Carm1-arginine methylation of the transcription factor C/EBPα regulates transdifferentiation velocity
Figures
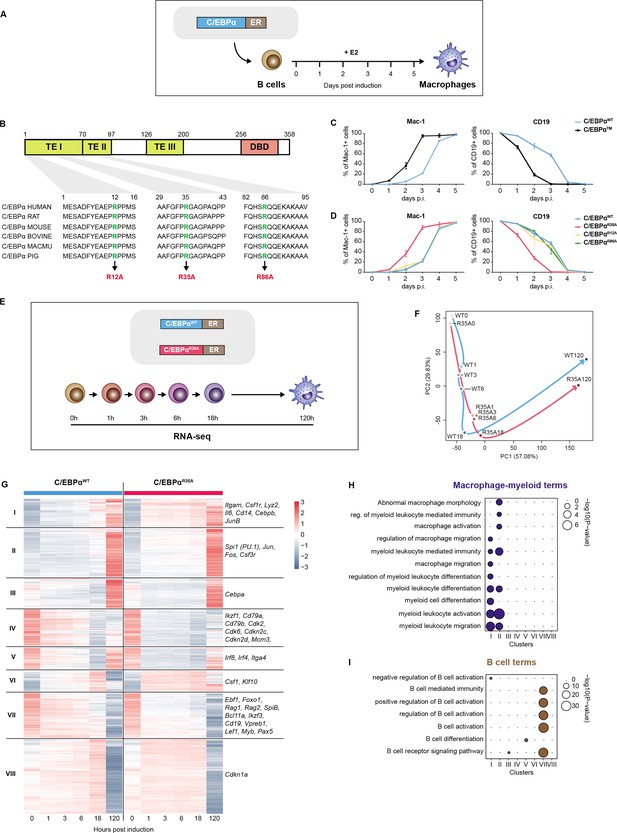
A mutation of arginine 35 in C/EBPα accelerates C/EBPα-induced B cell to macrophage transdifferentiation (BMT).
(A) Schematics of the BMT method using primary cells. Bone marrow-derived pre-B cells infected with C/EBPα-ER-GFP retrovirus were treated with E2 to induce the factor’s translocation into the nucleus, inducing a BMT within 4–5 days as assessed by Mac-1 and CD19 expression. (B) C/EBPα structure (TE = transactivation element; DBD = DNA-binding domain) and location of conserved arginines R12, R35, and R86. (C) Kinetics of BMT induced by C/EBPα wild-type (WT) and C/EBPα with alanine replacements of R12, R35, and R86. BMT (mean ± s.d., n=3). (D) As in (C) but comparing the effects of separate mutations in the three arginines, showing that C/EBPαR35A is the critical residue. (E) Schematics of experimental approach for RNA-sequencing (RNA-seq) of B cells infected with either C/EBPαWT-ER or C/EBPαR35A-ER retroviral constructs induced for various timepoints. (F) Principal component analysis (PCA) of 11,780 differentially expressed genes during BMT (n=2). Trajectories connecting individual timepoints are visualized in blue for the wild-type and in red for the mutant. (G) Hierarchical clustering of differentially expressed genes with examples shown next to each cluster. (H–I) Gene Ontology (GO) enrichment analysis of macrophage-myeloid (H) and B cell (I) terms of the clusters from (G). Diameter of circles is proportional to the p-value.
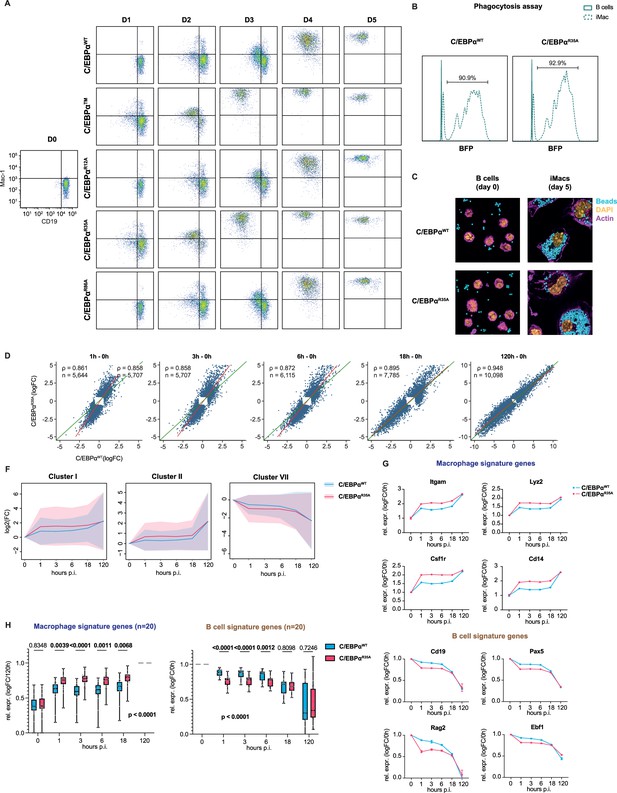
Mutation of arginine 35 in C/EBPα accelerates B cell gene silencing and macrophage gene activation.
(A) FACS plots of Mac-1 (CD11b) and CD19 expression in B cells induced with C/EBPαWT, C/EBPα, C/EBPαR12A, C/EBPαR35A, or C/EBPαR86A at different days post induction. (B) Histograms showing fluorescence intensity by flow cytometry of internalized BFP carboxylated beads in C/EBPαWT and C/EBPαR35A-induced B cells (dashed line) incubated overnight. Data for uninduced control B cells are represented by a continuous line. Percentage of phagocytic cells is indicated. (C) Immunofluorescent images of uninduced (day 0) and 5 days-induced B cells incubated overnight with BFP carboxylated beads. DNA was stained with picogreen (P7589) and F-actin with phalloidin Alexa Fluor 568. (D) Scatterplots showing gene expression changes at 1, 3, 6, 18, and 120 hpi relative to 0 hr for B cells induced with either C/EBPαWT or C/EBPαR35A. Red line = regression line fitted to each scatterplot; green line = identity line (x=y); ρ=Spearman correlation coefficient; n=number of differentially expressed genes. (F) Kinetics of gene expression of clusters I, II, and VII of B cells induced with either C/EBPαWT or C/EBPαR35A at different times post induction. The Y axis shows log2-fold changes relative to uninduced cells. The lines and the shaded backgrounds correspond to the mean ± 1.64 s.d., n=1103–1868. (G) RNA expression levels of key macrophage or B cell genes in B cells induced by either C/EBPαWT or C/EBPαR35A relative to 0 hr (mean ± s.d., n=2). (H) RNA expression levels of selected macrophage and B cell signature genes in B cells induced by either C/EBPαWT or C/EBPαR35A relative to 120 hr and 0 hr, respectively (median and quartiles are represented, n=20, statistical significance was determined using multiple paired Student’s t-test for individual timepoint comparisons as well as two-way ANOVA for overall statistical significance).
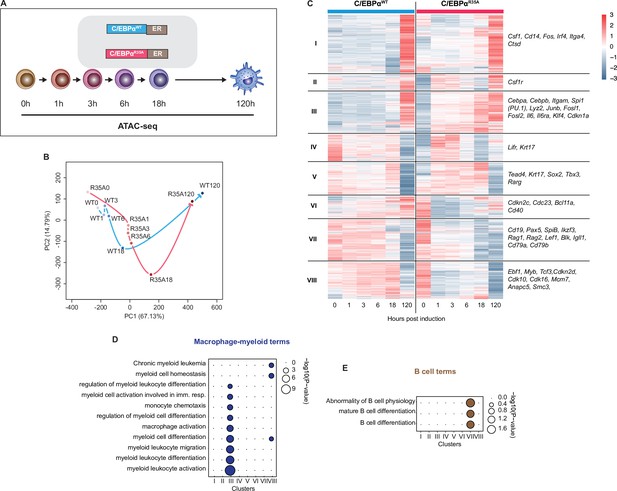
C/EBPαR35A accelerates chromatin accessibility at gene regulatory elements of lineage-restricted genes.
(A) Experimental approach used for chromatin accessibility profiling. B cells infected with either C/EBPαWT-ER or C/EBPαR35A-ER retroviral constructs (n=2 biological replicates) were induced for the indicated times and processed for assay for transposase-accessible chromatin sequencing (ATAC-seq). (B) Principal component analysis (PCA) of differential chromatin accessibility dynamics during B cell to macrophage transdifferentiation (BMT) induced by C/EBPαWT or C/EBPαR35A, based on 91,830 ATAC-seq peaks differentially called for the two conditions. Arrows connecting individual timepoints show trajectories. (C) Hierarchical clustering of differentially accessible promoters (14,233 peaks) with representative genes shown next to each cluster. (D, E) Gene Ontology analysis of macrophage-myeloid (D) and B cell (E) terms of each cluster. Diameter of circles is proportional to the p-value.
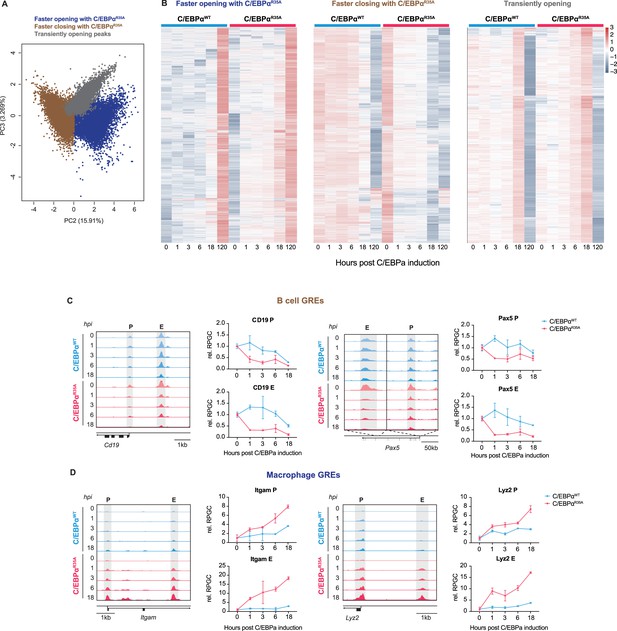
C/EBPαR35A accelerates chromatin remodeling at regulatory elements of lineage-restricted genes.
(A) Principal component analysis (PCA) of individual peaks showing PC2 and PC3 and the three clusters that were generated (n=91,830 peaks). (B) Three clusters were generated from PCA shown in (A), showing regions that are opened more rapidly with C/EBPαR35A (blue); regions that are closed more rapidly with C/EBPαR35A (brown); and regions that peak at 18 hr and are closed at 120 hr (gray). (C, D) Chromatin accessibility kinetics of key B cell GREs (C) and macrophage GREs (D). Genome browser views of ATAC peaks (gray highlights; P=promoter, E=enhancer) corresponding to GREs of B cell genes (Cd19 and Pax5) and macrophage genes (Itgam (Mac-1) and Lyz2). The coordinates of these GREs are: Cd19 promoter, chr7:126414750–126415070; Cd19 enhancer, chr7:126416582–126417061; Pax5 promoter, chr4:44710658–44711033; Pax5 enhancer, chr4:44671014–44673084; Itgam promoter, chr7:128062560–128062764; Itgam enhancer, chr7:128065643–128065864; Lyz2 promoter, chr10:117281959–117282561; Lyz2 enhancer, chr10:117284863–117285593. Kinetics of chromatin accessibility at different timepoints are displayed as reads per genomic content relative to 0 hpi (RPGC).
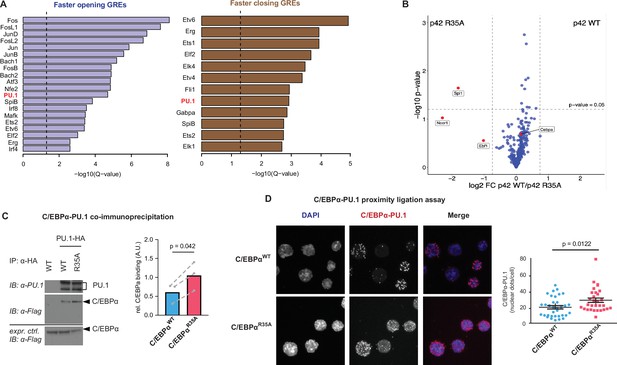
Enrichment of the PU.1 motif at differentially accessible chromatin sites and increased affinity of C/EBPαR35A for PU.1.
(A) Top de novo motifs in faster opening or closing GREs induced by C/EBPαR35A, with the PU.1 motif indicated in red. Dashed lines correspond to the significance threshold of q-value (≤0.05). (B) Protein interaction assay by mass spectrometry. Briefly, immortalized pre-B cells were either infected with retroviral vectors encoding doxycycline (Dox)-inducible full length (P42) C/EBPαWT or C/EBPαR35A proteins fused to the biotin ligase TurboID or with a TurboID only as a control. Six hours after Dox induction cells were treated with 500 μM biotin for 30 min, biotinylated proteins precipitated and analyzed by LC-mass spectrometry. Specific interactions were determined by plotting the ratio of p42 C/EBPαWT and C/EBPαR35A protein (log2-fold change) against the p-value (-log10). Vertical lines demarcate the mean ± 3 s.d. of log2-fold change ratios. Values above the horizontal line indicate significant interactions. (C) Western blot showing co-immunoprecipitation of PU.1 and C/EBPα in 293T cells transfected with either C/EBPαWT or C/EBPαR35A (left) and quantification of interaction experiments (n=3, right). Values were normalized for the expression of C/EBPα (mean + individual values). Dashed lines indicate paired values; statistical significance was determined using a paired Student’s t-test. (D) Proximity ligation assay of C/EBPα and PU.1 in primary B cells induced with either C/EBPαWT or C/EBPαR35A for 24 hr. Graph shows nuclear dots per cell (mean ± s.e., n=30–34; statistical significance determined using an unpaired Student’s t-test).
-
Figure 3—source data 1
Electrophoretic mobility shift assay (EMSA) with 293T cells transfected with either C/EBPαWT or C/EBPαR35A incubated with a fluorophore-labeled oligonucleotide containing a palindromic C/EBPα-binding site.
- https://cdn.elifesciences.org/articles/83951/elife-83951-fig3-data1-v1.docx
-
Figure 3—source data 2
Table listing 300 proteins that represent the interactome of C/EBPα showing log2-based differences in biotin labeling with the full length (p42) C/EBPaR35A protein and the p42 C/EBPαWT protein as well as p-values for the differences in the corresponding interactions.
- https://cdn.elifesciences.org/articles/83951/elife-83951-fig3-data2-v1.docx
-
Figure 3—source data 3
Co-immunoprecipitation of PU.1 and C/EBPα in 293T cells transfected with either C/EBPαWT or C/EBPαR35A and quantification of interaction.
- https://cdn.elifesciences.org/articles/83951/elife-83951-fig3-data3-v1.zip
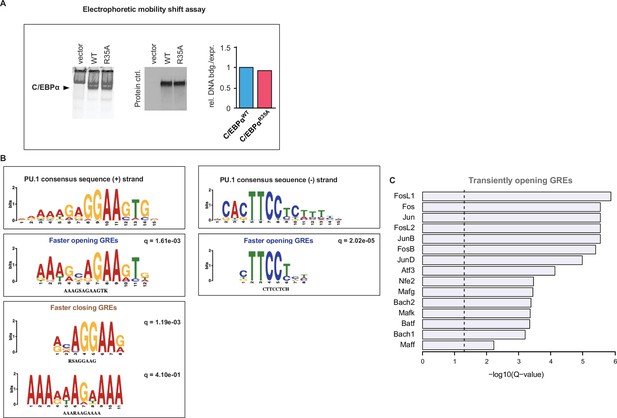
C/EBPα DNA binding capacity and motif analyses.
(A) Electrophoretic mobility shift assay of nuclear extracts from 293T cells transfected with either C/EBPαWT or C/EBPαR35A and incubated with a fluorophore-labeled oligonucleotide containing a palindromic C/EBPα-binding motif (left). Protein expression control of nuclear C/EBPα proteins by western blot (middle) and densitogram-based relative DNA binding versus protein expression (right). (B) PU.1 enriched motifs with consensus sequence in the + and – strand shown on top, as well as several additional matched enriched de novo motifs. (D) De novo motifs matched to known transcription factor (TF) motifs in transiently opened GREs. The top 15 motifs are shown in the order of their significance (log10 p-values).
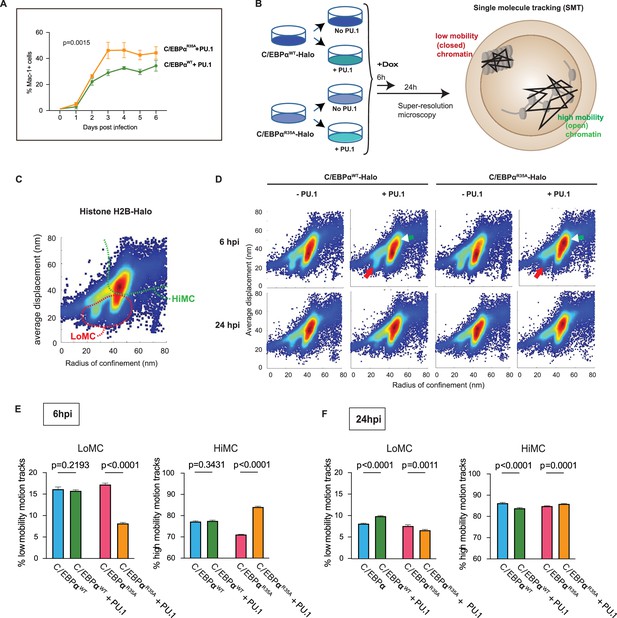
Synergy of PU.1 with C/EBPαWT and C/EBPαR35A in activating myeloid gene expression and chromatin accessibility changes in fibroblasts.
(A) NIH3T3 fibroblasts expressing C/EBPαWT-ER or C/EBPαR35A-ER were transfected with a PU.1 construct for 24 hr and induced by E2 for the indicated times, followed by FACS to monitor Mac-1 expression (mean ± s.d., n=3; statistical significance was determined using two-way ANOVA). (B) Strategy used for the single-molecule tracking (SMT) experiments: NIH 3T3 cells stably expressing doxycycline (Dox)-inducible C/EBPαWT-Halo or C/EBPαR35A-Halo (3T3aER-R and 3T3aER-A cells) were split and transfected or not with PU.1 for 24 hr and induced with Dox for either 6 or 24 hr. They were then used for SMT by HILO super resolution microscopy, allowing to determine the radius of confinement and average displacement parameters. Particles with a small radius of confinement and average displacement correspond to low mobility chromatin (LoMC); particles with a large radius of confinement and average displacement correspond to high mobility chromatin (HiMC). (C) Image shows two-parameter H2B-Halo distribution in chromatin of NIH-3T3 cells, used for calibration purposes, with a stippled red line indicating area of LoMC and a green line HiMC. (D) Two-parameter particle distribution of 3T3aER-R and 3T3aER-A cells at 6 hpi, with red arrows pointing to differences in LoMC between the various conditions and white arrowheads to differences in HiMC. (E) Quantification of two-parameter single-cell motion tracks at 6 hpi. Values represent mean ± s.d., obtained from 20,000 randomized and down-sampled motion tracks per cell (n=3); statistical significance was determined using two-way ANOVA with multiple comparisons. (F) Same as (E) but cells induced for 24 hr.
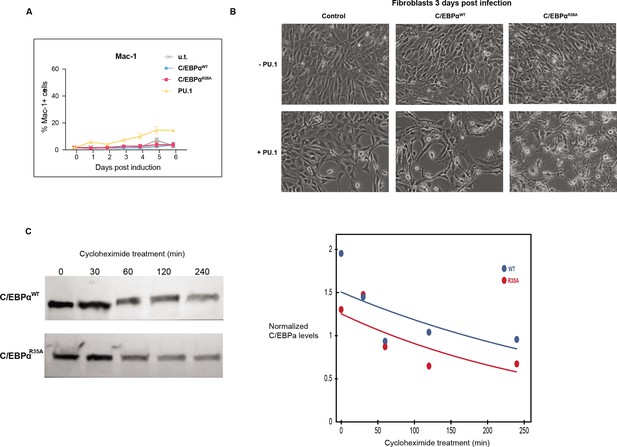
C/EBPαR35A hastens the relocation of PU.1 from B cell to myeloid GREs.
(A) Kinetics of Mac-1 expression induced in 3T3 cells by C/EBPαWT, C/EBPαR35A, or PU.1 and untransduced cells (u.t.) measured by FACS (mean ± s.d., n=3, statistical significance was determined using two-way ANOVA). (B) Phase contrast images of NIH3T3 cells induced with in combinations of C/EBPαWT or C/EBPαR35A and PU.1 for 3 days. (C) Western blots of C/EBPα expression in C/EBPαWT-Halo and C/EBPαR35A-Halo expressing lines 3T3aER-R and 3T3aER-A. Cells were induced with E2 for 24 hr, treated for the indicated times with cycloheximide and extracts used for western blotting. After obtaining a densitogram and correcting for the levels of tubulin expression, the values were plotted in the line graph on the right. (D) Single-molecule tracking (SMT) of either C/EBPαWT or C/EBPαR35A in 3T3aER-R and 3T3aER-A cells, respectively, induced for either 24 hr with or without PU.1 co-expression (n=20,000).
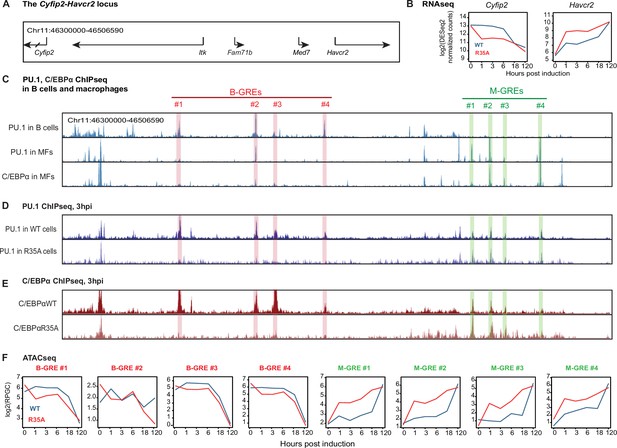
C/EBPα and PU.1 binding and chromatin accessibility changes of B cell and myeloid GREs.
(A) Structure of the Cyfip2-Havcr2 locus (chr11:46,300,000–46,506,590). (B) Kinetics of gene expression after E2 induction of C/EBPαWT or C/EBPαR35A. (C) Screenshots of the UCSC browser showing peaks corresponding to PU.1 binding in B cells and in bone marrow derived macrophages (BMDM), as well as C/EBPα binding in BMDM (gain 5000). B cell and macrophage GREs are highlighted in red and green, respectively. Coordinates of B-GREs: #1, chr11:46,343,904–46,344,618; #2, chr11:46,372,449–46,373,726; #3, chr11:46,379,961–46,380,726; #4, chr11:46,398,858–46,399,316. M-GREs: #1, chr11:46,454,489–46,455,316; #2, chr11:46,461,421–46,462,284; #3, chr11:46,466,901–46,467,255, #4, chr11:46,480,463–46,480,843. (D) Screenshots of PU.1 binding in B cells induced with E2 for 3 hr to activate expression of C/EBPαWT or C/EBPαR35A (gain 2000). (E) As in (D), but for C/EBPα binding. (F) Kinetics of chromatin accessibility, determined by assay for transposase-accessible chromatin sequencing (ATAC-seq) of B-GREs (framed in red) and M-GREs (in green) in C/EBPαWT or C/EBPαR35A-induced cells. The chromatin immunoprecipitation followed by high-throughput sequencing (ChIP-seq) data represent RPKM normalized values, the ATAC-seq data represent RPGC normalized values.
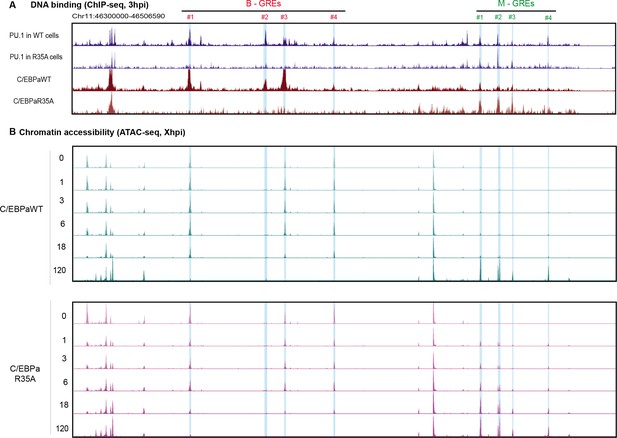
DNA binding of PU.1 and C/EBPa and assay for transposase-accessible chromatin sequencing (ATAC-seq) kinetics during B cell to macrophage transdifferentiation (BMT).
(A) Screenshots of UCSC browser showing biological duplicates of the data in Figure 5D and E. (B) ATAC-seq profiles obtained from samples collected at different timepoints during C/EBPαWT- and C/EBPαR35A-induced BMT. B- and M-GREs are highlighted in blue. Coordinates of GREs are as in Figure 5.
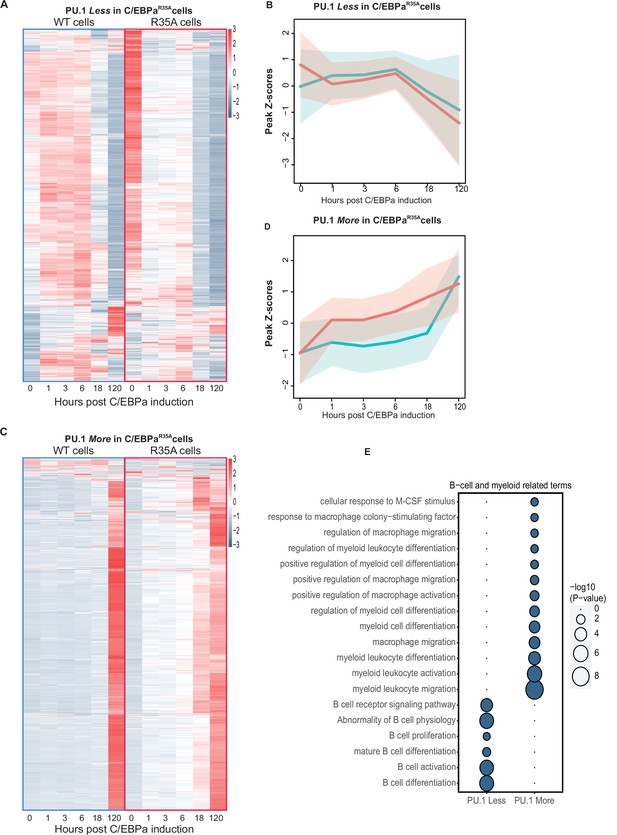
Analysis of chromatin accessibility changes during B cell to macrophage transdifferentiation (BMT) at GREs differentially bound by PU.1 in C/EBPαWT and C/EBPαR35A-induced B cells.
(A) Heat maps of ATAC peaks at sites bound Less by PU.1 in C/EBPαR35A cells compared to C/EBPαWT cells and line diagrams showing a comparison of the kinetics. Blue and red lines represent median values (log2) for wild-type and mutant C/EBPα, respectively, with shadows representing the 95% confidence interval. (C and D) Same as for (A and B), but for sites bound More by PU.1. (E and F) Gene Ontology analysis for B cell and myeloid-related terms, with size of circles being proportional to their p-values (log10).
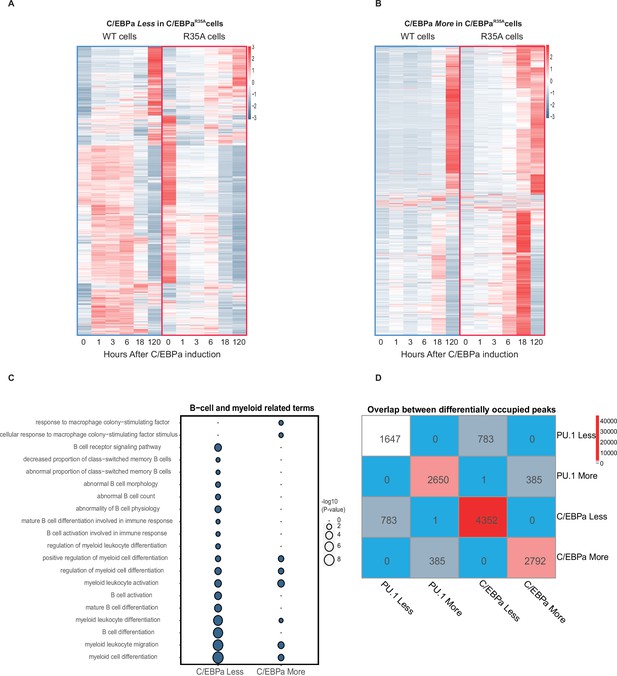
Analysis of chromatin accessibility changes during B cell to macrophage transdifferentiation (BMT) at regions differentially bound by C/EBPα in 3 hr induced C/EBPαWT and C/EBPαR35A cells.
(A) Heat maps of ATAC peaks at sites bound Less by C/EBPα in C/EBPαR35A cells compared to C/EBPαWT cells. (B) Heat maps of ATAC peaks at sites bound More by C/EBPα in C/EBPαR35A cells. (C) Gene Ontology analysis for B cell and myeloid-related terms, with size of circles being proportional to p-value (log10). (D) Number of sites bound by PU.1 and C/EBPα in wild-type and mutant C/EBPα cells, showing different pairwise combinations.
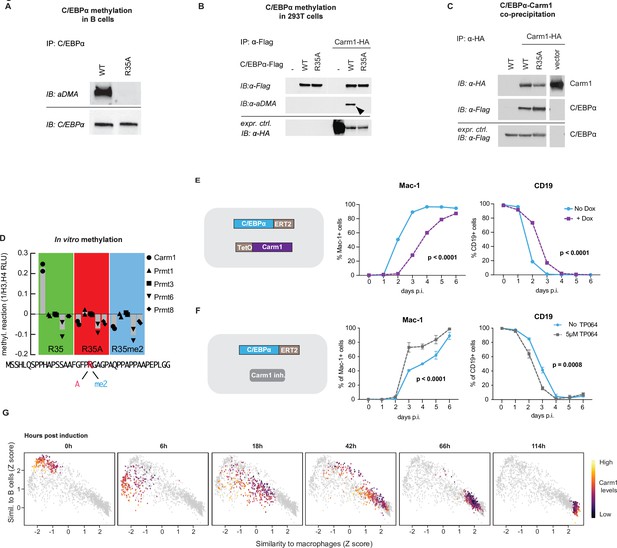
Carm1 asymmetrically di-methylates arginine 35 and the enzyme’s level correlates with the speed of C/EBPα-induced B cell to macrophage transdifferentiation (BMT).
(A) Immunoprecipitation (IP) and immunoblotting (IB) of C/EBPα and asymmetrically di-methylated arginine (aDMA) containing proteins. (B) Immunoprecipitation of C/EBPα from 293T cells co-transfected with either C/EBPαWT-Flag or C/EBPαR35A-Flag with or without Carm1-HA, followed by immunoblot with an antibody against aDMA, Flag, and HA. (C) As in (B), but immunoprecipitation of Carm1 from co-transfected 293T cells and immunoblotted with antibodies against Flag and HA. (D) In vitro methylation assay with recombinant Carm1, Prmt1, Prmt3, Prmt6, or Prmt8 proteins together with a C/EBPα peptide (aa 15–54) containing either an unmethylated arginine 35 (R in red), an alanine replacement (A in pink), or an asymmetric di-methylation (me2 in blue). (E) Effect of Carm1 overexpression on BMT kinetics of human B cells measured by Mac-1 and CD19 expression (mean ± s.d., n=3, statistical significance determined using two-way ANOVA). (F) Same as (E), but showing the effect of 5 µM of TP064. (F) Correlation with B cell and macrophage states of Carm1 expression levels in single-cell BMT trajectories. Data extracted from previously published work (Francesconi et al., 2019).
-
Figure 7—source data 1
Immunoprecipitation (IP) and immunoblotting of C/EBPα and asymmetrically di-methylated arginine (aDMA) containing proteins.
- https://cdn.elifesciences.org/articles/83951/elife-83951-fig7-data1-v1.zip
-
Figure 7—source data 2
Immunoprecipitation (IP) of C/EBPα from 293T cells co-transfected with either C/EBPαWT-Flag or C/EBPαR35A-Flag with or without Carm1-HA, followed by immunoblot (IB) with antibodies (Abs) against asymmetrically di-methylated arginine (aDMA), Flag, and HA.
- https://cdn.elifesciences.org/articles/83951/elife-83951-fig7-data2-v1.zip
-
Figure 7—source data 3
Immunoprecipitation (IP) of Carm1 from 293T cells co-transfected with either C/EBPαWT-Flag or C/EBPαR35A-Flag with or without Carm1-HA, followed by immunoblot with antibodies against Flag and HA.
- https://cdn.elifesciences.org/articles/83951/elife-83951-fig7-data3-v1.zip
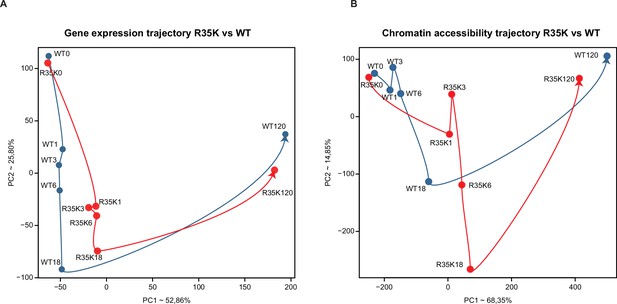
C/EBPαR35K-induced B cell to macrophage transdifferentiation (BMT): principal component analysis (PCA) of gene expression and chromatin accessibility.
(A) PCA of gene expression, showing the averages of biological duplicates. (B) PCA of chromatin accessibility, showing averages of biological duplicates. The blue lines trace the approximate trajectory for the C/EBPαWT values, the red lines for the C/EBPαR35K values.
-
Figure 7—figure supplement 1—source data 1
Immunoprecipitation (IP) of cells co-transfected with either C/EBPαWT-Flag or C/EBPαR35A-Flag and different type I Prmts followed by immunoblot with antibodies against asymmetrically di-methylated arginine.
- https://cdn.elifesciences.org/articles/83951/elife-83951-fig7-figsupp1-data1-v1.zip
-
Figure 7—figure supplement 1—source data 2
Western blot of Carm1 and GAPDH in B cell lines RRC3 and RAC1 with or without addition of doxycycline (Dox).
- https://cdn.elifesciences.org/articles/83951/elife-83951-fig7-figsupp1-data2-v1.zip
-
Figure 7—figure supplement 1—source data 3
Western blot of asymmetrically di-methylated BAF155 (AsDM-BAF155) and total BAF155 (BAF155) in B cells treated with different concentrations of TP064.
- https://cdn.elifesciences.org/articles/83951/elife-83951-fig7-figsupp1-data3-v1.zip
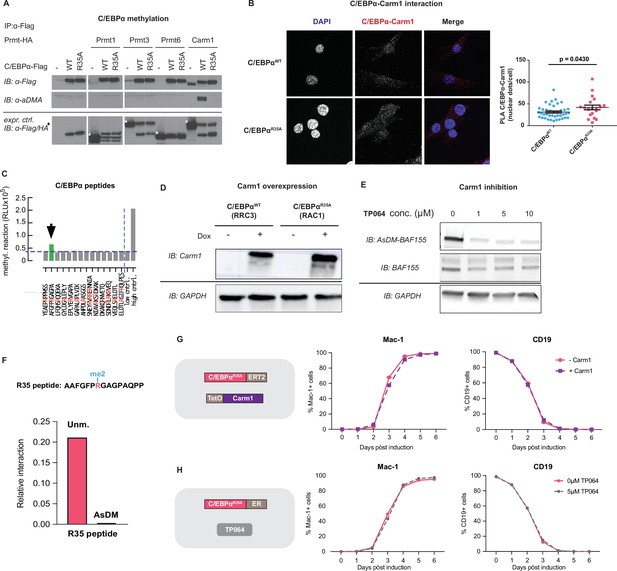
Protein methylation and proximity ligation assays (PLA); C/EBPαR35A interaction with Carm1 and effects of Carm1 perturbations on C/EBPαR35A-induced B cell to macrophage transdifferentiation (BMT).
(A) Immunoprecipitation of C/EBPαWT or C/EBPαR35A (Flag) from 293T cells co-transfected with either C/EBPαWT-Flag or C/EBPαR35A-Flag and either Prmt1, Prmt3, Prmt6, or Carm1, followed by immunoblot with antibodies against asymmetrically di-methylated arginine (aDMA), Flag or HA tags. (B) PLA of Carm1 and C/EBPαWT or C/EBPαR35A in the 3T3aER-R and 3T3aER-A cell lines induced with E2 for 24 hr. Images show cells stained with DAPI (blue) and fluorescent dots indicative of an interaction between C/EBPα and Carm1. Quantification of nuclear dots per cell is shown on the right (mean ± s.e.; n=20–40, statistical significance was determined using an unpaired Student’s t-test). (C) In vitro methylation assay using Carm1 and 13 arginine-containing peptides spanning the entire C/EBPα protein (15mer peptides, R-residues highlighted in red). Peptide containing R35 is indicated by a green bar. Low control: no enzyme; high control: optimized R-methylation peptide (BPS Bioscience). (D) Western blot of Carm1 in B cell lines RRC3 and RAC1 before and after addition of doxycycline (Dox) for 24 hr. (E) Western blot of asymmetrically di-methylated BAF155 (AsDM-BAF155) and total BAF155 in B cells treated with different concentrations of TP064 (1–10 µM). (F) PRISMA analysis comparing an unmethylated C/EBPα peptide with a peptide containing an asymmetrically di-methylated R35. (G) Kinetics of C/EBPαR35A-mediated BMT upon Carm1 overexpression by pre-treatment with Dox for 24 hr measured by Mac-1 and CD19 expression by flow cytometry (mean ± s.d.; n=3, statistical significance was determined using two-way ANOVA). (H) Kinetics of C/EBPαR35A-mediated BMT upon Carm1 inhibition by pre-treatment with TP064 for 24 hr measured by Mac-1 and CD19 expression by flow cytometry (mean ± s.d.; n=3, statistical significance was determined using two-way ANOVA).
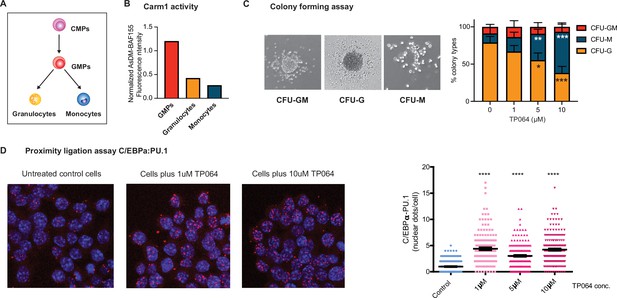
Effect of Carm1 activity on myeloid differentiation and C/EBPα-PU.1 interaction.
(A) Simplified representation of myeloid differentiation. Common myeloid progenitors (CMPs); granulocyte-macrophage progenitors (GMPs). (B) Levels of AsDM-BAF155 relative to total BAF155 in GMPs, granulocytes and monocytes as a proxy for Carm1 activity. (C) On the left, representative images of colony types obtained from GMPs grown in Methocult. On the right, quantification of colony numbers obtained in cultures without or with various concentrations of the Carm1 inhibitor TP064 for 14 days, showing percentage of bipotent (CFU-GM), monocytic (CFU-M) and granulocytic (CFU-G) colonies (mean ± s.d., n=3–4), statistical significance was determined using a one-way ANOVA for each cell type. (D) Proximity ligation assay of endogenous C/EBPα and PU.1 in the mouse macrophage cell line RAW 264.7 treated for 24 hr with TP064 or left untreated. On the left confocal microscopy images. On the right, counts of nuclear dots per cell (mean ± s.e., n=149–190 cells per condition). Four stars: p<0.0001 (statistical significance determined using an unpaired Student’s t-test.).
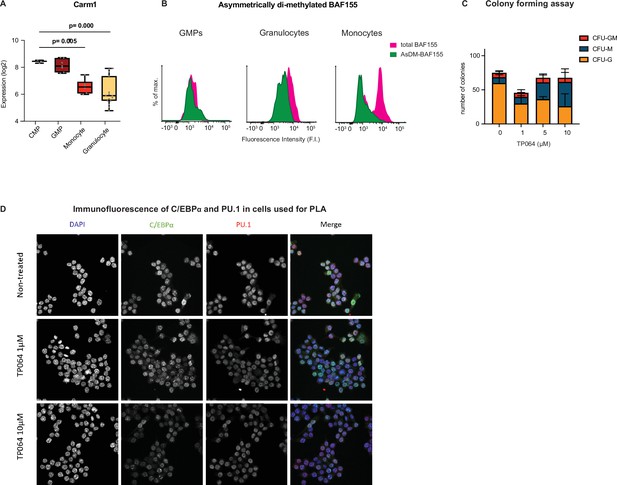
Carm1 activity in myeloid cells, effect of TP064 on viability of colony forming cells and expression of C/EBPα and PU.1 in cells used for proximity ligation assay (PLA).
Expression of Carm1 during myeloid differentiation obtained from published RNA-sequencing (RNA-seq) data (Choi et al., 2019) (n=3–7, statistical significance was determined using multiple unpaired Student’s t-tests). (B) Distribution asymmetrically di-methylated BAF155 and total BAF155 in granulocyte-macrophage progenitors (GMPs), granulocytes and monocytes as determined by FACS. (C) Total number of bipotent (CFU-GM), monocytic (CFU-M) and granulocytic (CFU-G) colonies obtained from GMPs treated with various concentrations of the Carm1 inhibitor TP064 after 12 days in Methocult. Values represent mean ± s.d., n=3–4, statistical significance determined using a one-way ANOVA. (D) C/EBPα and PU.1 fluorescence in RAW cells used for the PLA shown in Figure 8D. DNA was stained with DAPI, C/EBPα with AF488 and PU:1 with AF546 antibodies.
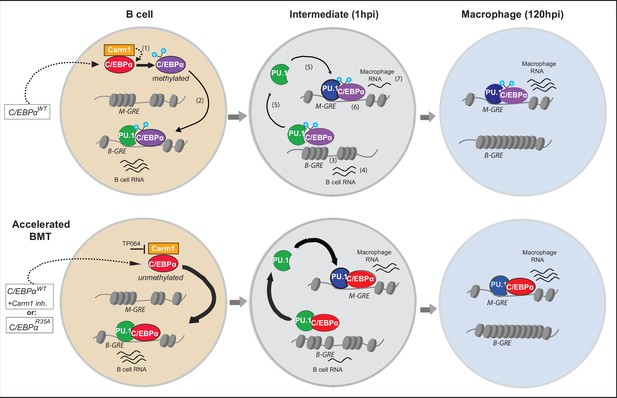
Diagram summarizing the mechanism of C/EBPα-induced B cell to macrophage transdifferentiation (BMT) and its acceleration by the R35 mutant.
Starting with a B cell shortly after E2-induced translocation of C/EBPαER into the nucleus, the C/EBPα-induced BMT occurs in various steps, illustrated in the upper row. In a first step incoming C/EBPα is bound by Carm1 and becomes asymmetrically di-methylated on arginine 35 (1). Then, C/EBPα interacts with PU.1, bound to B cell-restricted gene regulatory elements (B-GREs) (2). This induces PU.1 release, chromatin closing at B-GREs (3) and downregulation of associated B cell genes (4). Free PU.1 (possibly in a complex with C/EBPα) relocates to macrophage-restricted GREs (M-GREs), which are also bound by C/EBPα (5) resulting in chromatin opening at M-GREs (6) and upregulation of associated macrophage genes (7). As shown in the lower row, unmethylated C/EBPα, either because it is mutated at R35 or Carm1 is inhibited, interacts with a higher affinity for PU.1 than the wild-type, leading to the acceleration of all subsequent steps. In the diagram PU.1 is represented as a green icon when acting as a B cell regulator and as a blue icon after being repurposed as a myeloid regulator. Methylated and unmethylated C/EBPα are represented by purple and red icons (their depiction ignores the fact that C/EBPα binds as homo-or heterodimers). In the model we assume that methylated and unmethylated C/EBPα have distinct but overlapping functions. However, it is possible that C/EBPαWT cells contain a mixture of methylated and unmethylated C/EBPα, and that only the unmethylated form is active and can induce BMT. We assume that the methylated form is active in other cellular contexts, such as during granulocyte specification.
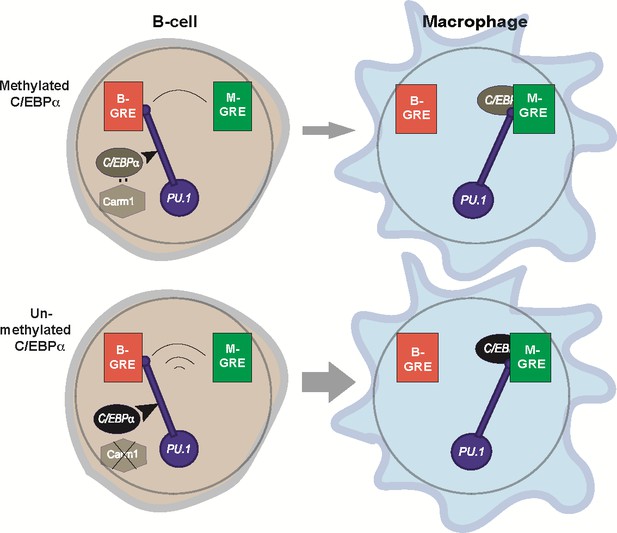
Relay model of B cell to macrophage transdifferentiation (BMT).
In this model we propose that PU.1 acts as a relay that coordinates the silencing of B cell-restricted and activation of macrophage-restricted genes. The relay is triggered by C/EBPα entering into the nucleus (curved arrow) where it can interact with Carm1, which in turn can modulate C/EBPα activity (for details, see Figure 9). PU.1 is initially bound to B-GREs in B cells, and during BMT gets relocated to M-GREs that are co-occupied by C/EBPα. The relay velocity is tuned by the potency of incoming C/EBPα, that is, whether or not the factor is methylated. The mutually antagonistic regulation of B cell and macrophage genes enabled by this mechanism ensures the faithful generation of the macrophage phenotype and prevents the formation of stable cell states that express mixed lineage markers (‘confused cells’). Of note, the relay mechanism is unidirectional since about 24hpi B cells become irreversibly committed to macrophages, due to the upregulation of endogenous Cebpa that maintains the novel cell state (Bussmann et al., 2009).
Additional files
-
Supplementary file 1
Antibodies used for cell surface staining in flow cytometry experiments.
The table lists the antibodies used for the experiments and the sources. Related to Figure 1A, C and D; Figure 2A; Figure 1—figure supplement 1A and Figure 7—figure supplement 2G,H
- https://cdn.elifesciences.org/articles/83951/elife-83951-supp1-v1.docx
-
Supplementary file 2
Materials used to prepare buffers for western blot experiments.
The table lists the chemical reagents used for western blot experiments. (Figures 3C;; 7A, B, C; Figure 4—figure supplement 1C; Figure 7—figure supplement 2A,D,E).
- https://cdn.elifesciences.org/articles/83951/elife-83951-supp2-v1.docx
-
Supplementary file 3
Antibodies used for western blot experiments.
The table lists the antibodies used for western blot experiments and their sources (Figures 3C;; 7A, B, C; Figure 4—figure supplement 1C; Figure 7—figure supplement 2A, D, E).
- https://cdn.elifesciences.org/articles/83951/elife-83951-supp3-v1.docx
-
Supplementary file 4
Peptides used for in vitro methylation experiments.
The table lists the peptides used for the in vitro Carm1 methylation experiments (Figure 7—figure supplement 2C).
- https://cdn.elifesciences.org/articles/83951/elife-83951-supp4-v1.docx
-
MDAR checklist
- https://cdn.elifesciences.org/articles/83951/elife-83951-mdarchecklist1-v1.pdf