Columnar neurons support saccadic bar tracking in Drosophila
Figures
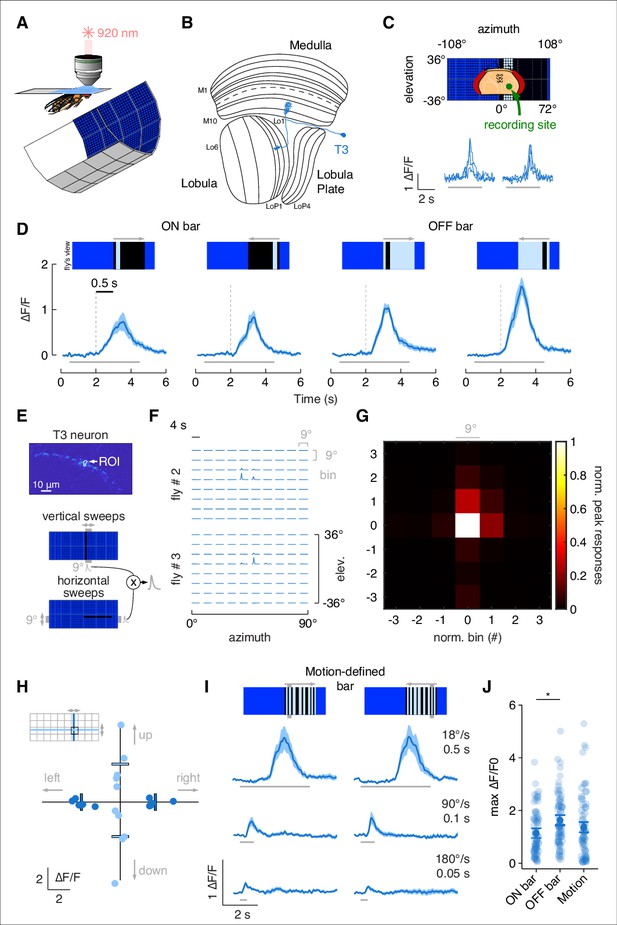
T3s are omnidirectional neurons with small receptive fields (RFs) and broad temporal sensitivity.
(A) Head-fixed fly for two-photon calcium imaging while presented with visual stimuli from a surrounding LED display. (B) Fly optic lobe neuropils (medulla, lobula, and lobula plate) with a T3 neuron highlighted in blue. (C) Top: schematic representation of the fly head and the recording site framed in the center of the LED display. Bottom: calcium imaging responses to an ON solid moving bar at 18° s–1 (left: front-to-back; right: back-to-front) of a T3 neuron from a representative fly (three repetitions). (D) Average responses (mean ± SEM) to moving ON and OFF solid bars (9° × 72°, width × height) at 18° s–1 in two different directions (front-to-back and back-to-front). Visual stimuli are depicted at the top. Dashed vertical gray lines indicate the coarse onset of the responses. Light gray horizontal bars at the bottom indicate stimulus presentation (n = 11 flies, three repetitions per fly). (E) Top: region of interest (ROI) drawn around the presynaptic terminal in the lobula of a single T3 neuron expressing GCaMP6f. Image representing the mean fluorescence from the two-photon imaging over the entire experiment in a representative fly. Bottom: representation of the procedure used to compute the RF of a single T3 neuron recording from its presynaptic terminal. Gray shaded region behind the LED display represents a bin (9° × 72°, width × height) within which a single pixel dark bar (2.25° width) is swept in two different directions. Calcium responses to vertical and horizontal sweeps are multiplied together. (F) Matrix of the multiplied traces in the 10 × 8 bins (horizontal × vertical) in two representative flies. The RF is probed within a window of 90° × 72° (horizontal × vertical). (G) Mean of the normalized peak responses by spatial location in individual T3 neurons (n = 5 flies, one repetition per fly). Bin = 0 represents the center of the RF. (H) Directional calcium peak responses to a 2.25° dark bar moving (18° s–1) in the four cardinal directions of individual flies. Bars indicate the mean. Colors depict motion along different axes (horizontal: deep blue; vertical: light blue). (I) Average responses (mean ± SEM) to motion-defined bars (9° × 72°, width × height) moving in two different directions (front-to-back and back-to-front) at three different speeds (times on the right indicate how long it takes from the leading to the trailing edges). Light gray horizontal bars at the bottom indicate stimulus presentation (n = 11 flies, three repetitions per fly). (J) Maximum amplitude of the responses to ON, OFF, and motion-defined bars moving at 18° s–1 (front-to-back and back-to-front responses are pulled together). Dark dots indicate the mean, the horizontal bars indicate SEM, and light dots represent peak amplitudes in single recordings. A linear mixed model was used to fit the data and ANOVA with pairwise post-hoc comparisons using t-tests adjusted with Bonferroni method were used to compare the predictions related to the types of bar (ON vs. OFF, p=0.01, Cohen’s d = –0.51; ON vs. motion-defined: p=0.56, Cohen’s d = –.023; OFF vs. motion-defined: p=0.33, Cohen’s d = 0.28).
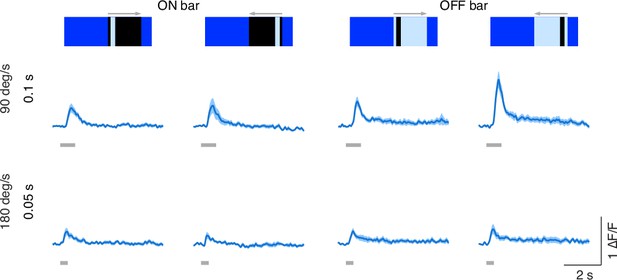
Speed sensitivity of T3 neurons.
Average responses (mean ± SEM) of T3 neurons to ON and OFF solid bars (9° × 72°, width × height) moving in two different directions (front-to-back and back-to-front) at two different speeds. Visual stimuli are depicted at the top. Light gray horizontal bars at the bottom indicate stimulus presentation (n = 11 flies, three repetitions per fly).
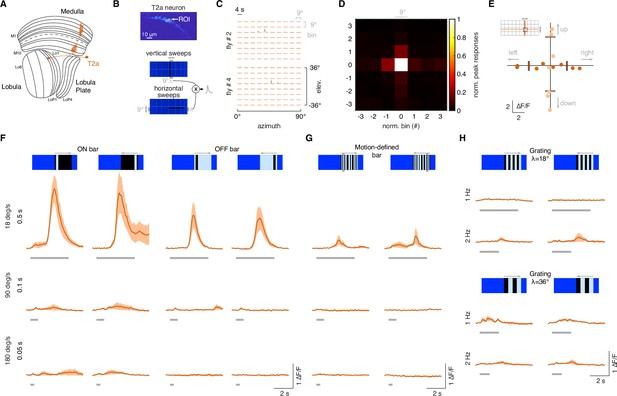
T2a neurons do not show a broad temporal sensitivity.
(A) Left: schematic representation of a T2a neuron (orange) within the optic lobe. (B) Top: region of interest (ROI) drawn around the presynaptic terminal in the lobula of a single T2a neuron expressing GCaMP6f. Image representing the mean fluorescence from the two-photon imaging over the entire experiment in a representative fly. Bottom: representation of the procedure used to probe the RF of a single T2a (as done in Figure 1E). (C) Matrix of the responses obtained by multiplying horizontal and vertical sweeps in two representative flies. (D) Mean of the normalized peak responses by spatial location in individual T2a neurons (n = 4 flies, four repetition per fly). Bin = 0 represents the center of the receptive field (RF). (E) Directional calcium peak responses to a 2.25° dark bar moving (18° s–1) in the four cardinal directions of individual flies. Colors depict motion along different axes (horizontal: deep orange; vertical: light orange). (F) Average responses (mean ± SEM) to moving ON and OFF solid bars (9° × 72°, width × height) at three different speeds (times on the left indicate how long it takes from the leading to the trailing edges) in two different directions (front-to-back and back-to-front). Visual stimuli are depicted at the top. Light gray horizontal bars at the bottom indicate stimulus presentation (n = 9 flies, three repetitions per fly). (G) Average responses (mean ± SEM) to motion-defined bars moving in two different directions (front-to-back and back-to-front) at three different speeds. (H) Top: T2a responses to a grating of λ = 18° moving front-to-back and back-to-front at two different temporal frequencies. Bottom: T2a responses to a grating of λ = 36° moving front-to-back and back-to-front at two different temporal frequencies.
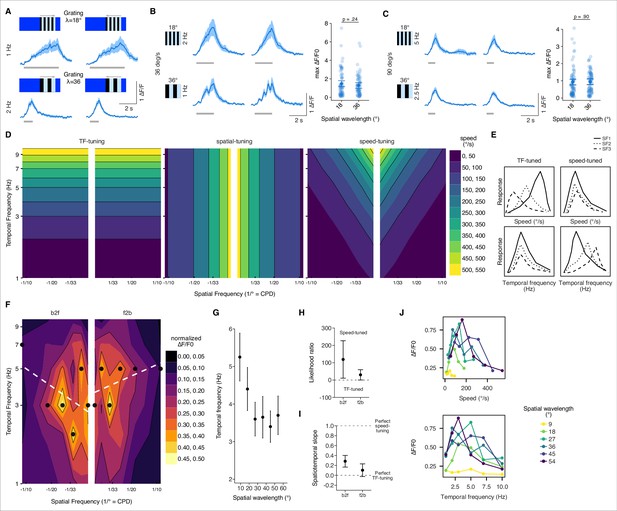
Speed tuning of T3 neurons.
(A) Average responses of T3 to moving gratings of different spatial frequency (SF) and temporal frequency (TF) (n = 11 flies, three repetitions per fly). (B) Left: T3 neurons show similar responses for gratings moving at 36° s–1 regardless of the SF of the stimuli (λ = 18°, top; λ = 36°, bottom). Right: maximum amplitude of the responses to gratings of different spatial wavelengths (18° and 36°) moving at 36° s–1 (front-to-back and back-to-front responses are pulled together). Dark dots indicate the mean, the horizontal bars indicate SEM, and light dots represent peak amplitudes in single recordings. A linear mixed model was used to fit the data and a t-test was used to compare the predictions related to the different spatial wavelengths (18° vs. 36°, p=0.24, Cohen’s d = 0.21). (C) Left: T3 neurons show similar responses for gratings moving at 90° s–1 regardless of the SF of the stimuli (λ = 18°, top; λ = 36°, bottom). Right: as in (B), maximum amplitude of the responses to gratings of different spatial wavelengths moving at 90° s–1 (18° vs. 36°, p=0.90, Cohen’s d = –0.02). (D) Left: theoretical spatiotemporal plot on a log-log scale of a model tuned to the TF of the visual patterns (TF is in Hz and SF in cycle per degree [CPD]). Colored bands represent equal TFs. Middle: theoretical spatiotemporal plot of a spatial tuned model. Colored bands represent equal spatial frequencies. Right: theoretical spatiotemporal plot of a speed-tuned model. Colored bands represent equal speeds. (E) Hypothetical tuning curves of a visual neuron where the y-axis represents the calcium response. Different types of lines represent different SFs. Left column: TF-tuned neuron. At the top, speed-tuning curves of a TF-tuned neuron. At the bottom, temporal-tuning curves of the same neuron. Right column: speed-tuned neuron. As for the left column, top and bottom plots represent speed- and temporal-tuning curves, respectively. (F) Contour plots for the combinations of moving gratings based on the median of the normalized peak amplitudes per fly (n = 10 flies, one repetition per fly). Black dots indicate the TFs of the maximal normalized response for each SF. White dashed lines represent the regression lines drawn through the black dots. Thin black iso-response lines are plotted every 0.1 normalized ΔF/F0. Both axes are on a log scale. (G) Average TF (mean ± SEM) of the maximal normalized peak amplitudes per spatial wavelength (front-to-back and back-to-front responses are pulled together). TF plotted against spatial wavelength yields a line with slope equal to 0 if the response is TF-tuned, while the relationship between TF and spatial wavelength is inversely proportional if the response is speed-tuned. (H) Likelihood ratio of speed-tuned to TF-tuned models of T3 responses for back-to-front and front-to-back moving gratings. The dots mark the mean across flies of an approximation of the Bayes factor and the error bars indicate SEM. Positive values mark that the speed-tuned model is more likely than the TF-tuned model, whereas negative values mark that the TF-tuned model is more likely than the speed-tuned model. (I) Spatiotemporal slope of T3 responses. The dots represent the mean of the regression coefficients across flies as in (F) and the error bars are SEM. The thresholds depicted as dashed lines indicate: 1 = perfect speed-tuning; 0 = perfect TF-tuning. (J) Top: speed-tuning curves of T3 neurons. Dots represent the median of the maximal calcium responses for different speeds. Colors of the lines indicate different spatial wavelengths. Bottom: TF-tuning curves of T3. Dots indicate the median of the maximal calcium responses for different TFs. Spatial wavelengths are colored as at the top. T3 neurons show a selectivity for speeds between 100° s–1 and 200° s–1 regardless of spatial wavelengths, whereas the TFs are more spread out depending on the spatial wavelengths.
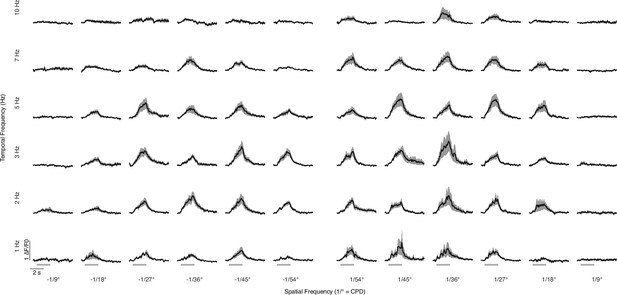
Spatiotemporal responses of T3 neurons.
Average calcium responses (mean ± SEM) to square-wave moving gratings in a single T3 neuron expressing jGCaMP7f (n = 10 flies, one repetition per fly). The time-series responses are based on the combinations of six different temporal frequencies (rows) and six different spatial frequencies (columns). Negative spatial frequencies refer to back-to-front, whereas positive values refer to front-to-back moving gratings. Light gray horizontal bars at the bottom indicate stimulus presentation.
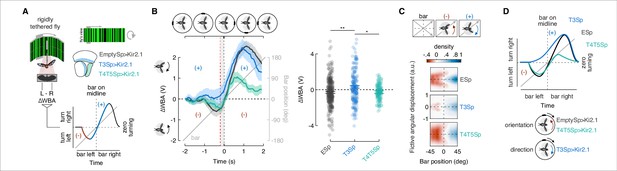
Constitutive silencing of T3 compromises the orientation response.
(A) Left: cartoon of the rigid-tether setup in which a fly is glued to a tungsten pin and placed within a surrounding LED display presenting a random pattern of bright and dark stripes. An infrared diode above the fly casts a shadow on an optical sensor that records the difference between the left and right wing beat amplitudes (ΔWBA). Right: schematic representation of the optic lobe regions where Kir2.1 channels were expressed in the three genotypes tested (data referred to T4/T5Sp>Kir2.1 flies are reproduced from Keleş et al., 2018). Bottom: schematic diagram of the experiment. A bar revolves around the fly (gray). Initially, steering is in the direction opposite to bar motion (plotted in red, negative quadrant), followed by steering in the same direction as the bar (plotted in blue, positive quadrant). Depending on the strength of each response, the steering effort may be non-zero when the bar is at zero degrees (midline). (B) Top: schematic of the bar positions over time. Left: population average time-series steering responses (mean ± SEM) in the three genotypes tested (T4/T5Sp data replotted from Keleş et al., 2018) to a motion-defined bar revolving at 90° s–1 (responses to counterclockwise [CCW] rotations were reflected and pooled with clockwise [CW] responses). Gray shaded region (between the vertical red and black dashed lines) represents a 200 ms time window before the bar crosses the fly’s visual midline (n = 44 EmptySp>Kir2.1, n = 26 T3Sp>Kir2.1, n = 22 T4/T5Sp>Kir2.1). Note that T3Sp>Kir2.1 reduces counter-directional steering, whereas T4/T5Sp>Kir2.1 reduces syn-directional steering. Right: dot plot average ΔWBA values across the 200 ms time window per trial. Dark dots indicate the mean, horizontal bars indicate SEM, and light dots represent the values in single recordings. A linear mixed model was used to fit the data and ANOVA with pairwise post-hoc comparisons using t-tests adjusted with Bonferroni method were used to compare the three genotypes (F(2, 89) = 5.83, p=0.004; EmptySp vs. T3Sp, p=0.005, Cohen’s d = –0.40;; EmptySp vs. T4/T5Sp, p=1, Cohen’s d = 0.03; T3Sp vs. T4/T5Sp, p=0.03, Cohen’s d = 0.38). (C) Heatmaps of flies’ steering effort at the population level in the three genotypes as function of the fictive fly angular displacement (arbitrary unit) and bar position (data are mirrored along the x-axis in order to get a uniform directional distribution). EmptySp and T4/T5Sp show a strong counter-directional response (red blob) while T3Sp show only a very slight counter-directional response. (D) Top: schematic summary of experimental results. T4/T5Sp>Kir2.1 reduces the syn-directional tracking effort while leaving the counter-directional (-) orientation response intact. T3Sp>Kir2.1 reduces the counter-directional steering effort, leaving the syn-directional response (+) intact, and therefore steers leftward of the bar as it crosses midline. Bottom: cartoon depicting normal orientation response in T4/T5Sp>Kir2.1 flies and compromised in T3Sp>Kir2.1 flies.
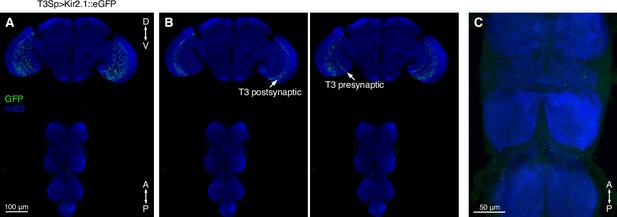
Expression pattern of T3 neurons.
(A) Maximum intensity projection (MIP) of confocal stack with ×10 objective. Brain and ventral nerve cord (VNC) from a fly expressing Kir2.1 tagged with GFP under the control of T3 split-Gal4 are shown. (B) Left: single plane (for GFP) showing T3 dendrites innervating layer 9 of the medulla (MIP for nc82). Right: single plane showing the presynaptic terminals innervating layers 2 and 3 of the lobula. (C) Maximum intensity projection of confocal stack with ×40 objective. No GFP expression is evident in the VNC.
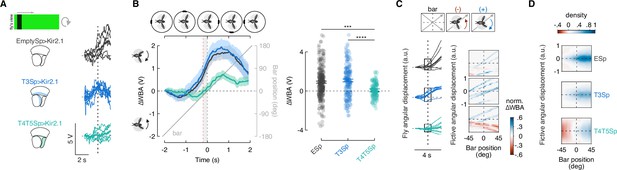
Hyperpolarization of T3 does not compromise the syn-directional response.
(A) Left: representation of the visual stimulus (dark bar revolving at 90° s–1). Middle: schematic representation of the optic lobe regions where Kir2.1 channels were expressed in the three genotypes tested. Right: single trials (3 repetitions × 2 directions) of ΔWBA responses (thin lines) to rotation of a luminance-defined bar in three representative flies (T4/T5Sp>Kir2.1 data are reproduced from Keleş et al., 2018). Thick line represents the mean (responses to counterclockwise [CCW] rotations were reflected and pooled with clockwise [CW] responses). Vertical dashed lines indicate when the bar is at the fly’s visual midline while the horizontal ones represent ΔWBA = 0. (B) Left: population average time-series steering responses (mean ± SEM) in the three genotypes tested (T4/T5Sp data replotted from Keleş et al., 2018) to a luminance-defined bar. Gray shaded region (between vertical red and black dashed lines) represents a 200 ms time window before the bar crosses the fly’s visual midline (n = 44 EmptySp>Kir2.1, n = 26 T3Sp>Kir2.1, n = 22 T4/T5Sp>Kir2.1). T4/T5Sp>Kir2.1 reduces syn-directional anticipatory steering, whereas T3Sp>Kir2.1 shows normal syn-directional steering. Right: dot plot average ΔWBA values across the 200 ms time window per trial. Dark dots indicate the mean and the horizontal bars indicate SEM (F(2, 89) = 14.48, p<0.0001; EmptySp vs. T3Sp, p=0.94, Cohen’s d = –0.12; EmptySp vs. T4/T5Sp, p<0.0001, Cohen’s d = 0.58; T3Sp vs. T4/T5Sp, p<0.0001, Cohen’s d = 0.70). (C) Left: arbitrary fly angular displacement (thin lines) resulting from the integration of ΔWBA values over time in three representative flies. Thick lines represent the mean. Right: plot of the normalized fly angular displacement within the gray shaded boxes highlighted to the left as function of the bar position. Color-code represents direction and magnitude of the normalized ΔWBA (red: counter-directional; blue: syn-directional). (D) Heatmaps of flies’ steering effort at the population level in the three genotypes as function of the fictive fly angular displacement and bar position. EmptySp and T3Sp flies show a strong syn-directional response (blue blob) while T4/T5Sp flies show a late counter-directional response and a weak syn-directional response.
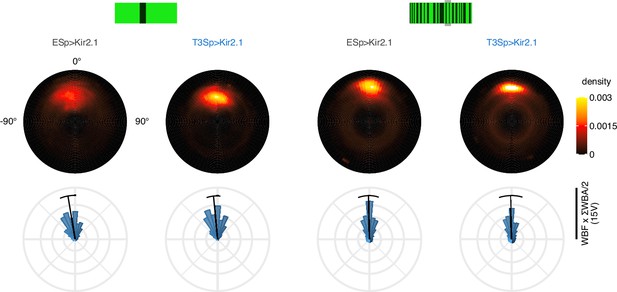
Silencing of T3 does not affect frontal bar fixation in rigid closed-loop.
Left: closed-loop fixation of a dark bar on a bright background over 60 s in EmptySp>Kir2.1 (n = 28) and T3Sp>Kir2.1 flies (n = 26). At the top, polar heatmaps of the heading where the eccentricity of the polar map represents the forward velocity (computed as WBF × ΣWBA/2). If the flies did not fly during the experiment, a blob of high density would have been confined at the center of the polar map with a strong peak at 0°, while if the flies flew at the highest velocity and spinning around during the entire experiment, a blob of high density would have been distributed around the edge of the polar map. At the bottom, polar distribution of the heading with the solid black line representing the mean with 95% bootstrap confidence interval. Each bin corresponds to 10°. Right: closed-loop fixation of a motion-defined bar on a similar textured background. Graph features are the same as on the left.
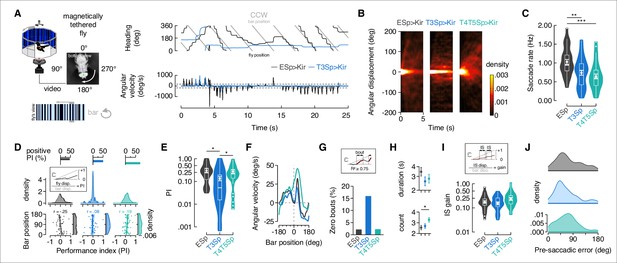
Flies with T3 hyperpolarized track a motion-defined bar poorly.
(A) Top-left: cartoon of the magnetic-tether setup in which a fly is glued to a stainless steel pin and suspended within a magnetic field in turn placed within a surrounding LED display presenting a random pattern of bright and dark stripes. Infrared diodes illuminate the fly from below and a camera captures videos of the fly’s behavior from the bottom. Bottom-left: motion-defined bar moving counterclockwise (CCW) from the fly’s perspective. Top-right: wrapped heading traces from two representative flies (black: EmptySp>Kir2.1; blue: T3Sp>Kir2.1) responding to a CCW revolving motion-defined bar at 112.5° s–1 for 25 s. Gray line represents the bar position (in this plot it relates to the EmptySp fly). Bottom-right: filtered angular velocity profiles referring to the two representative flies at the top. Horizontal dashed lines indicate the threshold for detecting saccades. (B) Heatmaps of the angular displacement (unwrapped) traveled by flies within bins of 5 s in the three genotypes during the rotation of a motion-defined bar (n = 23 EmptySp>Kir2.1, n = 22 T3Sp>Kir2.1, n = 22 T4/T5>Kir2.1). (C) Violin-box plots of the frequency of bar tracking saccades per trial in the three genotypes (black: EmptySp>Kir2.1, 1240 saccades; blue: T3Sp>Kir2.1, 851 saccades; green: T4T5Sp>Kir2.1, 708 saccades). Big white dot represents the mean, thin horizontal bars indicate SEM, and thick horizontal bar indicates the median. Small white dots on the violin tails represent outliers. A linear mixed model was used to fit the data and pairwise post-hoc comparisons using t-tests adjusted with Bonferroni method were used to compare the predictions (EmptySp vs. T3Sp, p=0.007, Cohen’s d = 1.01; EmptySp vs. T4/T5Sp, p=0.0002, Cohen’s d = 1.35; T3Sp vs. T4/T5Sp, p=0.87, Cohen’s d = 0.34). (D) Top: bar plot representing the percentage of trials in which flies showed a positive performance index (PI) per genotype. Color-code as in (C). A PI was computed based on the ratio between angular displacement of the fly and angular displacement of the bar at the end of the trial (inset). Middle: kernel density estimation of the PI distribution in the three genotypes. T3Sp>Kir2.1 flies show a high peak around zero. Bottom: scatter plot of the PI plotted against the initial bar position (at frame = 1). A regression line was drawn across the data points (thick line) and a Pearson correlation coefficient (r) computed (EmptySp, p=0.09; T3Sp, p=0.59; T4/T5Sp, p=0.22). On the right of each scatter plot, kernel density estimation of the initial bar position distribution. Colored shade around the regression line represents SEM. (E) Violin-box plots of the PI (y-axis is on a log scale) in trials with positive values (greater than 0). PI mean for clockwise (CW) and CCW revolving bars was computed and a generalized linear model (with gamma distribution and log link function) was fitted to the data (EmptySp vs. T3Sp, p=0.04, Cohen’s d = 0.80; EmptySp vs. T4/T5Sp, p=1, Cohen’s d = 0.09; T3Sp vs. T4/T5Sp p=0.05, Cohen’s d = –0.71). Graph features are as in (C). (F) Average angular velocity as a function of the bar position per genotype. T4T5Sp>Kir2.1 flies steer syn-directionally to the bar even when it is at the visual midline. (G) Bouts of bar tracking behavior were defined as periods of tracking in which the R-squared captured by linear regression was equal or greater than 0.75 (inset). Bar plot of the percentage of trials where zero bar tracking bouts were detected. (H) Top: average duration (mean ± SEM) of bar tracking bouts across flies on a log scale (EmptySp vs. T3Sp, p=0.38, Cohen’s d = 0.60; EmptySp vs. T4/T5Sp, p=0.44, Cohen’s d = 0.51; T3Sp vs. T4/T5Sp, p=1, Cohen’s d = –0.16). Bottom: average number (mean ± SEM) of bar tracking bouts per trial (EmptySp vs. T3Sp, p=1, Cohen’s d = –0.15; EmptySp vs. T4/T5Sp, p=0.04, Cohen’s d = –0.75; T3Sp vs. T4/T5Sp, p=0.17, Cohen’s d = –0.60). (I) Inter-saccadic (IS) gain was computed as the ratio between angular displacement of the fly and angular displacement of the bar during IS intervals (inset). Violin-box plots of IS gain (EmptySp vs. T3Sp, p=1, Cohen’s d = 0.27; EmptySp vs. T4/T5Sp, p=0.33, Cohen’s d = –0.63; T3Sp vs. T4/T5Sp, p=0.08, Cohen’s d = –0.90). Graph features and statistical approach are as in (E) with the only difference that a mixed-effects model was used. (J) Kernel density estimation of the pre-saccadic error angle distribution in the three genotypes. Color-code as in (A).
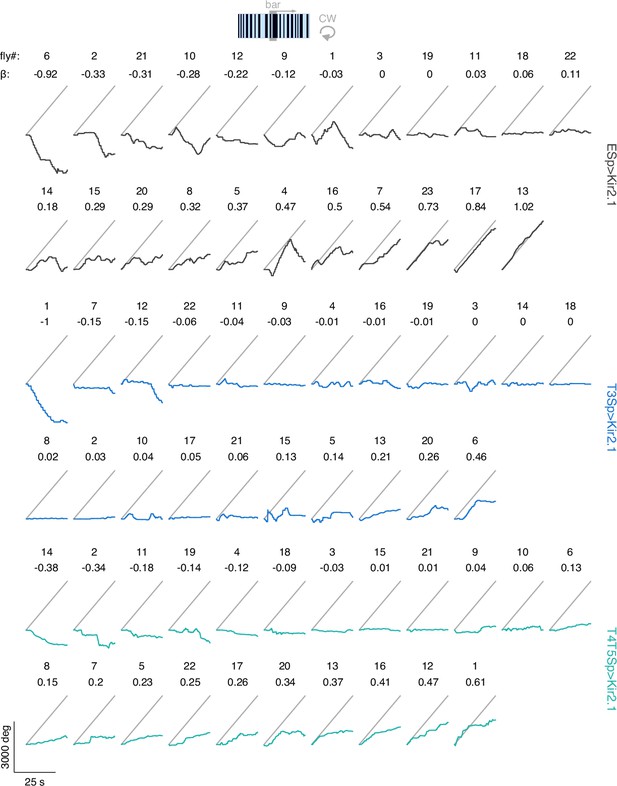
Raw unwrapped angular distance traveled by the flies during bar tracking.
The traces represent the responses per genotype (black: EmptySp>Kir2.1; blue: T3Sp>Kir2.1; green: T4T5Sp>Kir2.1) to a clockwise (CW) revolving motion-defined bar at 112.5° s–1 for 25 s. Gray line represents the bar angular displacement. The responses within genotype are sorted by the regression coefficient (β) of a linear fitting to the data.
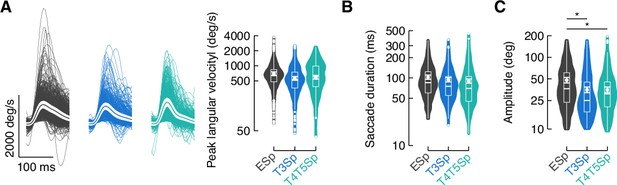
Saccade dynamics during bar tracking.
(A) Left: average time series of the angular velocity in the three genotypes. Thin lines represent single saccades. Thick line represents the mean and the white shade around the mean represents SEM. Right: violin-box plots of the peak angular velocity during saccades (y-axis is on a log scale). A generalized linear mixed model was used to fit the data (residuals were fitted with a gamma distribution and a log link function) and pairwise post-hoc comparisons using t-tests adjusted with Bonferroni method were used to compare the levels of the fixed effects (EmptySp vs. T3Sp, p=0.23, Cohen’s d = 0.43; EmptySp vs. T4/T5Sp, p=0.56, Cohen’s d = 0.32; T3Sp vs. T4/T5Sp, p=1, Cohen’s d = –0.11). Graph features are as in Figure 4C. (B) Violin-box plots of saccade duration (EmptySp vs. T3Sp, p=1, Cohen’s d = 0.17; EmptySp vs. T4/T5Sp, p=0.58, Cohen’s d = 0.26; T3Sp vs. T4/T5Sp p=1, Cohen’s d = 0.09). Graph features and statistical approach are as in (A). (C) Violin-box plots of saccade amplitude (EmptySp vs. T3Sp, p=0.04, Cohen’s d = 0.52; EmptySp vs. T4/T5Sp, p=0.04, Cohen’s d = 0.51; T3Sp vs. T4/T5Sp, p=1, Cohen’s d = –0.004). Graph features and statistical approach are as in (A).
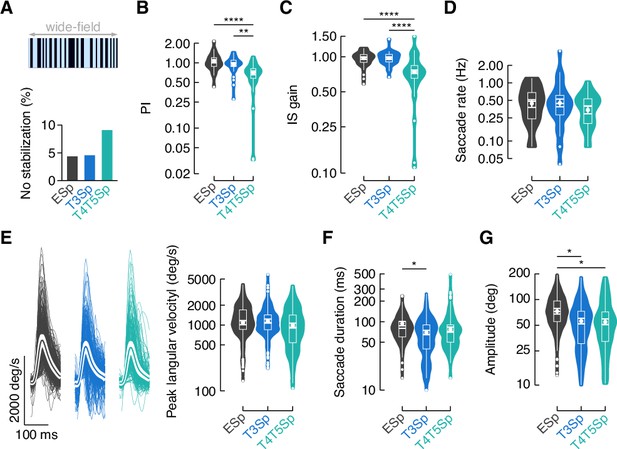
T3 silencing does not affect the response to the rotation of a wide-field panorama.
(A) Percentage of trials in which flies did not stabilize the rotating wide-field pattern of random dark and bright stripes. A performance index (PI) was computed as in Figure 4D. Bar plot represents the percentage of trials per genotype where the PI was equal of less than 0. (B) Violin-box plots of the PI in trials with positive values (y-axis is on a log scale). PI mean for clockwise (CW) and counterclockwise (CCW) rotating wide-field pattern was computed and a generalized linear model (with Gaussian distribution and log link function) was used to fit the data (EmptySp vs. T3Sp, p=0.29, Cohen’s d = 0.50; EmptySp vs. T4/T5Sp, p<0.0001, Cohen’s d = 1.89; T3Sp vs. T4/T5Sp p=0.001, Cohen’s d = 1.39). Graph features are as in Figure 4C. (C) Violin-box plots of inter-saccadic (IS) gain defined as in Figure 4I (EmptySp vs. T3Sp, p=1, Cohen’s d = –0.006; EmptySp vs. T4/T5Sp, p<0.0001, Cohen’s d = 2.31; T3Sp vs. T4/T5Sp, p<0.0001, Cohen’s d = 2.32). Graph features and statistical approach are as in (B). (D) Violin-box plots of the frequency of optomotor saccades per trial in the three genotypes (y-axis is on a log scale). A generalized linear mixed model (with gamma distribution and log link function) was used to fit the data (EmptySp vs. T3Sp, p=1, Cohen’s d = –0.04; EmptySp vs. T4/T5Sp, p=0.69, Cohen’s d = 0.43; T3Sp vs. T4/T5Sp, p=0.57, Cohen’s d = 0.47). Graph features are as in Figure 4C. (E) Left: average time series of the angular velocity during optomotor saccades in the three genotypes. Thin lines represent single saccades. Thick line represents the mean and the white shade around the mean represents SEM. Right: violin-box plots of the peak angular velocity during saccades (EmptySp vs. T3Sp, p=1, Cohen’s d = –0.11; EmptySp vs. T4/T5Sp, p=1, Cohen’s d = 0.19; T3Sp vs. T4/T5Sp, p=0.47, Cohen’s d = 0.30). Graph features and statistical approach are as (D). (F) Violin-box plots of saccade duration (EmptySp vs. T3Sp, p=0.01, Cohen’s d = 0.65; EmptySp vs. T4/T5Sp, p=0.21, Cohen’s d = 0.40; T3Sp vs. T4/T5Sp p=0.85, Cohen’s d = –0.24). Graph features and statistical approach are as in (D). (G) Violin-box plots of saccade amplitude (EmptySp vs. T3Sp, p=0.04, Cohen’s d = 0.60; EmptySp vs. T4/T5Sp, p=0.02, Cohen’s d = 0.64; T3Sp vs. T4/T5Sp, p=1, Cohen’s d = 0.04). Graph features and statistical approach are as in (D).
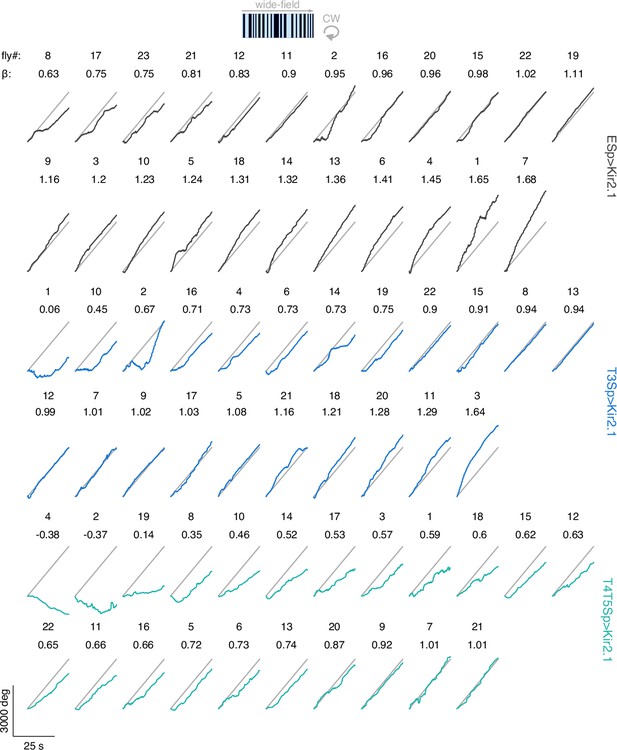
Raw unwrapped angular distance traveled by the flies during wide-field stabilization.
The traces represent the responses per genotype (black: EmptySp>Kir2.1; blue: T3Sp>Kir2.1; green: T4T5Sp>Kir2.1) to a clockwise (CW) rotating wide-field panorama at 112.5° s–1 for 25 s. Gray line represents the angular displacement traveled by the panorama. The responses within genotype are sorted by the regression coefficient (β) of a linear fitting to the data.
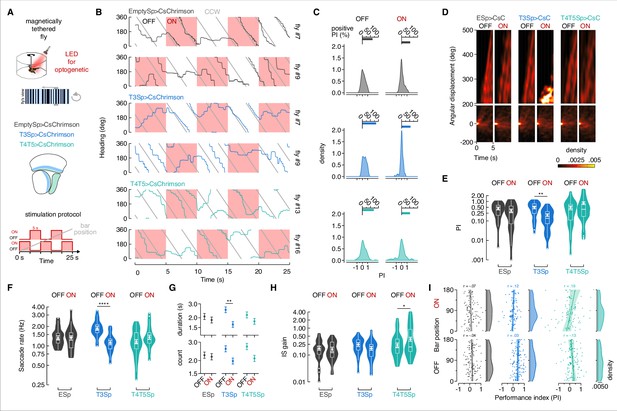
T3 decreases bar tracking behavior during non-selective depolarization.
(A) Top: cartoon of the magnetic-tether setup implemented with an optogenetic LED for neural stimulation. Middle: genotypes tested and schematic representation of the optic lobe regions innervated by T3 and T4/T5. Bottom: schematic representation of the stimulation protocol: repetition of 5 s optogenetic LED OFF followed by 5 s LED ON for 25 s. ON/OFF starting was randomly selected. (B) Wrapped heading traces from six representative flies (black: EmptySp>CsChrimson; blue: T3Sp>CsChrimson; green: T4/T5Sp>CsChrimson) responding to a counterclockwise (CCW) revolving motion-defined bar as in Figure 4A. Red shaded regions represent periods of LED ON. EmptySp>CsChrimson and T4/T5EmptySp>CsChrimson flies were slightly affected by the LED ON, while T3Sp>CsChrimson flies stop chasing the bar. (C) Top: bar plot representing the percentage of trials in which flies showed a positive performance index (PI) during periods of LED OFF and ON in the three genotypes. A PI was computed as in Figure 4D. Color-code as in (B). Bottom: kernel density estimation of the PI distribution per genotype and LED condition. T3Sp>CsChrimson flies show a high peak around zero when the LED was ON. (D) Heatmaps of the angular displacement (unwrapped) traveled by flies within bins of 5 s in the three genotypes during the rotation of a motion-defined bar (n = 20 EmptySp>CsChrimson, n = 22 T3Sp>CsChrimson, n = 21 T4/T5>CsChrimson). The map was divided in two windows to highlight the behavior of flies traveling an angular displacement greater than 200°. T3Sp>CsChrimson flies remain stuck during the optogenetic stimulation periods. (E) Violin-box plots of the PI in trials with positive values (y-axis is on a log scale). Big white dot represents the mean, thin horizontal bars indicate SEM, and thick horizontal bar indicates the median. Small white dots on the violin tails represent outliers. PI mean for clockwise (CW) and CCW revolving bars was computed and a generalized linear model (with gamma distribution and log link function) was fitted to the data (EmptySp OFF vs. ON, p=1, Cohen’s d = 0.33; T3Sp OFF vs. ON, p=0.002, Cohen’s d = 1.21; T4/T5Sp OFF vs. ON, p=0.53, Cohen’s d = –0.67). (F) Violin-box plots of the frequency of bar tracking saccades per trial during periods of LED OFF and ON (y-axis is on a log scale). A generalized linear mixed model was used to fit the data (residuals were fitted with a gamma distribution and a log link function) and pairwise post-hoc comparisons using t-tests adjusted with Bonferroni method were used to compare the predictions (EmptySp OFF vs. ON, p=1, Cohen’s d = 0.01; T3Sp OFF vs. ON, p<0.0001, Cohen’s d = 1.70; T4/T5Sp OFF vs. ON, p=0.24, Cohen’s d = –0.52). Graph features are as in (E). (G) Bouts of bar tracking behavior (defined as in Figure 4G) during periods of LED OFF and ON. Top: average duration (mean ± SEM) of bar tracking bouts across flies on a log scale (EmptySp OFF vs. ON, p=1, Cohen’s d = 0.26; T3Sp OFF vs. ON, p=0.007, Cohen’s d = 1.09; T4/T5Sp OFF vs. ON, p=1, Cohen’s d = 0.48). Bottom: average number (mean ± SEM) of bar tracking bouts during OFF or ON periods on a log scale (EmptySp OFF vs. ON, p=1, Cohen’s d = 0.10; T3Sp OFF vs. ON, p=0.08, Cohen’s d = 0.83; T4/T5Sp OFF vs. ON, p=0.13, Cohen’s d = 0.81). (H) Violin-box plots of inter-saccadic (IS) gain (defined as in Figure 4I) during periods of LED OFF and ON (EmptySp OFF vs. ON, p=1, Cohen’s d = 0.12; T3Sp OFF vs. ON, p=0.26, Cohen’s d = 0.49; T4/T5Sp OFF vs. ON, p=0.01, Cohen’s d = –0.75). Graph features and statistical approach are as in (F). (I) Scatter plot of the PI plotted against the bar position at the beginning of each 5 s period in ON and OFF conditions. A regression line was drawn across the data points (thick line) and a Pearson correlation coefficient (r) computed (EmptySp OFF, p=.068; EmptySp ON, p=0.48; T3Sp OFF, p=0.73; T3Sp ON, p=0.20; T4/T5Sp OFF, p=0.19; T4/T5Sp ON, p=0.06). On the right of each scatter plot, kernel density estimation of the initial bar position distribution. Colored shade around the regression line represents SEM.
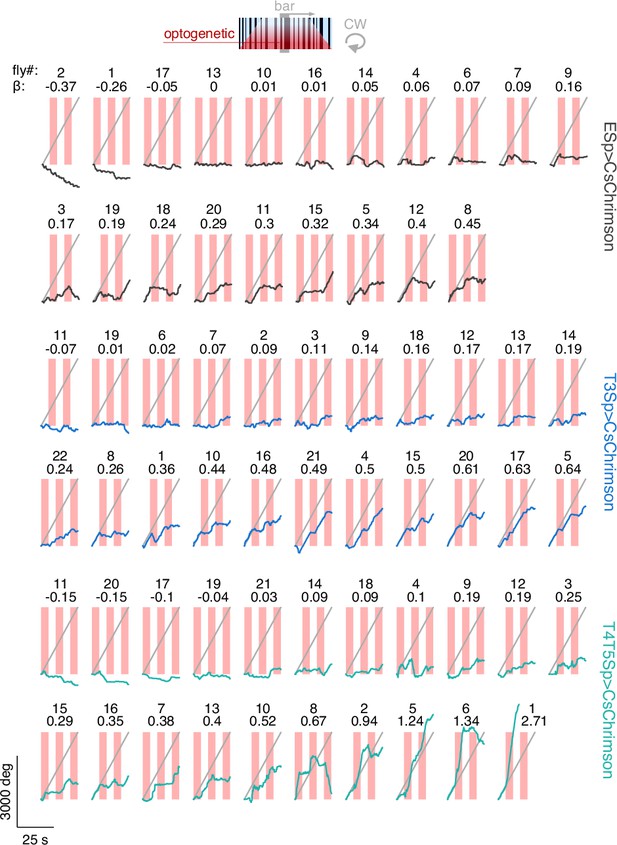
Raw unwrapped angular distance traveled by the flies during bar tracking and optogenetic stimulation.
The traces represent the responses per genotype (black: EmptySp>CsChrimson; blue: T3Sp>CsChrimson; green: T4T5Sp>CsChrimson) to a clockwise (CW) revolving motion-defined bar at 112.5° s–1 for 25 s. Gray line represents the bar angular displacement. The responses within genotype are sorted by the regression coefficient (β) of a linear fitting to the data.
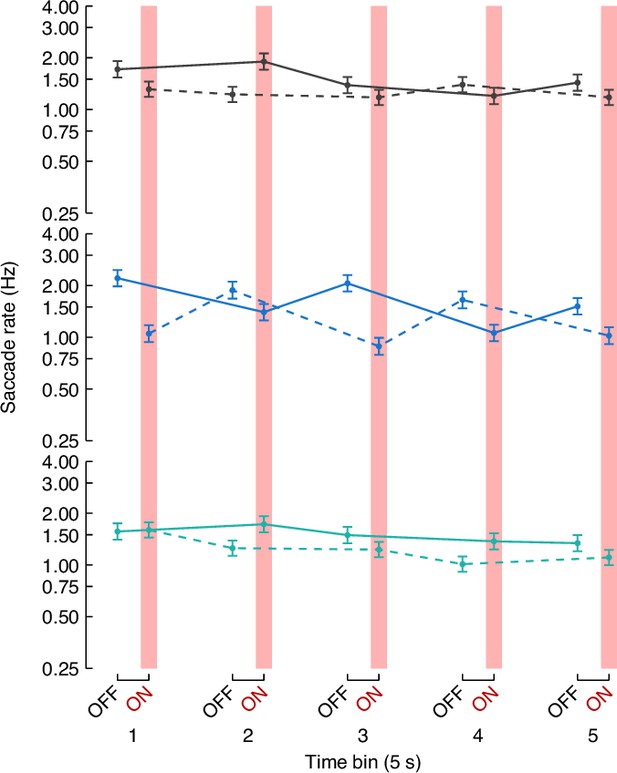
Frequency of bar tracking saccades per bin of LED OFF or ON (five progressive bins of 5 s each).
Colored dot represents the mean and error bar indicates SEM (y-axis is on a log scale). Lines connected the dots represents saccade frequencies from flies exposed to same sequence of optogenetic stimulation (solid line: OFF-ON-OFF-ON-OFF; dashed line: ON-OFF-ON-OFF-ON). T3Sp>CsChrimson flies starting with LED OFF show already an increased saccade rate that decreases when the LED turns ON.
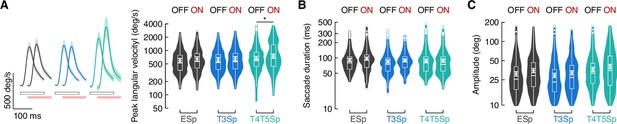
Saccade dynamics during bar tracking and optogenetic stimulation.
(A) Left: average angular velocity (mean ± SEM) of saccades. Light gray and red horizontal bars at the bottom indicate LED OFF and ON, respectively. Right: violin-box plots of saccade peak angular velocity during periods of LED OFF and ON (y-axis is on a log scale). A generalized linear mixed model (with gamma distribution and log link function) was fitted to the mean values per fly and pairwise post-hoc comparisons using t-tests adjusted with Bonferroni method were used to compare the levels of the fixed effect (EmptySp OFF vs. ON, p=.64, Cohen’s d = –0.44; T3Sp OFF vs. ON, p=1, Cohen’s d = –0.21; T4/T5Sp OFF vs. ON, p=0.02, Cohen’s d = –0.69). Graph features are as in Figure 5E. (B) Violin-box plots of saccade duration during periods of LED OFF and ON (EmptySp OFF vs. ON, p=0.99, Cohen’s d = –0.43; T3Sp OFF vs. ON, p=1, Cohen’s d = –0.34; T4/T5Sp OFF vs. ON, p=1, Cohen’s d = 0.02). Null model was the best model tested. Graph features and statistical approach are as in (A). (C) Violin-box plots of saccade amplitude during periods of LED OFF and ON (EmptySp OFF vs. ON, p=0.16, Cohen’s d = –0.65; T3Sp OFF vs. ON, p=0.66, Cohen’s d = –0.49; T4/T5Sp OFF vs. ON, p=0.39, Cohen’s d = –0.56). Graph features and statistical approach are as in (A).
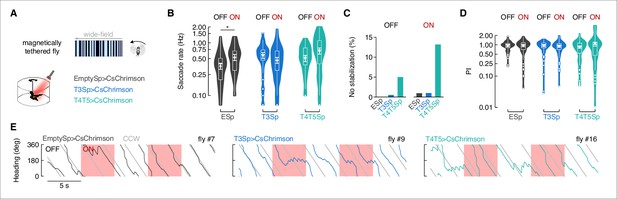
Depolarization of T3 does not affect the stabilization of a rotating wide-field panorama.
(A) Top: representation of the wide-field pattern consisting of bright and dark random stripes as seen by the fly. Bottom: cartoon of the magnetic-tether setup implemented for optogenetic stimulation and genotypes tested (n = 20 EmptySp>CsChrimson, n = 22 T3Sp>CsChrimson, n = 21 T4/T5>CsChrimson). (B) Violin-box plots of the frequency of optomotor saccades during periods of LED OFF and ON (y-axis is on a log scale). Big white dot represents the mean, thin horizontal bars indicate SEM, and thick horizontal bar indicates the median. Small white dots on the violin tails represent outliers. A generalized linear mixed model was used to fit the data (residuals were fitted with a gamma distribution and a log link function) and pairwise post-hoc comparisons using t-tests adjusted with Bonferroni method were used to compare the predictions (EmptySp OFF vs. ON, p=0.02, Cohen’s d = –0.73; T3Sp OFF vs. ON, p=0.94, Cohen’s d = 0.41; T4/T5Sp OFF vs. ON, p=0.09, Cohen’s d = –0.62). (C) Percentage of trials in which flies did not stabilize the rotating wide-field pattern during periods of LED OFF and ON. A performance index (PI) was computed as in Figure 4D. Bar plot represents the percentage of trials per genotype where the PI was equal of less than 0. (D) Violin-box plots of the PI in trials with positive value (EmptySp OFF vs. ON, p=1, Cohen’s d = –0.01; T3Sp OFF vs. ON, p=1, Cohen’s d = 0.12; T4/T5Sp OFF vs. ON, p=0.36, Cohen’s d = –0.65). Null model was the best model tested. Graph features and statistical approach are as in Figure 5E. (E) Wrapped heading traces from three representative flies (black: EmptySp>CsChrimson; blue: T3Sp>CsChrimson; green: T4/T5Sp>CsChrimson) responding to a counterclockwise (CCW) rotating wide-field panorama during optogenetic stimulation. Red shaded regions represent periods of LED ON.
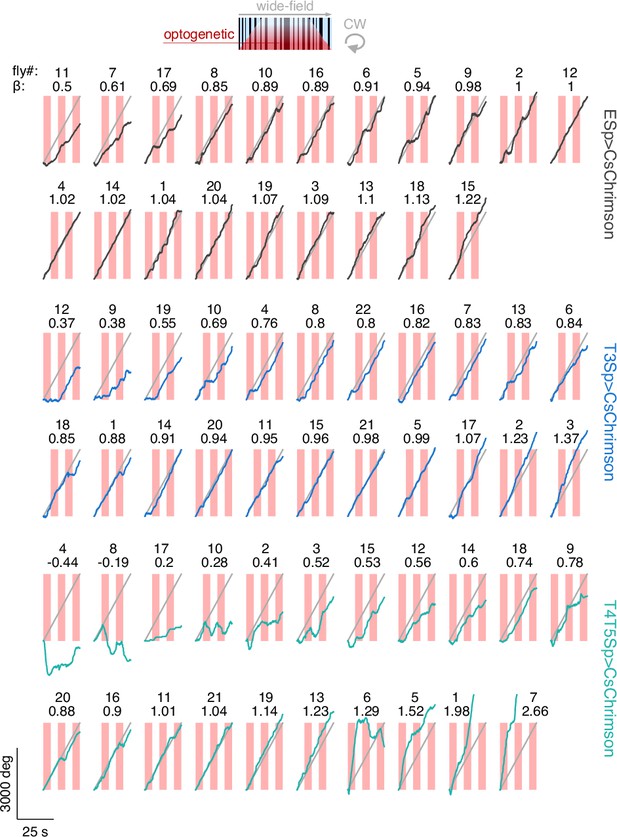
Raw unwrapped angular distance traveled by the flies during wide-field stabilization and optogenetic stimulation.
The traces represent the responses per genotype (black: EmptySp>CsChrimson; blue: T3Sp>CsChrimson; green: T4T5Sp>CsChrimson) to a clockwise (CW) rotating wide-field panorama at 112.5° s–1 for 25 s. Gray line represents the angular displacement traveled by the panorama. The responses within genotype are sorted by the regression coefficient (β) of a linear fitting to the data.

Saccade dynamics during wide-field stabilization and optogenetic stimulation.
(A) Left: average angular velocity (mean ± SEM) of saccades. Light gray and red horizontal bars at the bottom indicate LED OFF and ON respectively. Right: violin-box plots of saccade peak angular velocity during periods of LED OFF and ON (y-axis is on a log scale). A generalized linear mixed model (with gamma distribution and log link function) was fitted to the data and pairwise post-hoc comparisons using t-tests adjusted with Bonferroni method were used to compare the levels of the fixed effect (EmptySp OFF vs. ON, p=0.19, Cohen’s d = –0.50; T3Sp OFF vs. ON, p=1, Cohen’s d = 0.30; T4/T5Sp OFF vs. ON, p=0.37, Cohen’s d = –0.45). Model with only genotype as fixed effect was better than the one with an interaction between genotype and LED (BF01 = 2.24). Graph features are as in Figure 6B. (B) Violin-box plots of saccade duration during periods of LED OFF and ON (EmptySp OFF vs. ON, p=0.32, Cohen’s d = 0.47; T3Sp OFF vs. ON, p=1, Cohen’s d = 0.02; T4/T5Sp OFF vs. ON, p=0.02, Cohen’s d = 0.65). Graph features and statistical approach are as in (A). (C) Violin-box plots of saccade amplitude during periods of LED OFF and ON (EmptySp OFF vs. ON, p=1, Cohen’s d = 0.01; T3Sp OFF vs. ON, p=1, Cohen’s d = 0.06; T4/T5Sp OFF vs. ON, p=1, Cohen’s d = 0.06). Graph features and statistical approach are as in (A).

Saccade rate and performance index in bar tracking and wide-field stabilization in flies expressing CsChrimson.
(A) Violin-box plots of the frequency of bar tracking saccades per trial in the three genotypes (black: n = 19 EmptySp>CsChrimson; blue: n = 18 T3Sp>CsChrimson; green: n = 19 T4T5Sp>CsChrimson). Big white dot represents the mean, thin horizontal bars indicate SEM, and thick horizontal bar indicates the median. Small white dots on the violin tails represent outliers. A linear mixed model was used to fit the data and pairwise post-hoc comparisons using t-tests adjusted with Bonferroni method were used to compare the predictions (EmptySp vs. T3Sp, p=.005, Cohen’s d = –1.07; EmptySp vs. T4/T5Sp, p=0.007, Cohen’s d = –0.97; T3Sp vs. T4/T5Sp, p=1, Cohen’s d = 0.10). (B) Violin-box plots of the PI (y-axis is on a log scale) in trials with positive values (greater than 0). PI mean for clockwise (CW) and counterclockwise (CCW) revolving bars was computed and a generalized linear model (with gamma distribution and log link function) was fitted to the data (EmptySp vs. T3Sp, p=0.18, Cohen’s d = –0.56; EmptySp vs. T4/T5Sp, p=0.60, Cohen’s d = –0.38; T3Sp vs. T4/T5Sp p=1, Cohen’s d = 0.19). Graph features are as in (A). (C) Violin-box plots of the frequency of optomotor saccades per trial in the three genotypes (y-axis is on a log scale). A generalized linear mixed model (with gamma distribution and log link function) was used to fit the data (EmptySp vs. T3Sp, p=1, Cohen’s d = –0.46; EmptySp vs. T4/T5Sp, p=1, Cohen’s d = –0.22; T3Sp vs. T4/T5Sp, p=1, Cohen’s d = 0.24). Graph features are as in (A). (D) Violin-box plots of the PI in trials with positive values (y-axis is on a log scale). PI mean for CW and CCW rotating wide-field pattern was computed and a generalized linear model (with Gaussian distribution and log link function) was used to fit the data (EmptySp vs. T3Sp, p=1, Cohen’s d = 0.32; EmptySp vs. T4/T5Sp, p=0.18, Cohen’s d = 0.64; T3Sp vs. T4/T5Sp p=1, Cohen’s d = 0.32). Graph features are as in (A).
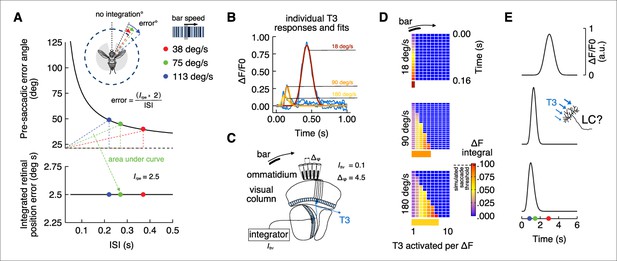
Integrate-and-fire model physiologically inspired by T3 calcium dynamics.
(A) Top: pre-saccadic error angles modeled by using the inverse function of the integrated retinal position error (Mongeau and Frye, 2017). Knowing the inter-saccadic intervals (ISI) from previous behavioral experiments as a function of the bar speed and the value of the integrated retinal position error (Irpr), we can compute the pre-saccadic error angle as a function of ISI. Modeled error angles are very close to the average errors from behavioral experiments (ISI = 0.22 s, error = 49°; ISI = 0.27 s, error = 45°; ISI = 0.37 s, error = 40°). Dashed black line represents the offset from which the integration of the bar position over time starts (in this model ~26° from visual midline). The area of the triangles defined by the dashed colors lines is constant and represents the Irpr. Bottom: integrated retinal position error (Irpr = 2.5° s) derived from previous experiments (Mongeau and Frye, 2017) that represents the multiplication of pre-saccadic error angle by ISI. (B) Average calcium responses of T3 neurons (blue) to a moving motion-defined bar at three different speeds (data from Figure 1I). These responses were fitted using nonlinear regression analysis (yellow: 180° s–1; orange: 90° s–1; red: 18° s–1). Areas under the fitted curves were computed (ICa2+). (C) Schematic representation of the fly visual lobe with a 1D organization of T3 neurons that are sequentially activated by a moving bar across the retina. A T3 neuron is present in each column and the interommatidial angle (Δφ) is ~4.5°. We set an arbitrary integrated threshold (Ithr = 0.1) that a downstream partner of T3 would use to trigger a saccade. (D) Space-time plots of Ithr accumulating over time across cells depending on bar speed (ICa2+ is based on the physiology, Δφ is known and Ithr is arbitrary). Histogram at the bottom indicates the number of T3 cells that in a 1D space would be required to trigger a saccade according to the model. (E) Simulated responses by speed of a hypothetical integrator downstream of T3 neurons. LC17 cells might play this role.
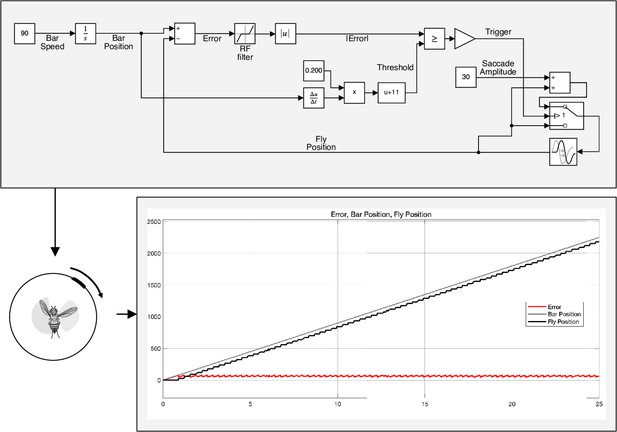
Control model for triggering saccades.
Top: Simulink (MATLAB) implementation of the physiologically-inspired model in Figure 7. The bar speed (90° s–1) is integrated over a quite narrow time window (~200 ms) and the integration starts with a spatial offset with respect to the visual midline (~11°). This means that T3 neurons might encode the bar speed and a downstream partner might integrate this information over a selective amount of time, encoding the bar position and triggering a saccade when the amount of calcium reaches a specific threshold. In this model, saccade amplitudes are considered a fixed parameter (30°) but in an alternative version they could be easily tuned to the bar speed. Bottom: simulation of the fly behavior (angular position) in a bar tracking task according to the control model.
Videos
Calcium imaging recording from T3 dendrites.
T3 neurons expressing GCaMP6f respond to a back-to-front moving bright bar at 90° s–1.
EmpySp>Kir2.1 fly presented with a revolving motion-defined bar.
Left: bottom view of a single fly within an animated cartoon of the surrounding display presenting a motion-defined bar revolving for 25 s at 112.5° s–1. Right-top: fly heading (white) and bar position (gray). Right-bottom: error (red) between bar position and fly heading. EmptySp flies track the bar by using a saccade-and-fixation strategy. Original video recorded at 200 fps.
T3Sp>Kir2.1 fly presented with a revolving motion-defined bar.
Conditions identical to Video 2. T3Sp flies do not track the bar.
T4/T5Sp>Kir2.1 fly presented with a revolving motion-defined bar.
Conditions identical to Video 2. T4/T5Sp flies track the bar with some defects in gaze stabilization.
Tables
Origin of reagents used in this study.
Reagent type | Source | Identifier |
---|---|---|
D. melanogaster: Empty-SplitGal4 [p65ADZp.Uw(attP40); ZpGDBD.Uw(attP2)] | Bloomington Drosophila Stock Center | BDSC #79603 |
D. melanogaster: T3-SplitGal4 [VT002055-p65ADZp(attP40); R65B04- ZpGDBD(attP2)] | Keleş et al., 2020 | N/A |
D. melanogaster: T2a-SplitGal4 [VT012791-p65ADZp(attP40); R47E02- ZpGDBD(attP2)] | Keleş et al., 2020 | N/A |
D. melanogaster: T4/T5-SplitGal4 [R59E08-p65.AD(attP40); R42F06-Gal4.DBD(attP2)] | G.Rubin | N/A |
D. melanogaster: 20XUAS-IVS-GCaMP6f(VK00005) | Bloomington Drosophila Stock Center | BDSC #52869 |
D. melanogaster: 20XUAS-IVS-jGCaMP7f(VK00005) | Bloomington Drosophila Stock Center | BDSC #79031 |
D. melanogaster: 10XUAS-IVS-eGFP-Kir2.1(attP2) | von Reyn et al., 2017 | N/A |
D. melanogaster: 20xUAS-Chrimson::tdTomato(VK00005) | D.Anderson | N/A |
Antibody: anti-GFP (chicken polyclonal) | Abcam | Cat#: ab13970; RRID:AB_300798 |
Antibody: anti-Brp (mouse monoclonal) | Developmental Studies Hybridoma Bank | Cat#: nc82; RRID:AB_2314866 |
Antibody: anti-chicken, Alexa Fluor 488 (goat polyclonal) | Thermo Fisher Scientific | Cat#: A11039; RRID:AB_2534062 |
Antibody: anti-mouse, Alexa Fluor 647 (goat polyclonal) | Thermo Fisher Scientific | Cat#: A21236; RRID:AB_2535804 |
Genotypes and experimental parameters used in each figure.
Description | Genotype | Experiment | Figure # | Flies # | Repetition # |
---|---|---|---|---|---|
T3Sp>GCaMP6f | w-/+; VT002055-AD/+; R65B04-DBD/20xUAS-GCaMP6f | ON and OFF moving bars (9° width) in two directions (front-to-back and back-to-front) at three different speeds (18° s–1, 90° s–1, 180° s–1) | Figure 1D and J; Figure 1—figure supplement 1 | 11 | 3 |
T3Sp>GCaMP6f | w-/+; VT002055-AD/+; R65B04-DBD/20xUAS-GCaMP6f | Single-pixel OFF bar (2.25° width) moving in four different directions (upward, downward, leftward, and rightward) at 18° s–1 | Figure 1G and H | 5 | 1 |
T3Sp>GCaMP6f | w-/+; VT002055-AD/+; R65B04-DBD/20xUAS-GCaMP6f | Motion-defined bars (9° width) moving in two directions (front-to-back and back-to-front) at three different speeds (18° s–1, 90° s–1, 180° s–1) | Figure 1I | 11 | 3 |
T2aSp>GCaMP6f | w-/+; VT012791-AD/+; R47E02-DBD/20xUAS-GCaMP6f | Single-pixel OFF bar (2.25° width) moving in four different directions (upward, downward, leftward, and rightward) at 18° s–1 | Figure 1—figure supplement 2D and E | 4 | 1 |
T2aSp>GCaMP6f | w-/+; VT012791-AD/+; R47E02-DBD/20xUAS-GCaMP6f | ON, OFF, and motion-defined moving bars (9° width) in two directions (front-to-back and back-to-front) at three different speeds (18° s–1, 90° s–1, 180° s–1) | Figure 1—figure supplement 2F and G | 9 | 3 |
T2aSp>GCaMP6f | w-/+; VT012791-AD/+; R47E02-DBD/20xUAS-GCaMP6f | Gratings patterns of λ = 18° and λ = 36° moving at two different temporal frequencies (1 Hz and 2 Hz) in two directions (front-to-back and back-to-front) | Figure 1—figure supplement 2H | 9 | 3 |
T3Sp>GCaMP6f | w-/+; VT002055-AD/+; R65B04-DBD/20xUAS-GCaMP6f | Gratings patterns of λ = 18° and λ=36° moving at three different temporal frequencies (1 Hz, 2 Hz, and 5 Hz) in two directions (front-to-back and back-to-front) | Figure 2A–C | 11 | 3 |
T3Sp>jGCaMP7f | w-/+; VT002055-AD/+; R65B04-DBD/20xUAS-jGCaMP7f | Gratings patterns of six different spatial frequencies (λ = 9°, 18°, 27°, 36°, 45°, 54°) moving at six different temporal frequencies (Hz = 1, 2, 3, 5, 7, 10) in two directions (front-to-back and back-to-front) | Figure 2F–J; Figure 2—figure supplement 1 | 10 | 1 |
EmptySp>Kir2.1 | w-/+; empty-AD/+; empty-DBD/10xUAS-Kir2.1 | Motion-defined and dark bars (18° width) moving at 90° s–1 CW and CCW and starting from behind the fly | Figure 3B and C; Figure 3—figure supplement 2B and D | 44 | 3 |
T3Sp>Kir2.1 | w-/+; VT002055-AD/+; R65B04-DBD/10xUAS-Kir2.1 | Motion-defined and dark bars (18° width) moving at 90° s–1 CW and CCW and starting from behind the fly | Figure 3B and C; Figure 3—figure supplement 2B and D | 26 | 3 |
T4/T5Sp>Kir2.1 | w-/+; R59E08-AD/+; R42F06-DBD/10xUAS-Kir2.1 | Motion-defined and dark bars (18° width) moving at 90° s–1 CW and CCW and starting from behind the fly | Figure 3B and C; Figure 3—figure supplement 2B and D | 22 | 3 |
EmptySp>Kir2.1 | w-/+; empty-AD/+; empty-DBD/10xUAS-Kir2.1 | Dark and motion-defined bars in closed-loop for 60 s | Figure 3—figure supplement 3 | 28 | 1 |
T3Sp>Kir2.1 | w-/+; VT002055-AD/+; R65B04-DBD/10xUAS-Kir2.1 | Dark and motion-defined bars in closed-loop for 60 s | Figure 3—figure supplement 3 | 26 | 1 |
EmptySp>Kir2.1 | w-/+; empty-AD/+; empty-DBD/10xUAS-Kir2.1 | Motion-defined bar (18° width) and large-field panorama moving at 112.5° s–1 CW and CCW (bar started at random locations) | Figure 4B–J; Figure 4—figure supplement 2; Figure 4—figure supplement 3 | 23 | 1 |
T3Sp>Kir2.1 | w-/+; VT002055-AD/+; R65B04-DBD/10xUAS-Kir2.1 | Motion-defined bar (18° width) and large-field panorama moving at 112.5° s–1 CW and CCW (bar started at random locations) | Figure 4B–J; Figure 4—figure supplement 2; Figure 4—figure supplement 3 | 22 | 1 |
T4/T5Sp>Kir2.1 | w-/+; R59E08-AD/+; R42F06-DBD/10xUAS-Kir2.1 | Motion-defined bar (18° width) and large-field panorama moving at 112.5° s–1 CW and CCW (bar started at random locations) | Figure 4B–J; Figure 4—figure supplement 2; Figure 4—figure supplement 3 | 22 | 1 |
EmptySp>CsChrimson | w-/+; empty-AD/+; empty-DBD/20xUAS-Chrimson | Motion-defined bar (18° width) and large-field panorama moving at 112.5° s–1 CW and CCW (bar started at random locations) during optogenetic stimulation | Figure 5C–I; Figure 5—figure supplement 2; Figure 5—figure supplement 3; Figure 6B–D; Figure 6—figure supplement 2 | 20 | 1 |
T3Sp>CsChrimson | w-/+; VT002055-AD/+; R65B04-DBD/20xUAS-Chrimson | Motion-defined bar (18° width) and large-field panorama moving at 112.5° s–1 CW and CCW (bar started at random locations) during optogenetic stimulation | Figure 5C–I; Figure 5—figure supplement 2; Figure 5—figure supplement 3; Figure 6B–D; Figure 6—figure supplement 2 | 22 | 1 |
T4/T5Sp>CsChrimson | w-/+; R59E08-AD/+; R42F06-DBD/20xUAS-Chrimson | Motion-defined bar (18° width) and large-field panorama moving at 112.5° s–1 CW and CCW (bar started at random locations) during optogenetic stimulation | Figure 5C–I; Figure 5—figure supplement 2; Figure 5—figure supplement 3; Figure 6B–D; Figure 6—figure supplement 2 | 21 | 1 |
EmptySp>CsChrimson | w-/+; empty-AD/+; empty-DBD/20xUAS-Chrimson | Motion-defined bar (18° width) and large-field panorama moving at 112.5° s–1 CW and CCW (bar started at random locations) | Figure 6—figure supplement 3 | 19 | 1 |
T3Sp>CsChrimson | w-/+; VT002055-AD/+; R65B04-DBD/20xUAS-Chrimson | Motion-defined bar (18° width) and large-field panorama moving at 112.5° s–1 CW and CCW (bar started at random locations) | Figure 6—figure supplement 3 | 18 | 1 |
T4/T5Sp>CsChrimson | w-/+; R59E08-AD/+; R42F06-DBD/20xUAS-Chrimson | Motion-defined bar (18° width) and large-field panorama moving at 112.5° s–1 CW and CCW (bar started at random locations) | Figure 6—figure supplement 3 | 19 | 1 |
-
CW: clockwise; CCW: counterclockwise.