ARHGAP18-ezrin functions as an autoregulatory module for RhoA in the assembly of distinct actin-based structures
Figures
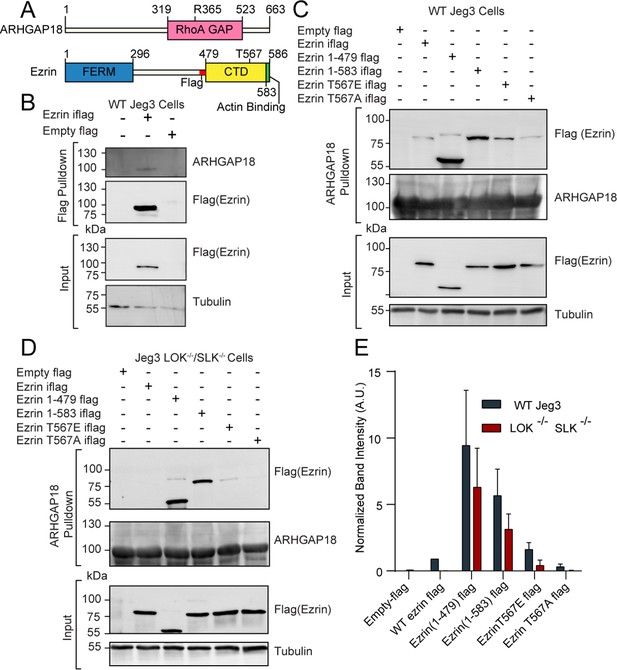
ARHGAP18 binds active, open ezrin through the FERM domain.
(A) Protein domain schematics of human ARHGAP18 and ezrin. (B) Western blot from pulldown experiment from passing WT-Jeg3 placental epithelial cell lysate over an anti-flag resin then blotting against flag-tagged ezrin and endogenous ARHGAP18. Ezrin tagged internally (iflag) within a flexible linker region at residue 479. (C) Western blot of ARHGAP18-bound resin pulldown experiment blotting against ezrin constructs expressed in WT-Jeg3 cells. (D) Western blot of ARHGAP18-bound resin pulldown experiment blotting against ezrin-constructs expressed in LOK-/-SLK-/--Jeg3 cells. (E) Quantification of normalized band intensity from all experiment presented in (C, D). Bars represents mean ± SEM; n = 4. Aggregated WT and LOK-/-SLK-/- conditions as populations were compared using a ratio paired t-test and found to be significantly different (p=0.0235).
-
Figure 1—source data 1
Full western blot images from all blots shown in Figure 1.
- https://cdn.elifesciences.org/articles/83526/elife-83526-fig1-data1-v2.zip
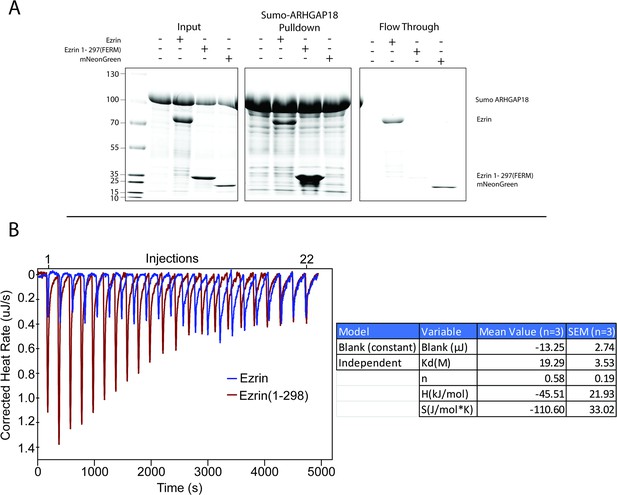
ARHGAP18 affinity for ezrin using purified proteins.
(A) Coomassie stained gels from assay testing the binding of full-length ezrin, ezrin-FERM (1–297), and mNeonGreen (negative control) to a resin saturated with SUMO-ARHGAP18. From left to right: input proteins, pulled-down proteins, flowthrough proteins. Both FL-ezrin and mNeon green are observed in the flowthrough while FERM is only found bound to the column. FL-ezrin shows weaker affinity for the column than FERM, whereas mNeonGreen did not bind the SUMO-ARHGAP18 column. (B) Representative injections (above) and plotting of area under each injection peak with fit (below). FL-WT ezrin did not show measurable binding to ARHGAP18 while FERM produced measurable binding curves. Reported Kd is (mean ± SEM) from replicates of individual isothermal calorimetry (ITC) experiments.
-
Figure 1—figure supplement 1—source data 1
Full western blot images from all blots shown in Figure 1—figure supplement 1.
- https://cdn.elifesciences.org/articles/83526/elife-83526-fig1-figsupp1-data1-v2.zip
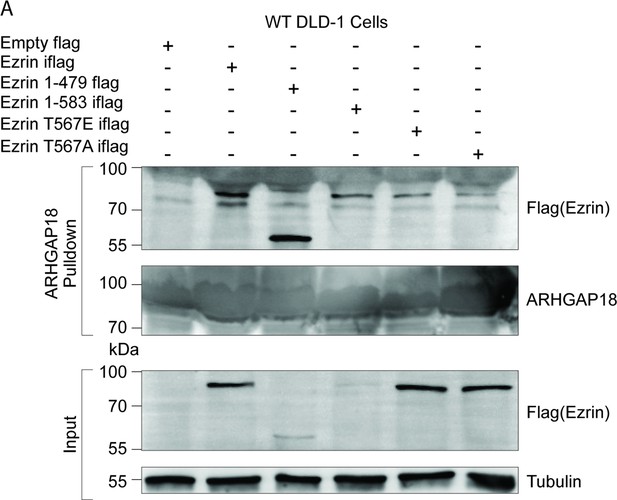
ARHGAP18 binds active, open ezrin through the FERM domain in DLD-1 cells.
(A) Western blot replication of experiment in Figure 1C except done in DLD-1 human colorectal cells instead of placental Jeg3 cells. Expression of constitutively active forms of ezrin and FERM is detrimental to many cell types leading to lower expression. Even with much reduced expression, comparison of input to pulldown shows a preferential binding of FERM to ARHGAP18 compared to WT ezrin.
-
Figure 1—figure supplement 2—source data 1
Full western blot images from all blots shown in Figure 1—figure supplement 2.
- https://cdn.elifesciences.org/articles/83526/elife-83526-fig1-figsupp2-data1-v2.zip
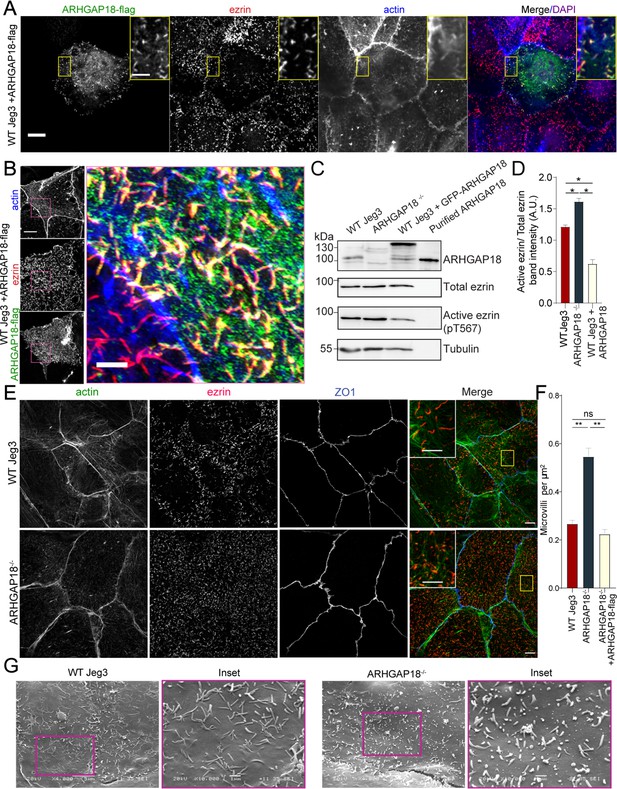
ARHGAP18 localizes to microvilli and alters ezrin T567 phosphorylation.
(A) Confocal immunofluorescence images of WT-Jeg3 cells expressing ARHGAP18-flag. Scale bar 10 μm; yellow box inset scale 2 μm. (B) Structured illumination microscopy (SIM) reconstructions of WT-Jeg3 cells expressing ARHGAP18-flag with zoom in on boxed area. Scale bar 10 μm; inset 2 μm. (C) Western blot showing CRISPR KO of endogenous ARHGAP18 and ezrin pT567 phosphorylation relative to total ezrin. Antibody against ARHGAP18 was confirmed in part by identification of endogenous ARHGAP18, GFP-ARHGAP18, and purified ARHGAP18 while showing no detection in KO cells. (D) Quantification of normalized band intensity from representative experiments presented in (C). Bars represent mean ± SEM; n = 4 paired t-test; *p≤0.05. (E) SIM images of WT vs. ARHGAP18-/- Jeg3 cells with ezrin as a marker for the abundance of microvilli at the apical surface. Scale bar 10 μm; yellow box inset scale 2 μm (F) Quantification of the density of microvilli on the surface of Jeg3 cells in WT, ARHGAP18-/-, and ARHGAP18 overexpression conditions. Microvilli counts per cell were normalized to the area of each cell to account for cell size variability. Bars represents mean ± SEM; n = 3 replicates; t-test; **p≤0.001. (G) Scanning electron microscopy (SEM) micrographs of WT-Jeg3 cells’ apical surface compared to those of ARHGAP18-/- cells. Left panels imaged at 4000×; scale 5 μm. Right panels imaged at 10,000× within of the boxed areas; scale 1 μm.
-
Figure 2—source data 1
Full western blot images from all blots shown in Figure 2.
- https://cdn.elifesciences.org/articles/83526/elife-83526-fig2-data1-v2.zip
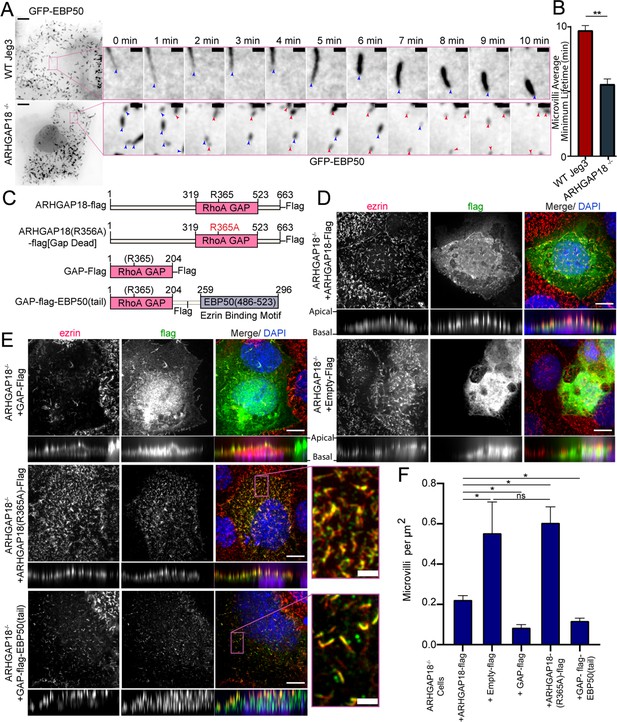
ARHGAP18 KO rescue is dependent on ezrin binding and GAP-activity.
(A) Larger images are the first frame of Video 3 with inset zoom-in of the magenta box showing frames from the time course. Each frame shows the tip of the microvilli present in the inset image at t = 0 (blue arrowhead). In the ARHGAP18-/- condition, the original microvilli disappear and are replaced by new microvilli not present in the first image (magenta arrowheads). Scale bars 10 μm; inset 1 μm. (B) Quantification of minimum microvilli lifetime from (A) (bars represents mean ± SEM; n = 151; t-test; ** p≤0.001). (C) Schematic of constructs used in this figure. (D) Immunofluorescence confocal images of ARHGAP18-/- Jeg3 cells expressing flag-tagged WT-ARHGAP18-flag (rescue) or the control flag vector. XY images above; side projections showing apical/basolateral localization below. Scale bars 10 μm. (E) Immunofluorescence confocal images of ARHGAP18-/- Jeg3 cells expressing ARHGAP18(319–523) GAP domain only, a point mutant GAP-dead variant or a chimera variant of the GAP domain of ARHGAP18(319–523) linked to the ezrin binding motif of EBP50(486–523). Expressing the GAP domain alone does not target to microvilli, disrupts microvilli formation and ezrin targeting to the apical surface. The GAP-dead variant is localized to microvilli and apical ezrin but does not rescue the knockout microvilli phenotype (zoomed inset). GAP-flag-EBP50(tail) targets to microvilli (inset), is sufficient to reduce microvilli abundance in the ARHGAP18-/- cells, and ensures ezrin localization to the apical surface. Scale bars 10 μm, insets 2 μm. (F) Quantification of the density of microvilli on the surface of ARHGAP18-/- cells expressing the conditions shown in (C–E). Microvilli counts per cell were normalized to the area of each cell to account for cell size variability. ARHGAP18-/-+ARHGAP18-flag condition duplicated from Figure 2F for comparison between conditions. Bars represents mean ± SEM; n ≥ 3; t-test; *p≤0.05. Side projections expanded in Z-dimension for clarity.
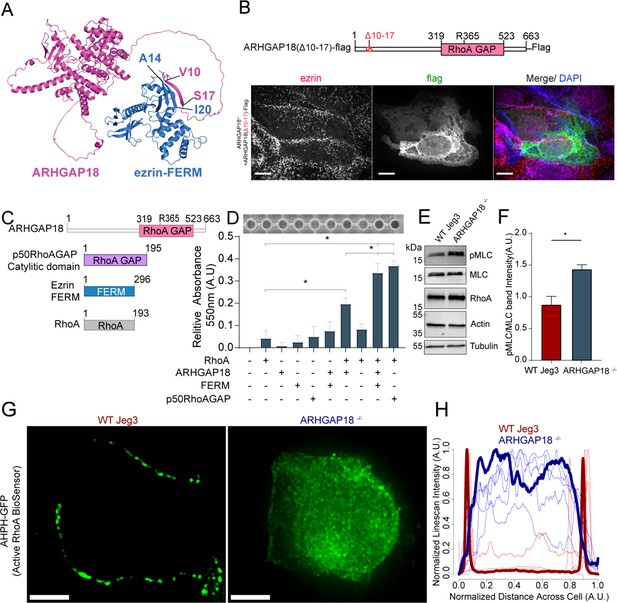
Ezrin binding to ARHGAP18 enhances GAP activity excluding active RhoA inside microvilli.
(A) AlphaFold2 structural interaction prediction of full-length human ARHGAP18 (magenta) with human ezrin-FERM domain (blue). (B) Schematic of and of ARHGAP18(Δ10–17)-flag construct and fluorescent images of ARHGAP18-/- Jeg3 cells expressing the construct which localizes diffusely throughout the cytoplasm. (C) Cartoon schematic of purified proteins used in (D). (D) Representative image of RhoA GAP assay and graph of combined results from multiple assays. Each assay normalized to the buffer only condition. Bars represents mean ± SEM; n = 4; t-test; *p≤0.05. (E) Western blotting indicating changes in phosphorylated myosin regulatory light chain (pMLC) in response to altered expression of ARHGAP18 in Jeg3 cells. (F) Quantification of pMLC over total MLC from replicates of the data in (E). (F) Bars represents mean ± SEM; n = 4; t-test; *p≤0.05. (G) Z-projection confocal fluorescent images of active RhoA biosensor. Scale bars 10 μm. (H) Line scan intensity profiles of RhoA biosensor across single WT (red) and ARHGAP18-/- (blue) cells. Line scans from the cells shown in (G) are bold while replicate cells shown as thinner lines (n = 11 cells).
-
Figure 4—source data 1
Full western blot images from all blots shown in Figure 4.
- https://cdn.elifesciences.org/articles/83526/elife-83526-fig4-data1-v2.zip
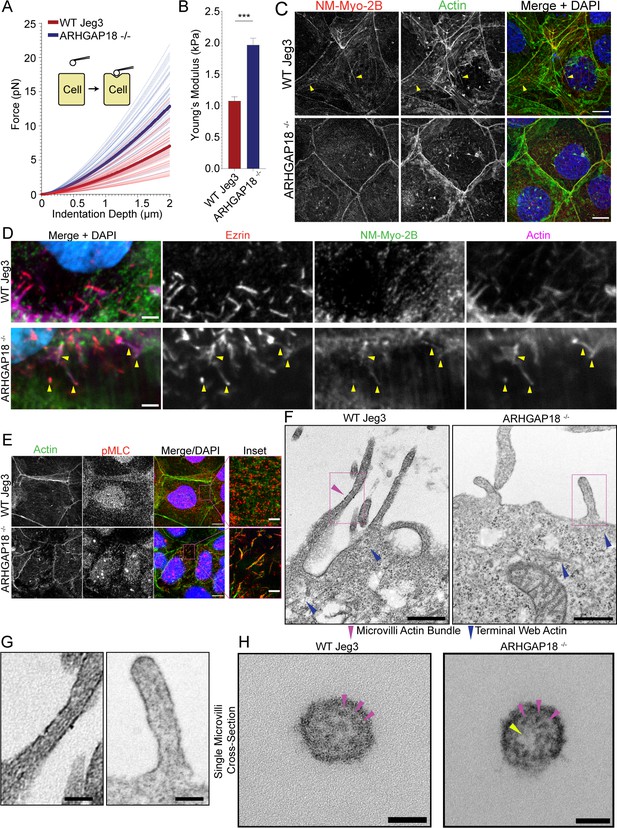
ARHGAP18 regulates actomyosin activation and organization in microvilli.
(A) Graph of force as a function of indentation depth. As the probe tip is indented against the cell surface, the force increases following a Hertzian model. Each trace indicates the fit curve from an individual cell indentation profile comparing ARHGAP18-/- cells (blue) to WT Jeg3 cells (red). The bold lines represent the average curve for each condition. (B) Average Young’s modulus WT = 1.1 ± 0.1 kPa; ARHGAP18-/- = 2.0 ± 0.1 kPa (mean ± SEM); error bars represent the SEM. Significance: t-test; p<0.0001; n = 49 WT cells and 54 ARHGAP18-/- cells. (C) Representative maximum Z-projections of immunofluorescence structured illumination microscopy (SIM) images of fixes cells showing non-muscle myosin-2B localization (yellow arrows show contractile fibers). Scale bars 10 μm. (D) Immunofluorescence staining of ezrin and non-muscle myosin-2B showing colocalization of actin, ezrin, and non-muscle myosin to microvilli in cells lacking ARHGAP18 imaged using SoRa confocal Z-projected slices of the apical surface. Scale bars 2 µm. (E) Immunofluorescence staining of phosphorylated myosin regulatory light chain (pMLC) in WT Jeg3 cells vs ARHGAP18-/- cells inset showing localization to the microvilli in the KO cells. Scale bars 10 μm; inset 2 μm. (F) Representative transmission electron microscopy (TEM) of actin at the microvilli core bundle (magenta arrowhead) to terminal web (blue arrowhead) interface. The actin core bundle in WT cells is made from aligned tightly bundled actin filaments; however, the actin in microvilli of cells lacking ARHGAP18 is less organized and aligned bundled actin is not visible. The core bundle embeds into the terminal web actin (blue arrowheads) which is made from a delicate network of actin in both conditions and has no visibly apparent differences between conditions. Scale bars 500 nm. (G) Zoom-in of the boxed areas from (F). Scale bars 125 nm. (H) Cross section of a single microvillus where individual actin filaments making up the core actin bundle are resolved (magenta arrowheads). The ARHGAP18-/- microvillus shows a central region of lighter electron density or ‘hole’ where there are no actin filaments (yellow arrowhead). In contrast, the WT cell microvillus shows actin filaments throughout the internal volume. Scale bars 50 nm.
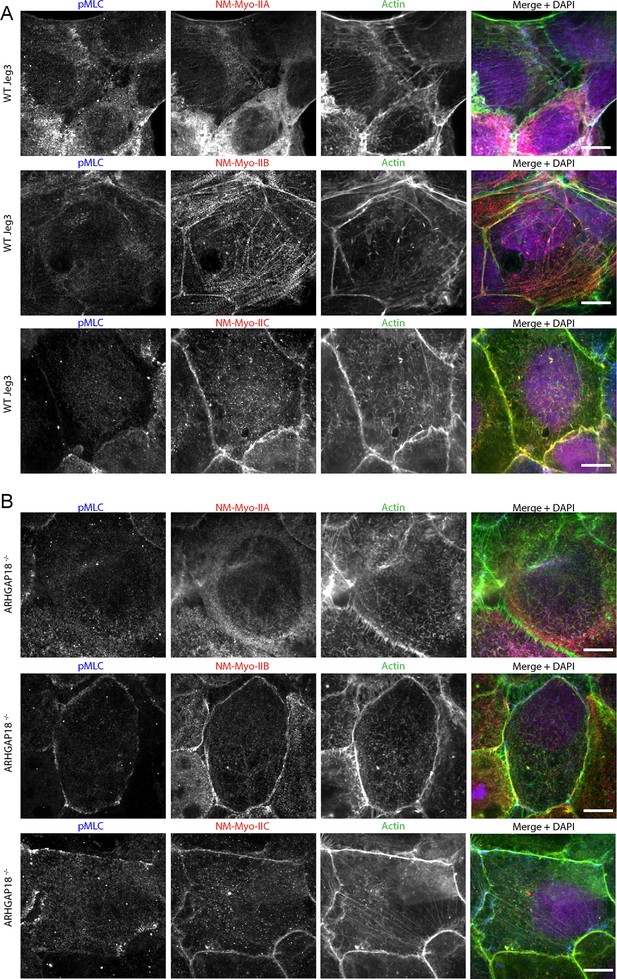
Comparison of phospho-myosin light chain, non-muscle myosin-2A, B, or C, and actin in WT Jeg3 or ARHGAP18-/- cells.
Representative confocal immunofluorescence images of the apical surface of fixed cells. Maximum Z-projections of just the apical surface were made by limiting slices where microvilli were visible. The mouse anti-pMLC antibody shown here allows for colocalization with either of the three NM-myo-2 isoforms; however, it shows significant non-specific background compared with the rabbit-pMLC shown in Figure 5. In both (A) WT cells and (B) ARHGAP18-/- cells, NM-myo2A and B largely overlap in their localizations, while NM-Myo-2C is comparatively less localized to large contractile fibers.
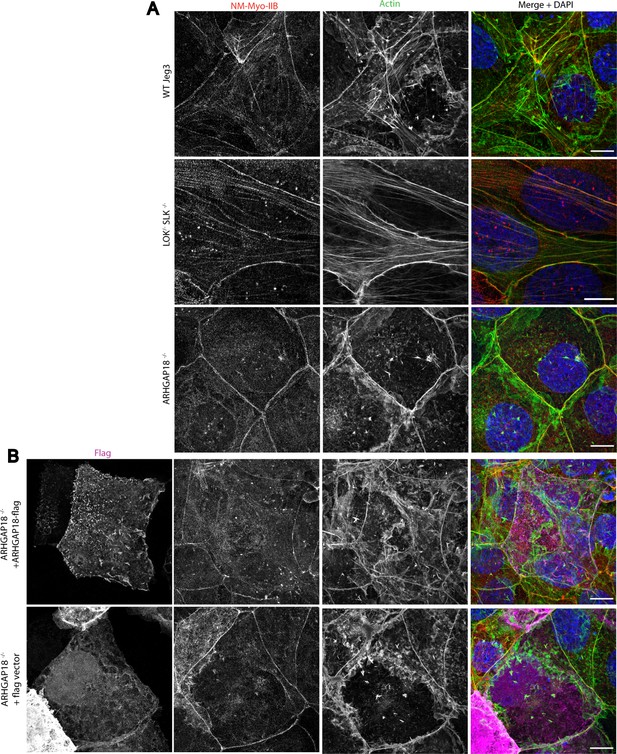
Comparison of non-muscle myosin-2B, and actin in WT Jeg3, LOK-/-SLK-/- ARHGAP18-/-, and ARHGAP18 rescue with empty vector control.
Representative maximum Z-projections of immunofluorescence structured illumination microscopy (SIM) images of fixed cells. (A) WT and ARHGAP18-/- images are the same as Figure 5A shown here to present direct comparison to rescue, control, and LOK/SLK knockout conditions. Large contractile fibers at the apical surface are increased in LOK-/- SLK-/- while reduced in the ARHGAP18-/- compared to WT Jeg3. (B) Rescue of ARHGAP18-/- with a flag-tagged ARHGAP18 but not empty vector restores actin back to near WT conditions most clearly seen in the actin channel. Scale bars 10 µm.
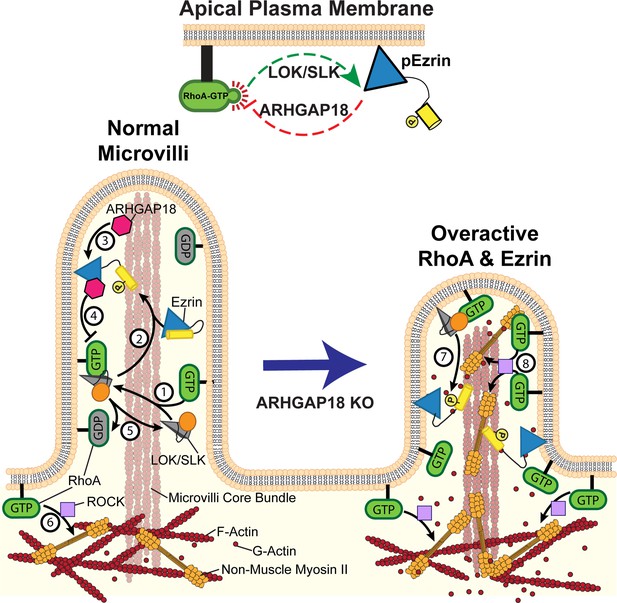
Model of ezrin and RhoA feedback loops in microvilli.
(1) Active RhoA binds and activates LOK and SLK to activate ezrin through their kinase domain (orange circle). These kinases are specifically localized to the microvilli of epithelial cells. (2) In cooperation with PIP2, the kinases phosphorylate and activate ezrin. Once active, ezrin links the plasma membrane to the actin core bundle within microvilli and also makes available a binding site for ARHGAP18. (3) ARHGAP18 binds the FERM domain of ezrin and this activates its RhoA-GAP activity. (4) This activity locally reduces the concentration of active-RhoA in microvilli, thereby modulating the ability of RhoA to activate LOK to phosphorylate ezrin. (5) Inactive Rho-GDP cannot bind or activate LOK kinase, thus completing a negative feedback system (top center of figure). (6) In the terminal web, RhoA-GTP activates the assembly of non-muscle myosin-2 through the ROCK pathway. In ARHGAP18 knockout cells, excessive active RhoA in the microvilli (7) overactivates ezrin through the action of LOK/SLK and (8) promotes inappropriate assembly of myosin-2 through the activity of ROCK. These changes result in increased microvilli formation (through ERMs) and disruption of the F-actin core bundle within microvilli (through non-muscle myosin-2).
Videos
Time-lapse movie of live WT Jeg3 vs. ARHGAP18-/- cells transiently expressing GFP-EBP50 which targets to microvilli.
Each frame taken at 1 min intervals. ARHGAP18-/- cells have more but smaller microvilli, which turn over more rapidly than in the WT condition. Scale bars 5 µm.
Time-lapse movie of live WT Jeg3 vs. ARHGAP18-/- cells transiently expressing GFP-EBP50.
Each frame taken at 1 min intervals. Tracking of Individual microvilli is shown. Scale bars 5 µm.
Time-lapse movie of live WT Jeg3 vs. ARHGAP18-/- cells transiently expressing GFP-EBP50.
Each frame taken at 1 min intervals. Still frames of selected individual microvilli shown in Figure 3A. Scale bars 5 µm.
Tables
Reagent type (species) or resource | Designation | Source or reference | Identifiers | Additional information |
---|---|---|---|---|
Gene (Homo sapiens) | ARHGAP18 | ensembl.org | ENSG00000146376 | |
Strain, strain background (H. sapiens) | OneShot TOP10 (DH10B) | Thermo Fisher | C404010 | Chemically competent cells |
Cell line (H. sapiens) | Jeg-3 | ATCC.org | Htb-36 | |
Cell line (H. sapiens) | DLD-1 | ATCC.org | CCL-221 | |
Cell line (H. sapiens) | Jeg-3 LOK-/-SLK-/- | Zaman et al., 2021 | LOK-/-SLK-/- | LOK and SLK knock out cells |
Cell line (H. sapiens) | Jeg-3 ARHGAP18-/- | This paper | ARHGAP18-/- Clone 2–4 | ARHGAP18 knock out cells |
Antibody | Anti-ARHGAP18 rabbit (polyclonal) | This paper | B116 | Antigen full-length human ARHGAP18 (1:1000 for WB) |
Antibody | Anti-ezrin rabbit (polyclonal) | Bretscher, 1989 | B64 | Antigen full-length human Ezrin (1:1000 for WB) |
Antibody | Anti-phospho-ERM antibody rabbit (polyclonal) | Hanono et al., 2006 | B81 | Detects pT567 on ezrin, radixin, and moesin (1:1000 for WB) |
Antibody | Anti-phospho-myosin light chain, rabbit (polyclonal) | Cell Signaling Technology | 3674 | Against phospho-Thr18/Ser19 (1:500 for WB) |
Antibody | Anti-non-muscle myosin-2B, rabbit (polyclonal) | BioLegend | 909902 | (1:100 for WB) |
Recombinant DNA reagent | Human ARHGAP18 | Harvard Plasma Database | HsCD00379004 | cDNA of ARHGAP18 |
Recombinant DNA reagent | EBP50-GFP | Garbett and Bretscher, 2012 | ATL 1469 | Backbone pEGFP-C2 |
Recombinant DNA reagent | AHPH-GFP | Piekny and Glotzer, 2008 | Addgene; 68026 | Backbone pEGFP-C1 |
Recombinant DNA reagent | SUMO-ARHGAP18 | This paper | ATL 4748 | Backbone: pE-SUMO |
Recombinant DNA reagent | ARHGAP18-flag | This paper | ATL 3160 | Backbone: pQCXIP |
Recombinant DNA reagent | ARHGAP18-pLentiCrispr-V2 | This paper | ATL 4740 | Backbone: lentiCrispr-V2 |
Commercial assay or kit | RhoA GAP assay kit | Cytoskeleton Inc | BK105 |
Additional files
-
MDAR checklist
- https://cdn.elifesciences.org/articles/83526/elife-83526-mdarchecklist1-v2.pdf
-
Supplementary file 1
Contains plasmids used, oligonucleotides used, and a table of all quantified data with summary statistics.
- https://cdn.elifesciences.org/articles/83526/elife-83526-supp1-v2.xlsx