particles were selected for two ab initio reconstructions. A generated model displaying protein features (yellow) and a ‘decoy’ model lacking such features (gray) were both used for several rounds of heterogenous refinement followed by non-uniform refinement yielding a map at 3.9 Å resolution. (D) Heatmap displaying the angular distribution of particle orientations. (E) FSC plot of the final refined spherical map (orange), corrected mask (purple), tight mask (red), loose mask (green), and unmasked (blue) cryo-EM density map of the hsFPN/Sy12/vamifeport complex. The dotted line indicates the resolution at which the FSC drops below the 0.143 threshold. (F) The final 3D reconstruction in indicated orientations colored according to the local resolution.
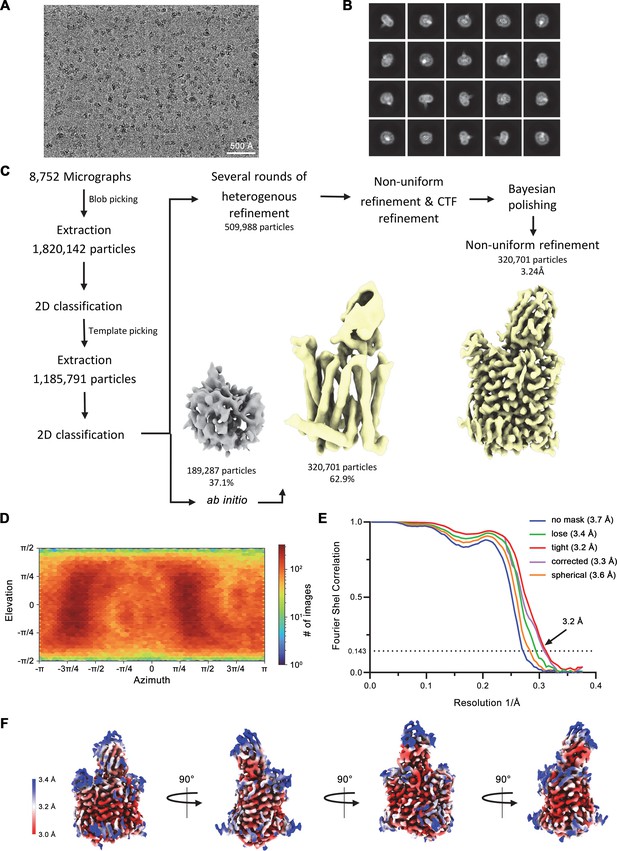
Cryo-electron microscopy (cryo-EM) reconstruction of the hsFPN/Sy3/vamifeport complex.
(A) Representative micrograph (of a total of 8752) of the hsFPN/Sy3/vamifeport dataset. (B) 2D class averages of the hsFPN/Sy3/vamifeport complex. (C) Data-processing workflow. Particles were extracted and subjected to 2D classification, and the obtained 2D class averages were subsequently used as templates for particle picking. After extraction, the novel set of particles was subjected to a second 2D classification round. Based on 2D class averages, particles were selected for two ab initio reconstructions. A generated model displaying protein features (yellow) and a ‘decoy’ model lacking such features (gray) were both used for several rounds of heterogenous refinement followed by non-uniform refinement yielding a map at 3.4 Å resolution. The particles were imported into RELION for Bayesian polishing. The polished particles were imported back to cryoSPARC for a final round of non-uniform refinement resulting in a map at 3.2 Å resolution. (D) Heatmap displaying the angular distribution of particle orientations. (E) FSC plot of the final refined spherical map (orange), corrected mask (purple), tight mask (red), loose mask (green), and unmasked (blue) cryo-EM density map of the hsFPN/Sy3/vamifeport complex. The dotted line indicates the resolution at which the FSC drops below the 0.143 threshold. (F) The final 3D reconstruction at indicated orientations is colored according to the local resolution.
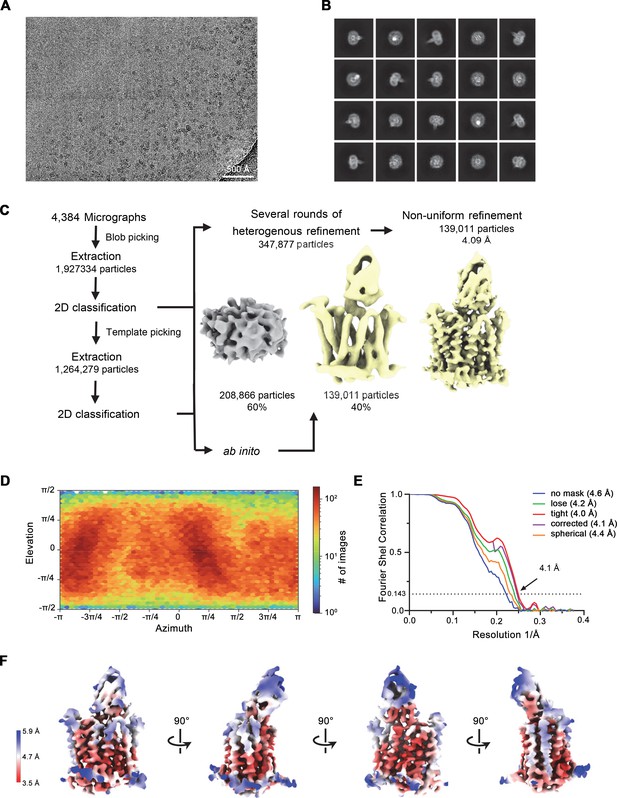
Cryo-electron microscopy (cryo-EM) reconstruction of the hsFPN/Sy3 complex.
(A) Representative micrograph (of a total of 4384) of the hsFPN/Sy3 dataset. (B) 2D class averages of the hsFPN/Sy3 complex. (C) Data-processing workflow. Particles were extracted and subjected to 2D classification, and the obtained 2D class averages were subsequently used as templates for particle picking. After extraction, the new set of particles was subjected to a second round of 2D classification. Based on 2D class averages, particles were selected for two ab initio reconstructions. A generated model displaying protein features (yellow) and a ‘decoy’ model lacking such features (gray) were both used for several rounds of heterogenous refinement, followed by non-uniform refinement yielding a map at 4.1 Å resolution. (D) Heatmap displaying the angular distribution of particle orientations. (E) FSC plot of the final refined spherical map (orange), corrected mask (purple), tight mask (red), loose mask (green), and unmasked (blue) cryo-EM density map of the hsFPN/Sy3 complex. The dotted line indicates the resolution at which the FSC drops below the 0.143 threshold. (F) The final 3D reconstruction at indicated orientations is colored according to the local resolution.
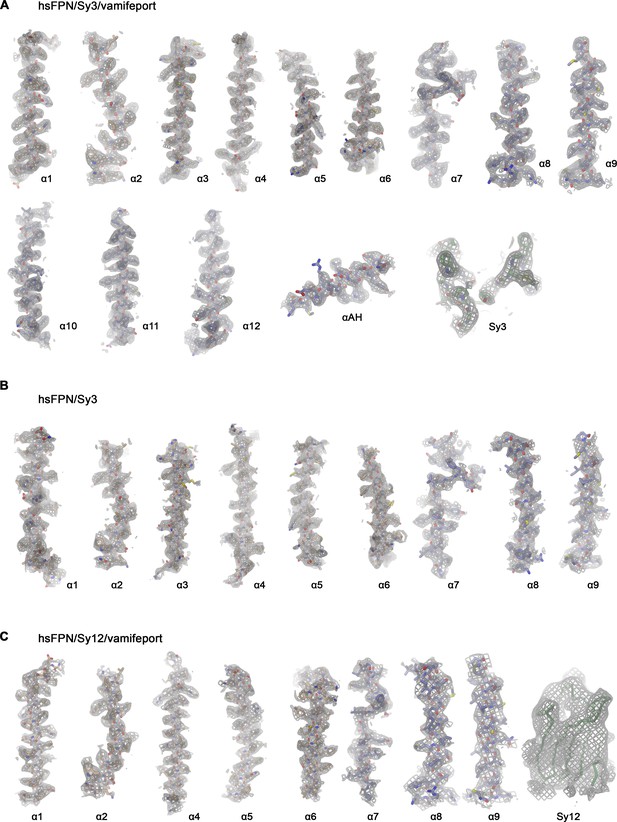
Cryo-electron microscopy (cryo-EM) density of hsFPN/sybody complexes.
Cryo-EM density of specified hsFPN complexes superimposed on selected regions of the protein with secondary structure elements indicated. (A) hsFPN/Sy3/vamifeport complex at 3.2 Å. Sharpened density (b=−100, contoured at 6.5 σ) is superimposed on the model. ‘Sy3’ refers to the binding epitope of Sy3. (B) hsFPN/Sy3 complex at 4.1 Å. Sharpened density (b=−50, contoured at 6.0 σ) is superimposed on the model. (C) hsFPN/Sy12/vamifeport complex at 3.9 Å. Sharpened density (b=−30, contoured at 5 σ) is superimposed on the model. ‘Sy12’ displays the envelope of the blurred electron density (b=50, contoured at 5 σ), which defines the orientation of Sy12, superimposed on a Cα-trace of the sybody.
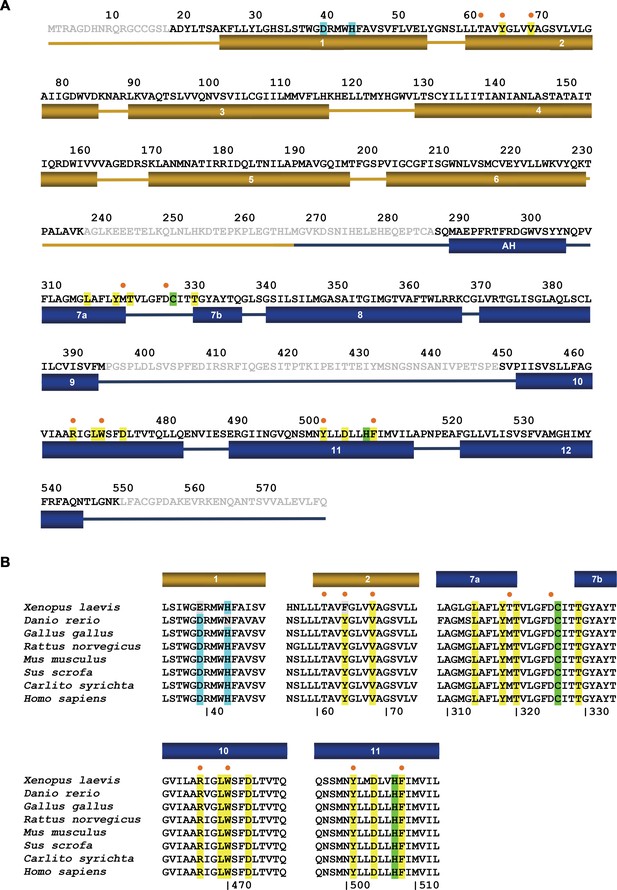
FPN sequence.
(A) Amino acid sequence of hsFPN (GenBank: AAF36697.1). Secondary structure elements are indicated below. Residues of the metal binding site S1 are highlighted in cyan, residues of the metal binding site S2, which also interact with vamifeport, in green. Additional residues that contact vamifeport are highlighted in yellow. (B) Superposition of the vamifeport interacting region of selected FPN sequences (H. sapiens, GenBank: AAF36697.1; X. laevis, GenBank: XP_018122848.2; D. rerio: Q9I9R3.2; G. gallus, GenBank: NP_001012931.1; R. norvegicus, GenBank: AAK77858.2; M. musculus, GenBank: XP_006496200.1; S. scrofa, GenBank: XP_003483749.1; C. syrichita, GenBank: XP_008060857.1). Color coding of highlighted residues is as in A. (A and B) Residues in contact with vamifeport that also participate in the interaction with hepcidin are labeled (●).
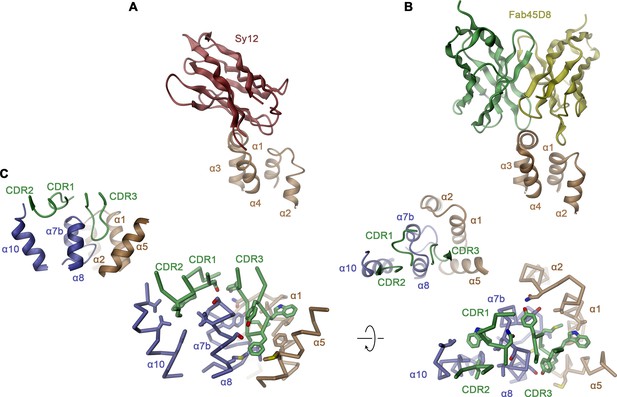
hsFPN/sybody interactions.
Interaction regions of (A) Sy12 and (B) the Fv-part of Fab45D8 (PDBID 6WBV) on the outward-facing conformations of hsFPN. (C) Binding interface between Sy3 and hsFPN as defined in the hsFPN/Sy3/vamifeport complex. Shown are two orientations parallel to the membrane (left) and from the extracellular side (right). The protein is shown as Cα-trace with interacting side chains displayed as sticks. Insets (top left) show the corresponding views of the same interaction site as ribbon representation. (A–C) Secondary structure elements are indicated.
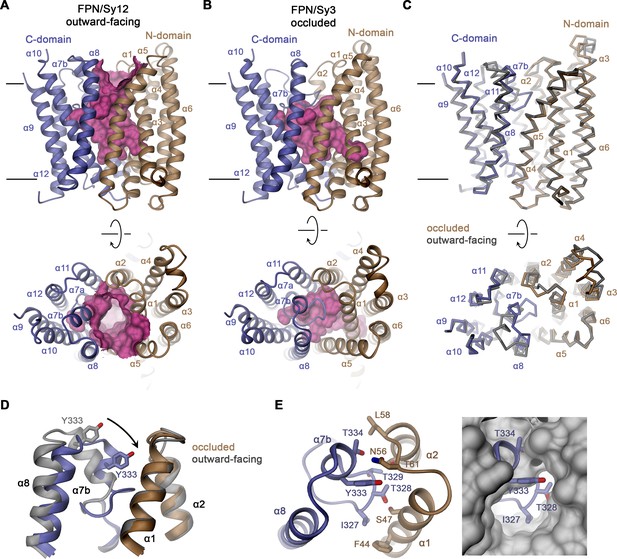
Features of hsFPN conformations.
(A) Outward-facing conformation of hsFPN as observed in the hsFPN/Sy12/vamifeport complex. (B) Occluded conformation of hsFPN as observed in the hsFPN/Sy3/vamifeport complex. (A and B) The molecular surface of the outward-facing and occluded cavities harboring the substrate binding sites is shown in magenta. (C) Cα representation of a superposition of both conformations of hsFPN. (A–C) Views are from within the membrane (top) and from the outside (bottom). (D) Comparison of both conformations in the region showing the largest differences. (E) Interaction between α7b and α2 and α3 closing the extracellular access to the substrate binding site (left). To further illustrate the occlusion by residues of the α7a–α7b region, the occluded model is superimposed on a surface representation of the outward-facing structure (right). (C and D) The outward-facing structure is colored in gray for comparison. (A–E) Secondary structure elements are labeled. The coloring is as in Figure 1A.
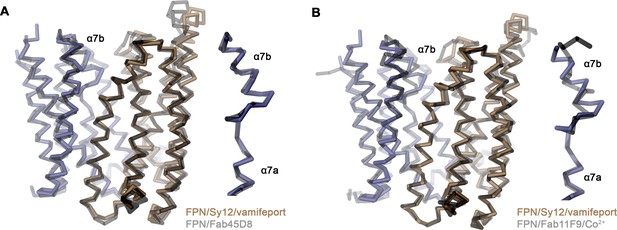
Superposition of outward-facing conformations.
Cα traces of the outward-facing conformation of hsFPN in the hsFPN/Sy12/vamifeport complex compared to the known structures of the (A), hsFPN/Fab45D8 complex (PDBID 6W4S) and (B), the tsFPN/Fab11F9/Co2+ complex (PDBID 6VYH). (A and B) The two sub-domains of hsFPN are colored as in Figure 2, and previously determined structures are shown in gray. Inset (right) shows blow-up of regions connecting helices α7a and α7b.
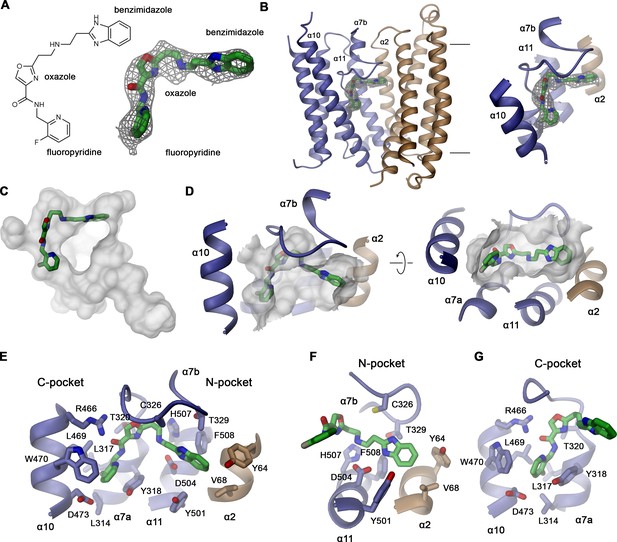
Structural basis of vamifeport binding.
(A) Chemical structure of vamifeport and its fit into the corresponding cryo-electron microscopy (cryo-EM) density of the hsFPN/Sy3/vamifeport complex. (B) Ribbon representation of the occluded conformation of FPN observed in the hsFPN/Sy3/vamifeport complex with the cryo-EM density attributed to vamifeport shown to illustrate its location within the transporter. Inset (right) shows blow-up of the binding region. (A and B) Cryo-EM density is contoured at 6.5 σ. (C) Location of vamifeport within the occluded cavity of hsFPN and (D), blowup of the binding pocket. (D and E) The molecular surface of the cavity is shown in gray. (E) Protein inhibitor interactions in the vamifeport binding site and interactions in (F) the N-pocket and (G) the C-pocket. (E–G) Side chains of interacting residues are shown as sticks and labeled.
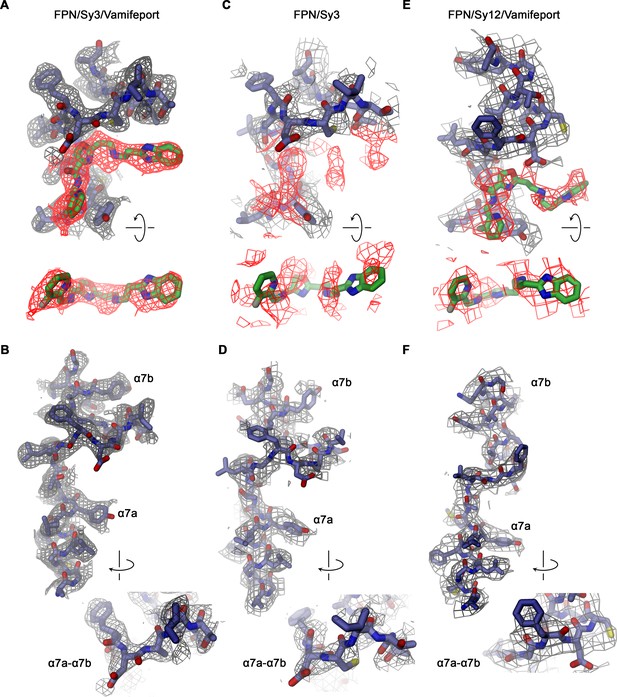
Density in the vamifeport binding site.
Sections of the cryo-electron microscopy (cryo-EM) densities surrounding the vamifeport binding site. (A) Vamifeport interactions in the hsFPN/Sy3/vamifeport complex and (B) density around α-helix 7 of the same complex (cryo-EM density is contoured at 6.5 σ). (C) The equivalent region in the hsFPN/Sy3 complex not containing vamifeport does not show any comparable density. (D) Density around α-helix 7 of the same complex (cryo-EM density is contoured at 6.0 σ). (E) Density in the binding region of the hsFPN/Sy12/vamifeport complex indicates vamifeport binding to the equivalent region in the outward-facing conformation that is less well defined. (F) Density around α-helix 7 of the same complex (cryo-EM density is contoured at 6.0 σ). (A, C, and E) Top views show cryo-EM density of the binding region (displayed in gray) with density surrounding the location of vamifeport in the hsFPN/Sy3/vamifeport complex (A and C) and the hsFPN/Sy12/vamifeport complex (E) colored in red. Bottom shows a view of the density surrounding the vamifeport location from the cytoplasm. In C, vamifeport obtained from the hsFPN/Sy3/vamifeport complex is shown as reference. (B, D, and F) Insets show blow-up of the loop connecting α7a and α7b in indicated orientation.
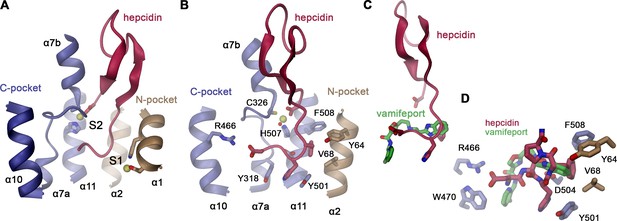
Hepcidin interactions.
(A) Location of the hepcidin binding site in hsFPN (obtained from PDBID 6WBV) relative to the metal ion binding sites S1 and S2. (B) Details of hsFPN-hepcidin interactions with the side chains of interacting residues shown as sticks and labeled. (A and B) Bound metal ions are indicated as yellow spheres. In B, a water molecule coordinating the metal is shown as red sphere. (C) Overlap in the binding site of hepcidin and vamifeport (obtained from a superposition of the respective complexes). (D) Closeup of the overlapping region of vamifeport and hepcidin with sidechains interacting with both molecules shown as sticks. Sidechain conformations are obtained from the hsFPN/hepcidin complex.
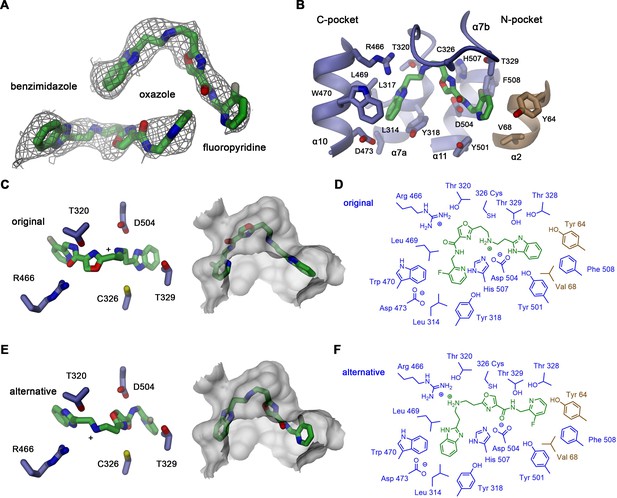
Alternative vamifeport binding mode.
(A) Vamifeport placed into its cryo-electron microscopy (cryo-EM) density in the hsFPN/Sy3/vamifeport complex (contoured at 6.5 σ) in opposite orientation. (B) Molecular interactions of the oppositely oriented vamifeport model with its binding site in hsFPN. (C) Selected interactions of vamifeport with protein side chains (left) and fit of the inhibitor into the binding pocket (right) in its original orientation displayed in Figure 4. (D) Schematic drawing of inhibitor interactions in the original orientation. (E) Selected interactions of vamifeport with protein side chains (left) and fit of the inhibitor into the binding pocket (right) in its alternative orientation defined in panels A and B. (D) Schematic drawing of inhibitor interactions in the alternative orientation. Although both orientations of the inhibitor would fit into the binding pocket, the chemical interactions with the protein are more favorable in the original orientation.
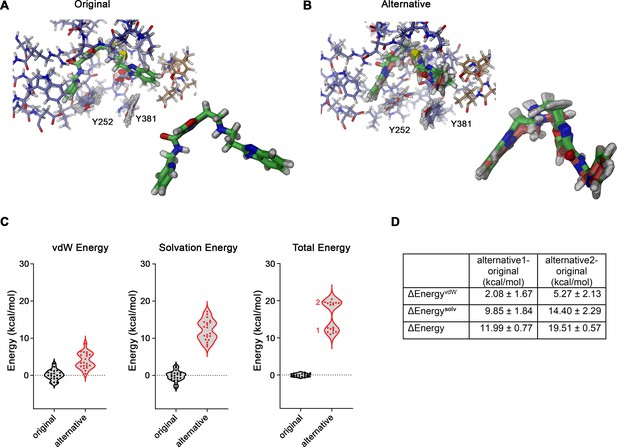
Evaluation of vamifeport interactions.
(A) Representative conformations of vamifeport in its original binding position. Inset (bottom, right) shows a superposition of the minimum-energy poses of vamifeport from 20 docking runs, which deviate marginally from the original position defined in the cryo-electron microscopy (cryo-EM) structure (mean ligand heavy-atom RMSD of 0.61 Å). (B) Representative conformations of vamifeport in its alternative binding position. Inset (bottom, right) shows a superposition of the minimum-energy vamifeport poses from 20 docking runs, which show some deviation from their original position defined in the cryo-EM structure resulting in two clusters of poses. Cluster 2 (green, 11 poses) is closer to the alternative position as defined in the cryo-EM density (mean ligand heavy-atom RMSD of 1.03 Å), and cluster 1 (red, nine poses) has somewhat deviated from this position to improve the interaction energy (mean ligand heavy-atom RMSD of 1.17 Å). (A and B) Shown are superpositions of minimum-energy conformations of short simulations of a restrained system as described in the methods. (C) Relative differences in the interaction energies of vamifeport in original and alternative orientations (compared to the median of the energy of the system in the original conformation). Shown are the differences in van der Waals (vdW, left), solvation (sum of direct mean field interaction [DMFI] and screened electrostatic terms, center), and total energy (right). Results of individual simulations are depicted as dots, and the two clusters of vamifeport positions in the alternative orientation are indicated. (D) Table of energy differences obtained from the data shown in C calculated for both clusters of vamifeport positions in the alternative orientation with respect to the original orientation. Mean and SD values in kcal/mol are reported.
-
Figure 4—figure supplement 4—source data 1
Total energies of hsFPN-vamifeport complex in original and alternative conformation obtained by docking calculations and CAMPARI keyword files and input and output files for docking calculations.
- https://cdn.elifesciences.org/articles/83053/elife-83053-fig4-figsupp4-data1-v1.zip
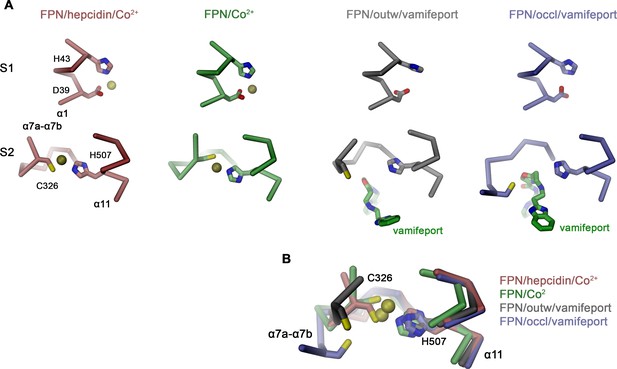
Interactions in the metal ion binding sites.
(A) Residues constituting the observed metal ion binding sites S1 (top) and S2 (bottom) in different FPN structures. Models are from: FPN/hepcidin/Co2+, PDBID 6WBV; FPN/Co2+, PDBID 6VYH; FPN/outw/vamifeport, hsFPN/Sy12/vamifeport complex; FPN/occl/vamifeport, hsFPN/Sy3/vamifeport complex. (B) Superposition of the ion binding Site S2 in different conformations illustrating the large spread of the location of C326 in different structures. (A and B) The protein is shown as Cα-trace, bound ions are displayed as yellow spheres and vamifeport and selected side chains as sticks.
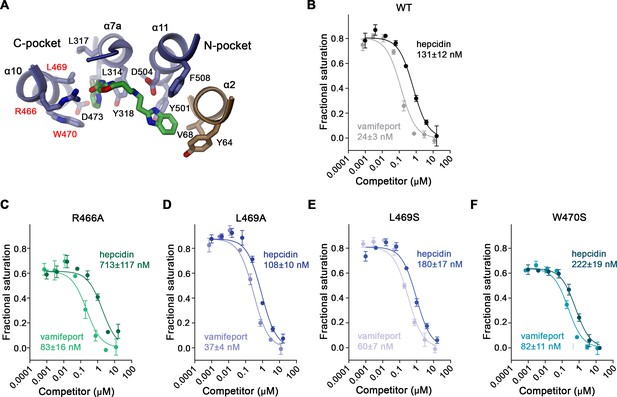
Properties of binding site mutants.
(A) Close-up of vamifeport/FPN interactions with mutated residues. Residues of the C-pocket whose mutations were characterized in detail are labeled in red. Displacement of TMR-hepcidin with unlabeled hepcidin-25 or vamifeport measured by changes in the fluorescence polarization of labeled hepcidin. Investigated constructs show: (B) hsFPN WT and the point mutants, (C) R466A, (D) L469A, (E) L469S, and (F) W470S. The KD values for the interaction between FPN/hepcidin-25 and FPN/vamifeport are displayed. Values were obtained from a fit to a competition binding model (lines) described in the methods. (B–F) Data show mean of three independent measurements; errors are SEM. Similar results were obtained in independent experiments using different preparations and concentrations of hsFPN.
-
Figure 5—source data 1
TMR-hepcidin fluorescence polarization displacement data of hsFPN WT and point mutants.
- https://cdn.elifesciences.org/articles/83053/elife-83053-fig5-data1-v1.zip
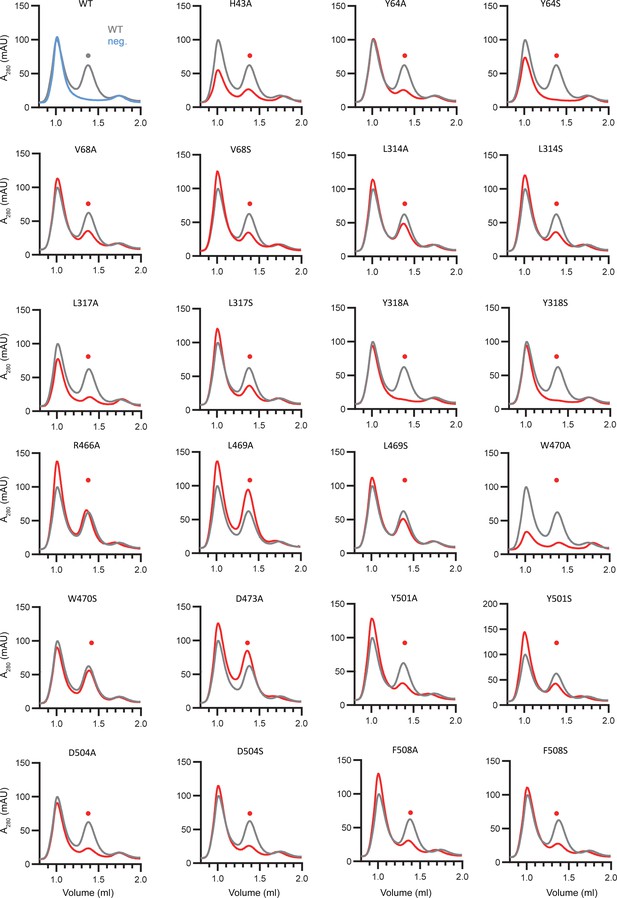
Expression of hsFPN mutants.
Fluorescence detection size-exclusion chromatography profiles of detergent solubilized extracts of hsFPN wild type (WT) and point mutants harboring the C-terminal GFP-tag. The first panel (top left) shows a comparison of traces of WT (gray) and non-transfected cells (blue, neg.) as negative control, demonstrating that the peak eluting at low volume is not related to FPN. The height of the second peak (●) indicates the monomeric transporters from mutant preparations (red) compared to WT (gray).
-
Figure 5—figure supplement 1—source data 1
Fluorescence detection size-exclusion chromatography data of detergent solubilized extracts of hsFPN WT and point mutants harboring a C-terminal GFP-tag.
- https://cdn.elifesciences.org/articles/83053/elife-83053-fig5-figsupp1-data1-v1.zip
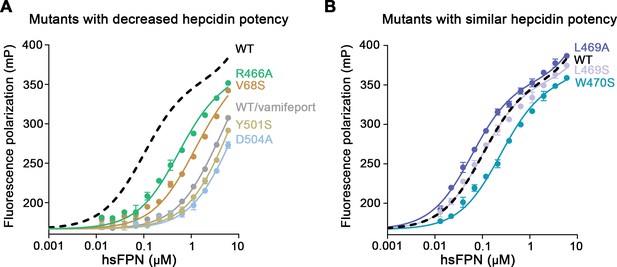
Hepcidin binding to hsFPN mutants.
Binding of fluorescently labeled hepcidin-25 (TMR-hepcidin) to increasing concentrations of hsFPN mutants determined by the change in the fluorescence polarization of the peptide. Point mutants with (A) decreased and (B) similar potency compared to wild type (WT) hsFPN (dashed black line). The gray curve in A shows an experiment with WT hsFPN in the presence of a large excess (i.e. 30 μM) of vamifeport to determine unspecific binding. Data show mean of three independent measurements, and errors are SEM. The fit yielded KD values of 100±4 nM for WT, 57±2 nM for L469A, 94±3 nM for L469S, 227±8 nM for W470S, 501±22 nM for R466A, 1044±60 nM for V68S, and about 4.5 μM and 6 μM for Y501S and D504A, respectively.
-
Figure 5—figure supplement 2—source data 1
TMR-hepcidin fluorescence polarization direct binding data of hsFPN WT and point mutants.
- https://cdn.elifesciences.org/articles/83053/elife-83053-fig5-figsupp2-data1-v1.zip
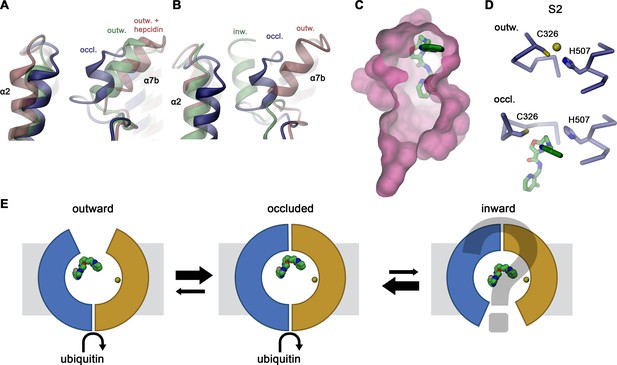
Hypothetical mechanism of FPN regulation by vamifeport.
Conformational plasticity of the extracellular region surrounding helix α7b as illustrated by a superposition of the occluded conformation of hsFPN with (A) hsFPN in outward-facing apo (PDBID 6W4S) and hepcidin-bound states (PDBID 6WBV) and (B) the prokaryotic bbFPN in outward- and inward-facing states (PDBID 5AYN and 5AYO). (C) Location of vamifeport at the extracellular rim of the occluded cavity leaves sufficient space for larger inhibitors. (D) Comparison of the metal ion binding site S2 in the outward-facing conformation of hsFPN (PDBID 6VYH) and its occluded conformation indicates competition of vamifeport with the ion binding to S2. (E) Hypothetical relationship between vamifeport-bound FPN conformations and the ability of the protein to get ubiquitinated. The possible binding of Fe2+ to the S1 site, which is not overlapping with the vamifeport binding site, is indicated. The stabilization of the occluded conformation might potentially facilitate the recruitment of the ubiquitination machinery on the intracellular side.
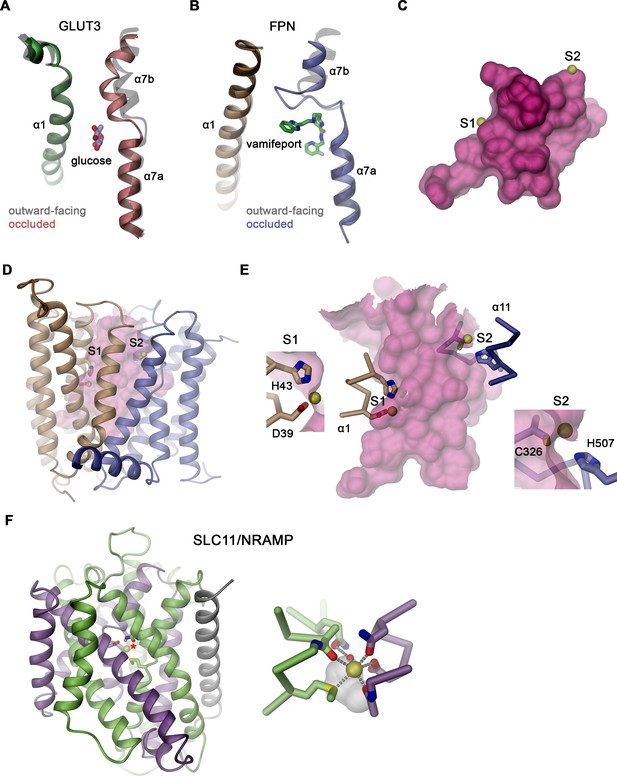
Conformational changes and substrate binding in different transporters.
Comparison of the transition of the outward-facing to the occluded conformations in (A) GLUT3 (PDBIDs 4ZWC and 4ZW9) and (B) hsFPN (PDBID 6VYH). Shown is the movement of α7 toward α1. Outward-facing structures are colored in gray, and the location of bound glucose and vamifeport is displayed. (C) Location of the presumed ion binding sites to the occluded conformation of hsFPN. The ion positions were obtained from the metal-bound outward-facing structure (PDB 6VYH). (D) View of the entire structure, and (E) close-up of the outward-facing cavity with insets showing closeup of the individual binding sites. The molecular surface is shown in magenta, bound metal ions as yellow spheres, and interacting protein residues as sticks. The proximity of the bound ions to the large cavity indicates their interaction with trapped water molecules. (F) Ribbon representation of the occluded conformation of the prokaryotic SLC11 transporter DraNRAMP (PDBID 8E60) with domains of the protein shown in unique colors. Inset (right) shows a zoom into the substrate binding site with residues coordinating the bound metal ion (yellow) displayed as sticks. The white pocket shows the only space in vicinity of the binding site that is not occupied by either the protein or the metal ion, interactions are indicated as dashed lined, and a water molecule involved in ion interactions is displayed.
Tables
Cryo-electron microscopy (cryo-EM) data collection, refinement, and validation statistics.
Dataset 1hsFPN/Sy3(EMDB-16353)(PDB 8C02) | Dataset 2hsFPN/Sy3-vamifeport(EMDB-16345)(PDB 8BZY) | Dataset 3hsFPN/Sy12-vamifeport(EMDB-16354)(PDB 8C03) | |
---|---|---|---|
Data collection and processing | |||
Microscope | FEI Titan Krios | FEI Titan Krios | FEI Titan Krios |
Camera | Gatan K3 GIF | Gatan K3 GIF | Gatan K3 GIF |
Magnification | 130,000 | 130,000 | 130,000 |
Voltage (kV) | 300 | 300 | 300 |
Electron exposure (e–/Å2) | 66 | 61/70.795 | 69.559/70.766 |
Defocus range (μm) | –2.4 to –1 | –2.4 to –1 | –2.4 to –1 |
Pixel size (Å) | 0.651 (0.3255) | 0.651 (0.3255) | 0.651 (0.3255) |
Symmetry imposed | C1 | C1 | C1 |
Initial particle images (no.) | 1,927,334 | 1,820,142 | 3,045,873 |
Final particle images (no.) | 139,011 | 320,701 | 387,990 |
Map resolution (Å) FSC threshold 0.143 | 4.09 Å | 3.24 Å | 3.89 Å |
Map sharpening b-factor (Å2) | –201.2 | –160.5 | –202.1 |
Refinement | |||
Model resolution (Å) FSC threshold 0.5 | 4.3 | 3.4 | 4.1 |
Non-hydrogen atoms | 4245 | 4314 | 3331 |
Protein residues | 549 | 549 | 429 |
Ligand | 0 | 2 | 1 |
Water | 0 | 0 | 0 |
B factors (Å2) protein | 162.30 | 168.28 | 130.63 |
B factors (Å2) ligand | --- | 116.10 | 63.72 |
RMSD Bond lengths (Å) | 0.004 (0) | 0.005 (0) | 0.004 (0) |
RMSD Bond angles (°) | 0.634 (0) | 0.732 (0) | 0.645 (0) |
MolProbity score | 1.88 | 1.63 | 2.00 |
Clashscore | 10.16 | 7.02 | 6.37 |
Poor rotamers (%) | 1.10 | 1.10 | 4.46 |
CaBLAM outliers (%) | 2.59 | 1.31 | 0.96 |
Ramachandran outliers (%) | 0.00 | 0.00 | 0.00 |
Ramachandran allowed (%) | 4.64 | 3.33 | 2.84 |
Ramachandran favored (%) | 95.36 | 96.67 | 97.16 |
Hepcidin and vamifeport binding.
hsFPN construct | KD TMR-hepcidin (nM) | KD hepcidin-25 (nM) | KD vamifeport (nM) |
---|---|---|---|
WT | 100±4 | 131±12 | 24±3 |
R466A | 501±22 | 713±117 | 83±16 |
L469A | 57±2 | 108±10 | 37±4 |
L469S | 94±3 | 180±17 | 60±7 |
W470S | 227±8 | 222±19 | 82±11 |
V68S | 1044±60 | n.d. | n.d. |
Y501S | >>1000 | n.d. | n.d. |
D504A | >>1000 | n.d. | n.d. |
-
n.d., not determined.
DNA sequences.
Codon optimized sequence of hsFPN GTACCAGAGCCGGAGATCACAACCGGCAGAGAGGCTGCTGCGGCAGCCTGGCAGATTACCTGACCTCTGCCAAATTCCTGCTGTACCTGGGCCATTCTCTGAGCACATGGGGCGACAGAATGTGGCACTTCGCCGTGTCCGTCTTTCTCGTGGAACTGTATGGAAACAGCCTGCTGCTGACTGCTGTTTACGGCCTGGTGGTGGCCGGCAGCGTGCTGGTGCTGGGAGCCATTATAGGCGATTGGGTCGACAAGAACGCCCGGCTGAAAGTGGCCCAAACAAGCCTGGTGGTGCAGAACGTGAGCGTGATCCTCTGTGGCATCATCCTGATGATGGTGTTCCTGCATAAGCACGAACTGCTGACAATGTACCACGGCTGGGTGCTGACCTCTTGCTACATCCTGATCATCACAATTGCCAATATCGCCAATCTGGCCAGCACCGCTACCGCCATCACCATTCAAAGAGATTGGATCGTGGTTGTGGCCGGCGAGGACCGGAGCAAGCTGGCTAACATGAACGCCACCATCCGGAGAATCGACCAGTTAACCAACATCCTGGCTCCTATGGCCGTGGGCCAGATCATGACATTTGGCAGCCCCGTGATCGGCTGTGGCTTCATCAGCGGCTGGAACCTCGTGTCCATGTGCGTGGAATACGTGCTGCTGTGGAAGGTGTACCAGAAAACCCCTGCCCTGGCCGTGAAGGCCGGCCTGAAGGAGGAAGAGACGGAGCTGAAGCAGCTGAACCTGCACAAGGACACCGAGCCTAAGCCTCTGGAGGGCACACACCTGATGGGAGTGAAAGACAGCAATATCCACGAACTGGAACACGAGCAGGAGCCCACCTGCGCCTCTCAGATGGCCGAGCCTTTTCGAACCTTCCGGGACGGCTGGGTGTCCTACTACAACCAGCCTGTGTTCCTGGCCGGCATGGGCCTGGCCTTCCTGTACATGACCGTGCTGGGATTCGATTGTATCACCACCGGCTACGCCTACACCCAGGGCCTGAGCGGCAGCATTCTGTCTATCCTGATGGGCGCCAGCGCCATCACCGGCATTATGGGTACAGTGGCTTTCACCTGGCTGAGAAGGAAGTGCGGACTTGTTAGAACCGGCCTGATCAGCGGCCTGGCCCAACTGTCTTGTCTGATCCTGTGCGTGATCAGCGTCTTTATGCCTGGCAGCCCTCTGGACCTGTCTGTGTCCCCATTCGAGGACATCAGATCAAGATTCATCCAGGGCGAGAGCATCACACCTACCAAGATCCCCGAGATTACCACCGAAATCTACATGAGCAACGGCTCCAACAGCGCTAACATCGTGCCCGAGACAAGCCCTGAATCTGTGCCCATCATCTCGGTGAGCCTCCTGTTCGCTGGAGTGATCGCCGCCAGAATCGGCCTGTGGTCCTTTGACCTGACAGTGACCCAGCTGCTGCAGGAGAACGTGATCGAGAGCGAGAGAGGAATCATCAACGGCGTGCAGAACAGCATGAACTACCTGCTGGATCTGCTGCACTTCATCATGGTCATCCTAGCTCCAAACCCCGAGGCCTTCGGCCTGCTGGTCCTGATCAGCGTGAGCTTCGTGGCCATGGGCCACATCATGTATTTCAGATTCGCCCAGAATACACTGGGCAACAAGCTGTTCGCATGCGGACCTGACGCCAAGGAAGTGCGGAAGGAAAATCAGGCCAATACCAGCGTGGTG |
Sequence of Sybody 1 GTTCAGCTGGTTGAGAGCGGTGGTGGCCTGGTCCAAGCTGGCGGTTCGCTGCGTCTGAGCTGCGCCGCAAGCGGTTTCCCGGTGGAACAGGGTTGGATGGCTTGGTATCGTCAGGCACCGGGCAAAGAACGTGAGTGGGTCGCGGCGATTTCTAGCTCTGGTTGGCATACGTGGTACGCAGATTCTGTTAAGGGCCGCTTTACCATCAGCCGCGACAACGCGAAGAATACGGTCTATTTGCAGATGAATAGCCTGAAACCGGAAGATACCGCGGTTTACTACTGTAACGTGAAAGACAACGGTTGGTACTCTGCTCAGTACGACTATTGGGGCCAAGGTACCCAAGTGACTGTGAGCGCAGGAAGAGCTGGCGAACAAAAACTCATCTCAGAAGAGGATCTGAATAGCGCCGTCGAC |
Sequence of Sybody 3 GTTCAGCTGGTTGAGAGCGGTGGTGGCCTGGTCCAAGCTGGCGGTTCGCTGCGTCTGAGCTGCGCCGCAAGCGGTTTCCCGGTGGCTTGGAACGAAATGCGTTGGTATCGTCAGGCACCGGGCAAAGAACGTGAGTGGGTCGCGGCGATTGCTAGCATCGGTGTTACTACGTACTACGCAGATTCTGTTAAGGGCCGCTTTACCATCAGCCGCGACAACGCGAAGAATACGGTCTATTTGCAGATGAATAGCCTGAAACCGGAAGATACCGCGGTTTACTACTGTAACGTGAAAGACTACGGTATGGCTTTCTGGTACTACGACTATTGGGGCCAAGGTACCCAAGTGACTGTGAGCGCAGGAAGAGCTGGCGAACAAAAACTCATCTCAGAAGAGGATCTGAATAGCGCCGTCGAC |
Sequence of Sybody 8 GTTCAGCTGGTTGAGAGCGGTGGTGGCCTGGTCCAAGCTGGCGGTTCGCTGCGTCTGAGCTGCGCCGCAAGCGGTTTCCCGGTGTACGCTTACAACATGGAATGGTATCGTCAGGCACCGGGCAAAGAACGTGAGTGGGTCGCGGCGATTGCTAGCTACGGTCATACTACGGAATACGCAGATTCTGTTAAGGGCCGCTTTACCATCAGCCGCGACAACGCGAAGAATACGGTCTATTTGCAGATGAATAGCCTGAAACCGGAAGATACCGCGGTTTACTACTGTAACGTGAAAGACTACGGTTACCTGTGGTACCATTACGACTATTGGGGCCAAGGTACCCAAGTGACTGTGAGCGCAGGAAGAGCTGGCGAACAAAAACTCATCTCAGAAGAGGATCTGAATAGCGCCGTCGAC |
Sequence of Sybody 11 GTTCAGCTGGTTGAGAGCGGTGGTGGCCTGGTCCAAGCTGGCGGTTCGCTGCGTCTGAGCTGCGCCGCAAGCGGTTTCCCGGTGTACTCTCGTGGTATGTACTGGTATCGTCAGGCACCGGGCAAAGAACGTGAGTGGGTCGCGGCGATTCATAGCTACGGTACTCAGACGGAATACGCAGATTCTGTTAAGGGCCGCTTTACCATCAGCCGCGACAACGCGAAGAATACGGTCTATTTGCAGATGAATAGCCTGAAACCGGAAGATACCGCGGTTTACTACTGTAACGTGAAAGACATGGGTTACCTGTGGTACCAGTACGACTATTGGGGCCAAGGTACCCAAGTGACTGTGAGCGCAGGAAGAGCTGGCGAACAAAAACTCATCTCAGAAGAGGATCTGAATAGCGCCGTCGAC |
Sequence of Sybody 12 GTTCAGCTGGTTGAGAGCGGTGGTGGCCTGGTCCAAGCTGGCGGTTCGCTGCGTCTGAGCTGCGCCGCAAGCGGTTTCCCGGTGTGGAACTCTCGTATGTTCTGGTATCGTCAGGCACCGGGCAAAGAACGTGAGTGGGTCGCGGCGATTGCTAGCAAAGGTTACTCTACGCATTACGCAGATTCTGTTAAGGGCCGCTTTACCATCAGCCGCGACAACGCGAAGAATACGGTCTATTTGCAGATGAATAGCCTGAAACCGGAAGATACCGCGGTTTACTACTGTAACGTGAAAGACGACGGTTGGTGGTGGCAGACTTACGACTATTGGGGCCAAGGTACCCAAGTGACTGTGAGCGCAGGAAGAGCTGGCGAACAAAAACTCATCTCAGAAGAGGATCTGAATAGCGCCGTCGAC |
Primers for mutagenesis.
Mutation | Primer sequences |
---|---|
Y64A | for: GCTGACTGCTGTTGCCGGCCTGGTGGTGGCCGGCAGC rev: AACAGCAGTCAGCAGCAGGCTGTTTCCATACAGTTCCACG |
Y64S | for: GCTGACTGCTGTTTCCGGCCTGGTGGTGGCCGGCAGC rev: AACAGCAGTCAGCAGCAGGCTGTTTCCATACAGTTCCACG |
V68A | for: GCTGACTGCTGTTTACGGCCTGGTGGCGGCCGGCAGC rev: AACAGCAGTCAGCAGCAGGCTGTTTCCATACAGTTCCACG |
Y68S | for: GCTGACTGCTGTTTACGGCCTGGTGTCGGCCGGCAGC rev: AACAGCAGTCAGCAGCAGGCTGTTTCCATACAGTTCCACG |
L314A | for: TGACCGTGCTGGGATTCGATTGTATCACCACCGGCTACG rev: CCCAGCACGGTCATGTACAGGAAGGCCGCGCCCATGC |
L314S | for: TGACCGTGCTGGGATTCGATTGTATCACCACCGGCTACG rev: CCCAGCACGGTCATGTACAGGAAGGCCGAGCCCATGC |
L317A | for: TGACCGTGCTGGGATTCGATTGTATCACCACCGGCTACG rev: CCCAGCACGGTCATGTACGCGAAGGCCAGGCCCATGC |
L317S | for: TGACCGTGCTGGGATTCGATTGTATCACCACCGGCTACG rev: CCCAGCACGGTCATGTACGAGAAGGCCAGGCCCATGC |
Y318A | for: TGACCGTGCTGGGATTCGATTGTATCACCACCGGCTACG rev: CCCAGCACGGTCATGGCCAGGAAGGCCAGGCCCATGC |
Y318S | for: TGACCGTGCTGGGATTCGATTGTATCACCACCGGCTACG rev: CCCAGCACGGTCATGGACAGGAAGGCCAGGCCCATGC |
R466A | for: GAGTGATCGCCGCCGCAATCGGCCTGTGGTCCTTTGACCTGACAG rev: GCGGCGATCACTCCAGCGAACAGGAGGCTCACCGAGATGATGG |
L469A | for: GAGTGATCGCCGCCAGAATCGGCGCGTGGTCCTTTGACCTGACAG rev: GCGGCGATCACTCCAGCGAACAGGAGGCTCACCGAGATGATGG |
L469S | for: GAGTGATCGCCGCCAGAATCGGCTCGTGGTCCTTTGACCTGACAG rev: GCGGCGATCACTCCAGCGAACAGGAGGCTCACCGAGATGATGG |
W470A | for: GAGTGATCGCCGCCAGAATCGGCCTGGCGTCCTTTGACCTGACAG rev: GCGGCGATCACTCCAGCGAACAGGAGGCTCACCGAGATGATGG |
W470S | for: GAGTGATCGCCGCCAGAATCGGCCTGTCGTCCTTTGACCTGACAG rev: GCGGCGATCACTCCAGCGAACAGGAGGCTCACCGAGATGATGG |
D473A | for: GAGTGATCGCCGCCAGAATCGGCCTGTGGTCCTTTGCCCTGACAG rev: GCGGCGATCACTCCAGCGAACAGGAGGCTCACCGAGATGATGG |
Y501A | for: GAACAGCATGAACGCCCTGCTGGATCTGCTGCACTTCATCATGG rev: GTTCATGCTGTTCTGCACGCCGTTGATGATTCCTCTCTCG |
Y501S | for: GAACAGCATGAACTCCCTGCTGGATCTGCTGCACTTCATCATGG rev: GTTCATGCTGTTCTGCACGCCGTTGATGATTCCTCTCTCG |
F508A | for: GAACAGCATGAACTACCTGCTGGATCTGCTGCACGCCATCATGG rev: GTTCATGCTGTTCTGCACGCCGTTGATGATTCCTCTCTCG |
F508S | for: GAACAGCATGAACTACCTGCTGGATCTGCTGCACTCCATCATGG rev: GTTCATGCTGTTCTGCACGCCGTTGATGATTCCTCTCTCG |