High-resolution structures with bound Mn2+ and Cd2+ map the metal import pathway in an Nramp transporter
Figures
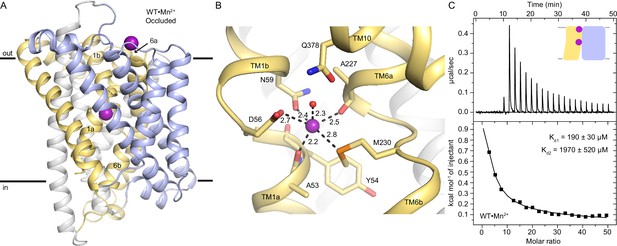
The occluded structure of DraNramp reveals a largely dehydrated Mn2+-coordination sphere.
(A) Cartoon representation of WT•Mn2+ in an occluded state. Anomalous signal confirmed the presence of Mn2+ in both the orthosteric metal-binding site and an additional site at the mouth of the external vestibule (Figure 1—figure supplement 1A) which is less conserved across the Nramp family (Figure 1—figure supplement 3). TM1 and TM6 are labeled. (B) Detail of the orthosteric metal-binding site of WT•Mn2+ where D56, N59, M230, and the pseudo-symmetrically related carbonyls of A53 and A227 coordinate the Mn2+ ion (Figure 1—figure supplement 1B). A water molecule completes the six-ligand coordination sphere. Coordinating residues are shown as sticks, and coordinating distances are indicated in Å. (C) ITC measurement of the affinity of WT DraNramp for Mn2+. Top graph shows heat absorbed upon injection of Mn2+ solution to the protein solution. Bottom graph shows the fit of the integrated and corrected heat to a binding isotherm. The data show an endothermic mode of binding and fits best with a two-site sequential binding model. The figure shows one of three measurements and the average Kd values ± SEM (Kd1=190±30 µM, Kd2=1970±520 µM; see Appendix 1). Based on ITC experiments comparing Mn2+ binding to WT or DraNramp constructs with mutations at the external site (Figure 1—figure supplement 2A), we assigned Kd1 to the orthosteric site. In all figures, unless otherwise noted, TMs 1, 5, 6, and 10 are pale yellow, TMs 2, 7, and 11 gray, TMs 3, 4, 8, and 9 light blue, and Mn2+ atoms are magenta spheres.
-
Figure 1—source data 1
Multiple sequence alignment of 6172 Nramp homologs.
- https://cdn.elifesciences.org/articles/84006/elife-84006-fig1-data1-v2.zip
-
Figure 1—source data 2
Maximum likelihood phylogenetic tree of Nramp homologs built with RAxML-NG.
- https://cdn.elifesciences.org/articles/84006/elife-84006-fig1-data2-v2.zip
-
Figure 1—source data 3
Raw data of metal ion uptake into proteoliposomes measured at four ΔΨ values for each DraNramp construct.
- https://cdn.elifesciences.org/articles/84006/elife-84006-fig1-data3-v2.xlsx
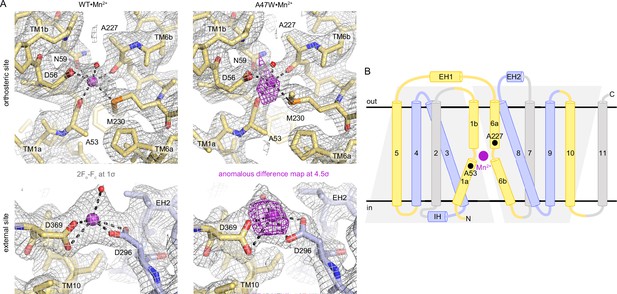
Structure of Mn2+ binding at the orthosteric and external sites of DraNramp.
(A) 2Fo-Fc (gray mesh; 1σ) and peaks from anomalous difference Fourier (magenta mesh; 4.5σ) maps calculated from the WT•Mn2+ and A47W•Mn2+ structures, respectively show Mn2+ bound at two sites, the orthosteric site (top) and an external site coordinated by D296 and D369 near the N-termini of EH2 and TM10, respectively (bottom). (B) Topology diagram showing the secondary structure organization of DraNramp with its characteristic LeuT fold, where TMs 1–5 and 6–10 form two pseudosymmetric inverted repeats. One intracellular helix, IH, and two extracellular helices, EH1 and EH2, connect TMs 2–3, 5–6, and 7–8, respectively. Black spheres indicate A53 in TM1a and A227 in TM6a, the two Mn2+-coordinating backbone carbonyls in the occluded state of DraNramp, one in each inverted repeat. Mn2+ is shown as magenta sphere.
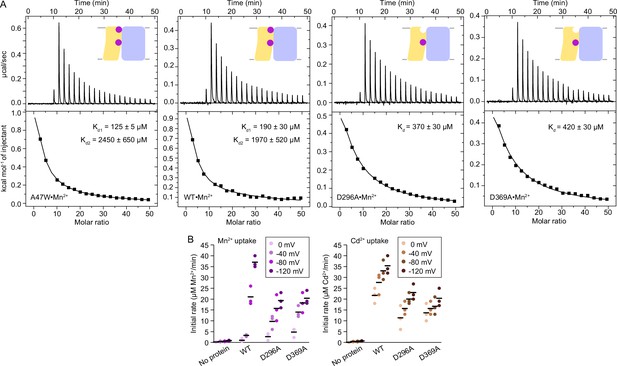
Affinity of Mn2+ for the orthosteric and external sites of DraNramp and transport activity of external-site variants.
(A) ITC measurements of Mn2+ binding to A47W (which behaves like WT) and WT (reproduced here from Figure 1 for comparison) which fit best to a two-site sequential binding model, and D296A and D369A (constructs with point mutations to either of the external site aspartates), which were fit using a one-site model with a fixed n=1 (and did not fit with a two-site model). The resulting Kd values for D296A and D369A are more similar to Kd1 of WT, indicating that the orthosteric site has higher Mn2+ binding affinity compared to the external site. The figure shows one of 2–3 measurements and the average Kd values ± SEM (see Appendix 1). (B) Initial metal uptake rates for DraNramp mutants at membrane potentials ranging from ΔΨ=0 to −120 mV (n=3; each data point is represented in the scatter plots and the black bars are the mean values). The metal ion concentration was 750 μM, and the pH was 7 on both sides of the membrane. D296A and D369A moderately reduced the initial transport rate at high membrane potentials. The overall trends are similar for both metals. Mn2+ transport showed higher voltage dependence than Cd2+ transport. Corresponding time traces are plotted in Figure 1—figure supplement 4.
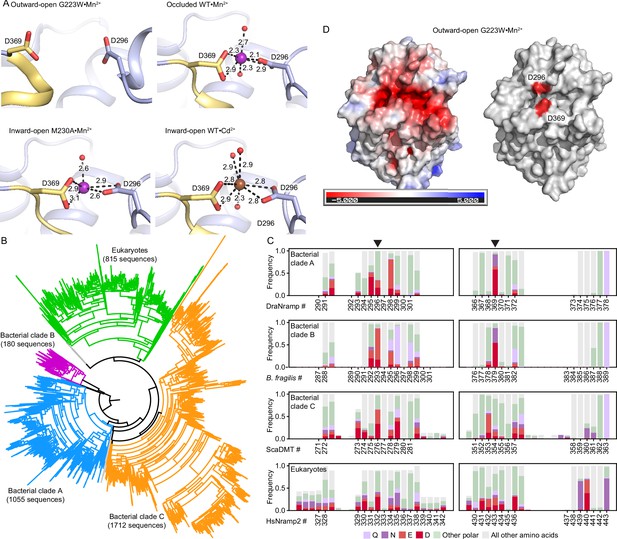
The external metal-binding site in DraNramp is somewhat conserved in clade A homologs, but poorly conserved across all Nramps.
(A) External site architecture in the G223W•Mn2+ (outward-open), WT•Mn2+ (occluded), M230A•Mn2+ and WT•Cd2+ (inward-open) structures showing positions of D296 and D369 across conformations, illustrating that the two residues are farther apart in the outward-open structure and cannot bind metal. Metal-coordinating distances are listed in Å. (B) A maximum likelihood phylogenetic tree illustrating evolutionary divergence of the Nramp family into several major clades for prokaryotes (clades A, B and C) and eukaryotes. (C) Frequencies of acidic and other polar amino acids in the loop regions surrounding D296 (left; loop preceding EH2) and D369 (right; loop preceding TM10) across phylogenetic clades, based on the sequence alignment used to build the tree in panel B (bacterial clade A, DraNramp numbering; bacterial clade B, Bacteroides fragilis MntH numbering; bacterial clade C, ScaDMT numbering; eukaryotic clade, human Nramp2 numbering). Across all clades, these two external loops have a high concentration of acidic amino acids. However, the exact positions of acidic residues observed in DraNramp—296 and 369 (arrowheads)—are not highly conserved except 369 in bacterial clades A and B, which is an aspartate, asparagine, or glutamate in most sequences (92.1% and 87.8% in clades A and B, respectively) (D) APBS (Jurrus et al., 2018)-generated electrostatic surface potential of the outward-open structure viewed from the extracellular side illustrates that D296 and D369 contribute to a funnel of negative charge leading into the orthosteric binding site.
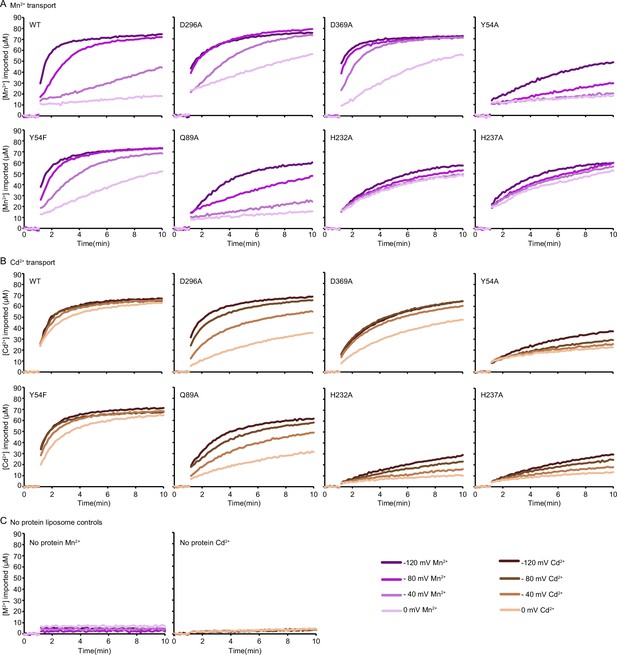
Representative time traces of metal ion uptake into proteoliposomes (n=2–3) measured at four ΔΨ values for each DraNramp construct.
(A, B) Mn2+ (A) and Cd2+ (B) uptake for WT and mutant DraNramp constructs. (C) Representative time traces of Mn2+ (left) and Cd2+ (right) uptake (n=3) shows that no metal was imported into control liposomes. The initial metal uptake rates calculated from these time traces are in Figure 1—figure supplement 2A, Figure 3C, and Figure 5—figure supplement 4F. The source data for all plots are provided as Figure 1—source data 3.
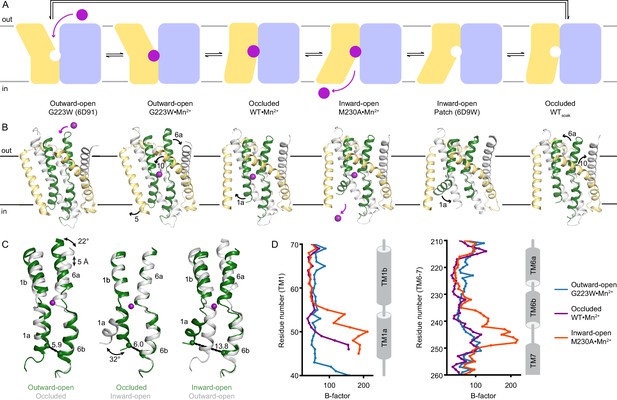
Structures in new conformations complete the Mn2+ transport cycle by DraNramp.
(A) Schematic of the conformational states that DraNramp traverses to import Mn2+. The mobile and stationary parts are pale yellow and light blue, respectively. (B) Corresponding structures of DraNramp, showing TMs 1 and 6 in green, TMs 5 and 10 in pale yellow, and TMs 2, 7, and 11 gray. Stationary TMs 3, 4, 8, and 9 are omitted to highlight the key motions in the mobile parts. Mn2+ ions are magenta. Black arrows indicate the key motions in TMs 1a and 6a detailed in panel (C), and TMs 5 and 10 detailed in Figure 2—figure supplement 1. Full structures and the electron density for TMs 1 and 6 are illustrated in Figure 2—figure supplement 2. (C) Pairwise superpositions of whole Mn2+-bound structures highlight the motions of TMs 1 and 6. Conformations are indicated at the bottom. The distance between residues 46 and 240 in TMs 1a and 6b, indicated for the green structures, increases from 5.9 Å to 13.8 Å from outward open to inward open. The large angular motions of TM1a and TM6b are also indicated. (D) Plots of B-factor by residue for the TM1 region (residues 40–70) and the TM6 region. The B-factors are highest for the inward-open state in which the interaction between TMs 1a and 6b (both in the inner leaflet) is broken.
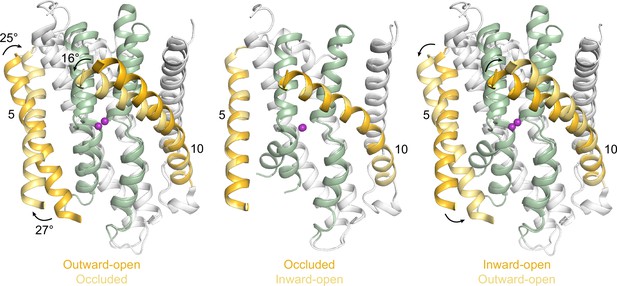
Pairwise superpositions of Mn2+-bound structures highlighting the motions of TMs 5 and 10.
Conformations are indicated at the bottom. The angular motions of TMs 5 and 10 across conformations are indicated by black arrows. All TMs other than TMs 5 and 10 are made transparent. The helices colored as in Figure 2B.
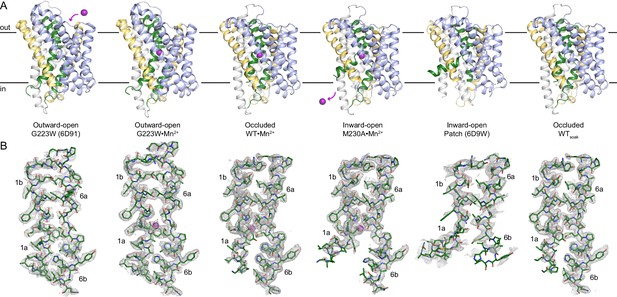
Structures of different conformational states of the DraNramp Mn2+ transport cycle.
(A) Crystal structures of each state of DraNramp. TMs 1 and 6 are colored green, TMs 5 and 10 are colored pale yellow, TMs 2, 7, and 11 in gray and TMs 3, 4, 8, and 9 are light blue. The Mn2+ ion in the orthosteric site, when present, is shown as a magenta sphere. (B) Corresponding 2Fo-Fc maps contoured at 1σ for TMs 1 and 6 of each structure illustrated in A.
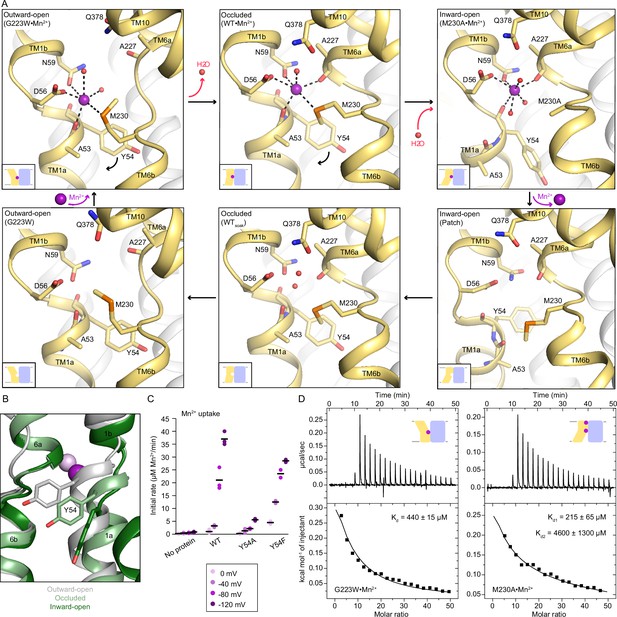
Coordination sphere changes across the Mn2+ transport cycle of DraNramp.
(A) Structures of the orthosteric metal-binding site in six conformations reveal the differences in coordination geometry and illustrate that the bound Mn2+ is more hydrated in the outward-open and inward-open states than the occluded state. In the occluded structure of metal-free WT DraNramp a density we have assigned as water replaces Mn2+. Y54 in TM1a progressively moves to open the inner vestibule in the transition from outward to inward open, shown by black curved arrows. (B) TM1 and TM6 from a superposition of the three Mn2+-bound structures in panel a illustrate the swing of the Y54 sidechain as sticks. The view is rotated 180° along the vertical axis from Figure 2C. (C) Initial Mn2+ uptake rates for DraNramp variants Y54A and Y54F at membrane potentials ranging from ΔΨ=0 to −120 mV (n=2–3; each data point is on the scatter plot and black bars are the mean values). The Mn2+ concentration was 750 μM, and the pH was 7 on both sides of the membrane. Y54A nearly abolishes transport whereas Y54F has near-wildtype initial transport rates. Corresponding time traces are plotted in Figure 1—figure supplement 4. (D) ITC measurements of G223W (left; one-site binding model with fixed n=1) and M230A (right; two-site sequential binding model) binding to Mn2+. One isotherm is shown of two measured, and the listed Kd values are the average ± SEM (see Appendix 1 for ITC analysis).
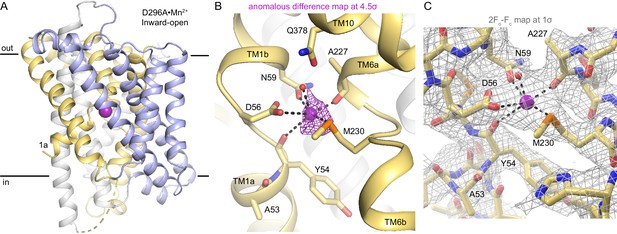
Structure and Mn2+-binding site architecture of D296A•Mn2+.
(A) Cartoon representation of the inward-open structure of D296A•Mn2+, which is nearly identical to the M230A•Mn2+ inward-open structure (Cα RMSD of 0.38 Å). (B) Coordination sphere of the orthosteric Mn2+ ion in the D296A structure is nearly the same as in M230A Mn2+-bound structure except for the sulfur of M230 replacing a water seen in M230A. We do not observe a bound water to complete the coordination sphere of D296A, likely because the resolution of the structure is lower (2.52 Å for M230A•Mn2+ vs. 3.12 Å for D296A•Mn2+), otherwise the structures are analogous. The peak from the anomalous difference Fourier map (magenta mesh; 4.5σ) calculated from a D296A•Mn2+ crystal confirms a bound Mn2+ in this inward-open state. (C) 2Fo-Fc map (gray mesh; 1σ) of the orthosteric site of D296A•Mn2+.
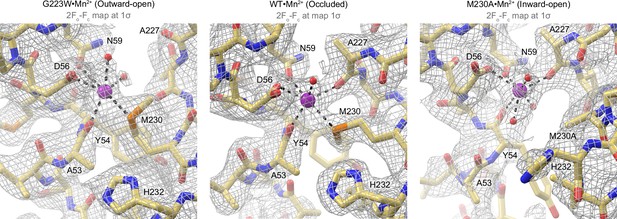
2Fo-Fc maps (gray mesh; 1σ) of the Mn2+-coordination sphere at the orthosteric site across different conformations of the Mn2+ transport cycle of DraNramp.
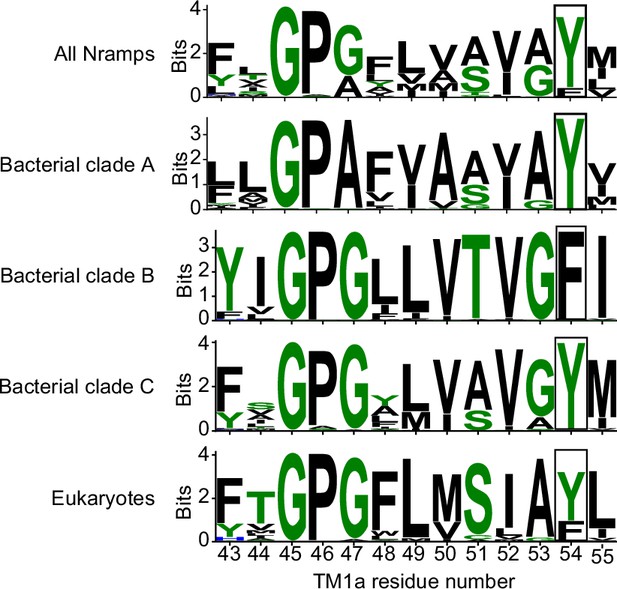
Sequence logos highlighting that Y54 in TM1a is 80% conserved in all Nramps (3762 sequences), 100% conserved in bacterial clades A and C, but replaced by a phenylalanine in clade B.
Eukaryotic Nramps have either tyrosine or phenylalanine at the corresponding position. Residue coloring is based on the ‘chemistry’ coloring scheme of WebLogo (Crooks et al., 2004). Clades are as defined in Figure 1—figure supplement 3B.
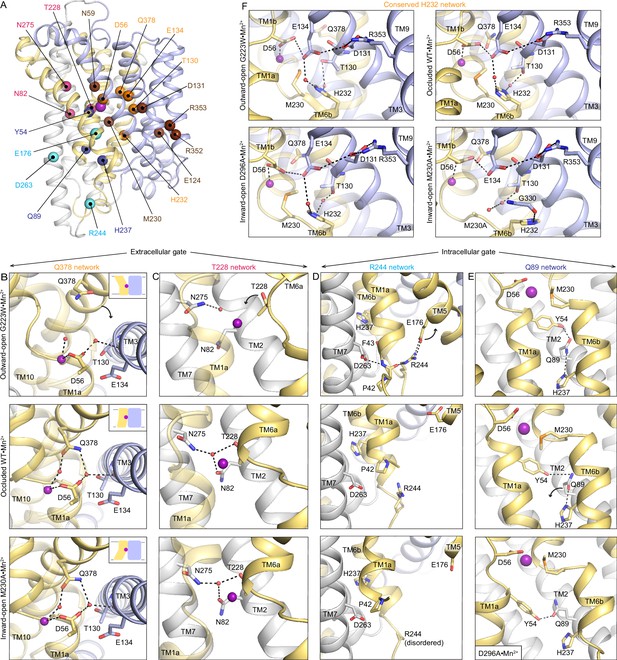
Networks of polar residues lining the outer and inner vestibules rearrange through the conformational transitions needed for Mn2+ transport.
(A) The Cα positions of residues in the Q378 (orange) and T228 (pink) networks lining the outer gate, R244 (cyan) and Q89 (blue) networks within the inner gate, and the H232 (orange) network coordinating with the proton pathway, are mapped on the occluded WT•Mn2+ structure. Metal-binding and proton pathway residues (Bozzi et al., 2019b) are represented as brown spheres. (B) The Q378 network forms as TM10 moves when DraNramp transitions from outward-open to occluded to close the outer vestibule. Water-mediated interactions form between Q378, D56, and T130. The other D56 carbonyl interacts directly with Mn2+. TM1 is transparent. (C) The T228 network forms with N275, N82, and T228 coordinating a water as TM6a moves to close the outer vestibule. TM1 is transparent. (D) In the R244 network, interactions between R244, E176, and D263 break as TM5 moves in the transition from outward-open to occluded state to initiate the opening of the inner vestibule. (E) In the Q89 network, Y54, Q89, and H237 rearrange from occluded to inward-open state as TM1a swings up to allow for metal release. (F) H232, which abuts the orthosteric Mn2+-binding site, interacts with E134 and T130 through waters conserved in all conformations. In the M230A•Mn2+ structure, H232 flips and is replaced by a water, retaining the interaction with T130 but breaking the connection with E134, suggesting that M230 helps stably position H232. TM8 is transparent. In panels c-e, TMs 3, 4, 8, and 9 are omitted to better visualize the interactions. The illustrated structures are indicated on the figure.
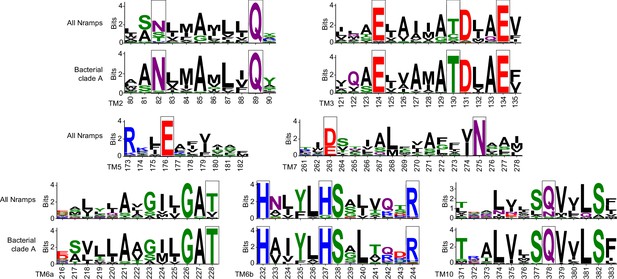
Sequence logos showing conservation of polar residues important for opening and closing the gates during Mn2+ transport (highlighted in black boxes).
The alignment contains 3762 Nramp sequences, including 1055 bacterial clade A Nramps. Most residues that are less conserved in all Nramps are completely conserved in clade A to which DraNramp belongs. Residue coloring is based on the WebLogo scheme for amino acid chemistry (Crooks et al., 2004).
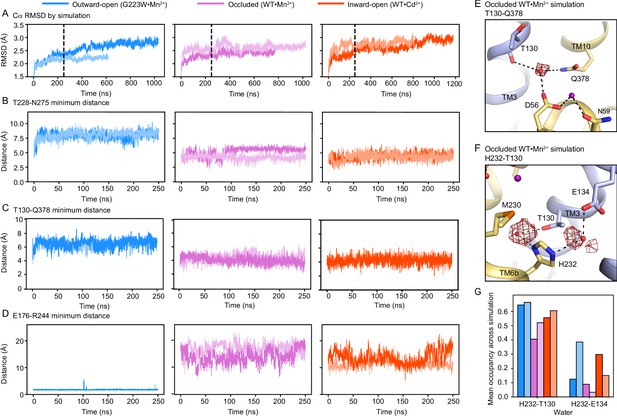
Structural stability of gating residue networks of the Mn2+ transport cycle.
(A–D) Time-course analyses of MD simulations starting in each of the three major conformations, showing replicates in different shades. (A) RMSD, calculated over all Cα atoms relative to the starting structure. Because some simulations experience substantial conformational changes after the first 250 ns (dashed line), we focused on this initial range for subsequent analyses. (B–C) Plots of the minimum distance between T228 and N275 (B) and T130 and Q378 (C) in the outer vestibule show that these interactions are stable in the inward-occluded and inward-open simulations but do not form in the outward-open simulations. (D) Likewise, simulations show that E176 and R244, located in the inner vestibule on TM5 and TM7, respectively, stably interact in the outward-open conformation, but not the occluded or inward-open conformations. (E–F) Representative 50% contour maps of water density calculated from a simulation starting the occluded conformation. (E) The water bridging T130, Q378 and D56 persists throughout most of the simulation, as do (F) the waters coordinated by H232 and T130 and H232 and E134, suggesting that all these waters-mediated interactions are robust. (G) A water is coordinated by H232 and T130 in 40–60% of frames across all simulations. A water is also coordinated by H232 and E134 but less frequently, in 2–40% of frames.
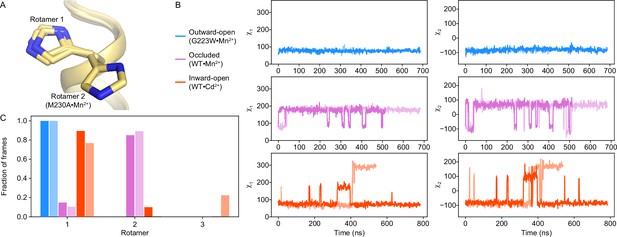
The H232 rotamer is fixed in the outward-open state but dynamic in the occluded and inward-open states.
(A) H232 takes on rotamer 1 in all structures, except in the M230A structure where it takes on rotamer 2. (B) Time-course analyses of the χ1 and χ2 angles of the H232 sidechain during the MD simulations starting in each of the three major conformations, showing replicates in different shades. Interestingly, the simulations in the occluded state show H232 rapidly switching to rotamer 2 and primarily occupying the rotamer 2 state throughout the simulation. In contrast, H232 is fixed in rotamer 1 throughout the outward-open simulation and shows the most flexibility in the inward-open state. (C) Distribution of H232 rotamer states across each MD simulation.
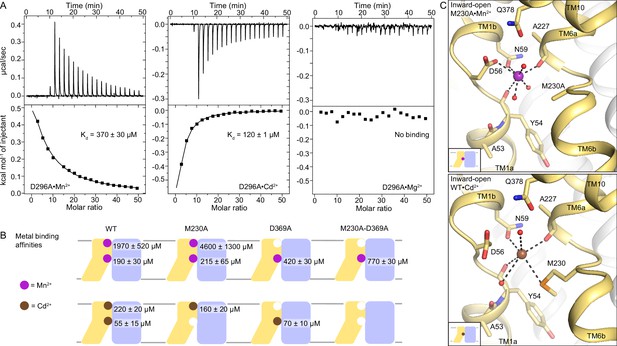
DraNramp binds differently to Mn2+ and Cd2+.
(A) ITC measurements show that DraNramp binds Mn2+ in an endothermic mode, Cd2+ in an exothermic mode and does not bind Mg2+ (6 mM metal). ITC was performed with DraNramp in which the external site is mutated (D296A) to analyze binding at the orthosteric site. One isotherm is shown of 2 or 3 measured. These isotherms were fit using a one-site model with fixed n=1, and the listed Kd values are the average ± SEM (see Appendix 1 for ITC analysis). (B) Schematic showing the ITC-measured Kd values of various DraNramp constructs for Mn2+ (top) and Cd2+ (bottom). WT DraNramp binds Mn2+ and Cd2+ at the same two sites, with the external-site affinity ~10-fold higher and the orthosteric-site affinity ~threefold higher for Cd2+ than Mn2+. The M230A mutation eliminates binding of Cd2+ but not Mn2+ at the orthosteric site. The D369A mutation eliminates binding of either metal at the external site. A variant with mutations at both the orthosteric and external sites, M230A-D369A, does not bind Cd2+ but maintains orthosteric site binding for Mn2+. For all ITC data, the Kd values were computed while fixing the number of sites (n) to 1 or 2 and assigned to the external or orthosteric site based on knowledge of the crystal structures and mutational analysis (Appendix 1; Supplementary file 1g). ITC traces are shown in Figure 5—figure supplement 1A, Figure 5—figure supplement 2 and Figure 5—figure supplement 3. (C) Comparison of the inward-open state bound to Mn2+ (M230A•Mn2+; top) and Cd2+ (WT•Cd2+; bottom) shows differences in coordination geometry at the orthosteric site. D56 is oriented differently and does not directly coordinate Cd2+. Cd2+ coordinates N59, M230, carbonyls of A227 and Y54, and two waters, for a total of six ligands compared to seven for Mn2+. Mn2+ and Cd2+ are magenta and brown spheres, respectively. Of note, we use M230A•Mn2+ here because soaking of WT crystals with Cd2+ yielded an inward-open WT•Cd2+ structure (Figure 5—figure supplement 1C) whereas soaking the same kind of crystals with Mn2+ yielded an occluded WT•Mn2+ structure (Figure 1).
-
Figure 5—source data 1
Source files (Origin files) of ITC experiments of magnesium binding to each DraNramp construct.
- https://cdn.elifesciences.org/articles/84006/elife-84006-fig5-data1-v2.zip
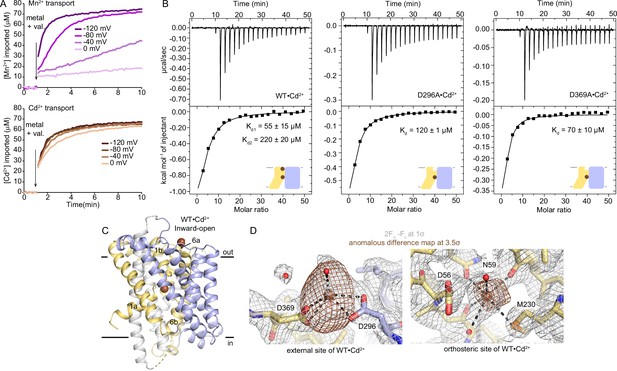
Analysis of Cd2+ and Mn2+ binding to DraNramp reveal differences.
(A) Proteoliposome-based assay with WT DraNramp showed higher voltage dependence for Mn2+ transport compared to Cd2+, with substantial transport of only Cd2+ at 0 mV. The concentration of metal used in this assay was 750 µM. (B) ITC measurements of WT, D296A, and D369A constructs of DraNramp binding to Cd2+. All Cd2+ isotherms show an exothermic mode of binding and are fit using a two-site sequential binding model (Kd1=55±15 µM, Kd2=220±20 µM) for WT, and one-site model with fixed n=1 for both D296A and D369A. By comparing ITC results from binding of Cd2+ to these constructs, we assigned a lower affinity (Kd2) to the external site. One isotherm is shown of 2 or 3 measured, and the listed Kd values are the average ± SEM (see Appendix 1 for ITC analysis). (C) Cartoon representation of WT•Cd2+, with Cd2+ ions as brown spheres. The positions of TM1 and TM6, especially the upward swing of TM1a, confirms the inward-open conformation. (D) Peaks from the anomalous difference Fourier map (brown mesh; 3.5σ) and electron density from the 2Fo-Fc map (gray mesh; 1σ) from WT•Cd2+ show that Cd2+ binds at both the external and orthosteric sites.
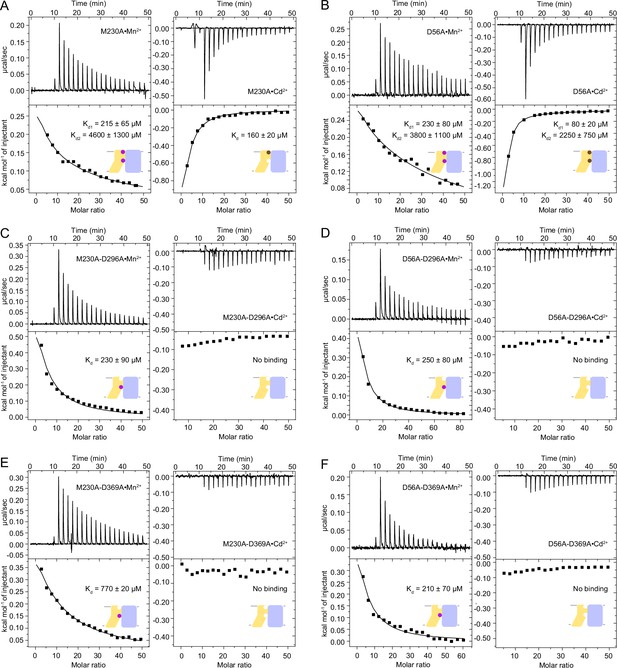
ITC measurements comparing the affinity for Mn2+ and Cd2+ of DraNramp constructs with mutations at metal-binding sites.
(A) M230A mutation at the orthosteric site impacts Cd2+ binding more than Mn2+. (B) D56A mutation leads to weaker binding compared to WT for both metals at both binding sites. (C–F) DraNramp constructs with double mutations, one at each metal binding site (M230A or D56A and D296A or D369A), show similar binding behavior, retaining binding of Mn2+ at the orthosteric site, but no Cd2+ binding. All Mn2+ and Cd2+ binding isotherms are endothermic and exothermic, respectively. One isotherm is shown of 2 or 3 measured, and the listed Kd values are the average ± SEM (see Appendix 1 for ITC analysis).
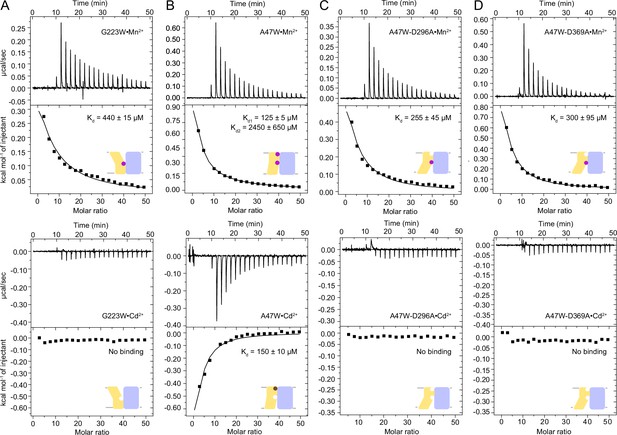
ITC measurements comparing Mn2+ and Cd2+ binding affinity of the conformation-locking mutants.
(A) In the G223W outward-locked construct, the orthosteric site is intact but the external-site ligands are far apart because of the opening of the extracellular vestibule (Figure 1—figure supplement 3A). Mn2+ binds at the orthosteric site in G223W, but Cd2+ binding is impaired. (B) The A47W inward-locked occluded construct binds both Mn2+ and Cd2, with two-site sequential binding and one-site binding models yielding the best fits, respectively. (C–D) Double mutants of A47W with either D296A (C) or D369A (D) retain Mn2+ binding but not Cd2+ binding, indicating that Cd2+ binds only at the external site and not the orthosteric site of the A47W construct, whereas Mn2+ binds at both binding sites. All Mn2+ and Cd2+-binding isotherms are endothermic and exothermic, respectively. One isotherm is shown of 2 or 3 measured, and the listed Kd values are the average ± SEM (see Appendix 1 for ITC analysis).
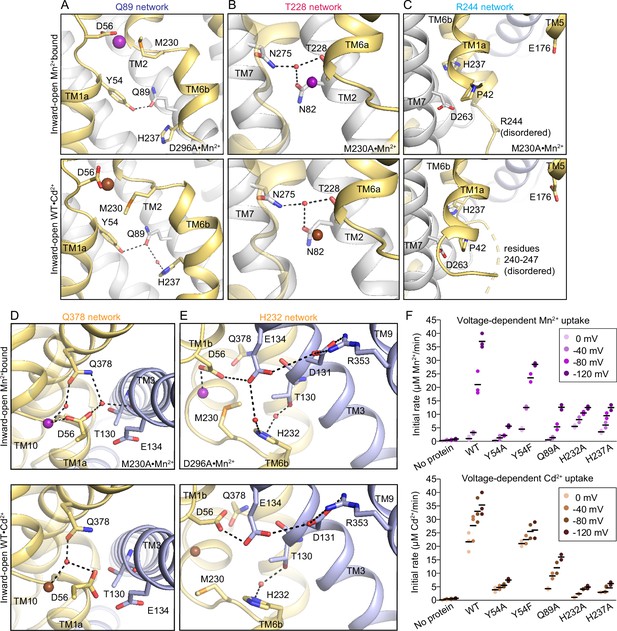
Comparison of the gating networks in the inward-open Mn2+ and Cd2+-bound structures.
(A–E) Structural comparisons of the five networks of polar residues with Mn2+ (top; M230A•Mn2+ or D296A•Mn2+ as indicated) or Cd2+ (WT•Cd2+; bottom) bound at the orthosteric site. Inward-open conformations of Mn2+ and Cd2+ structures show identical arrangement of residues for the Q89 (A), T228 (B), and R244 (C) networks. In the outer-gate Q378 network (D), D56 does not coordinate the Cd2+ directly, but instead coordinates a water that interacts with Cd2+ and Q378. Due to its reorientation, D56 does not hydrogen-bond to T130. The H232 network (E) is conserved except that the interaction between E134 and D56 takes a different orientation in the Cd2+-bound structure. Some of the top (Mn2+-bound) panels are reproduced from Figure 4 for ease of comparison. (F) Initial metal uptake rates for DraNramp variants at different membrane potential (ΔΨ=0 to −120 mV; n=2–3; each data point is represented in the scatter plots and black bars are the mean values). The metal ion concentration was 750 μM, and the pH 7 on both sides of the membrane. Y54A, H232A, H237A, and Q89A strongly reduced the initial transport rate, whereas Y54F only moderately reduced it. The overall trends are similar for both metals. Mn2+ transport showed higher voltage dependence compared to Cd2+. Mn2+ data from Figure 3 are replotted here for ease of comparison. Corresponding time traces are plotted in Figure 1—figure supplement 4.
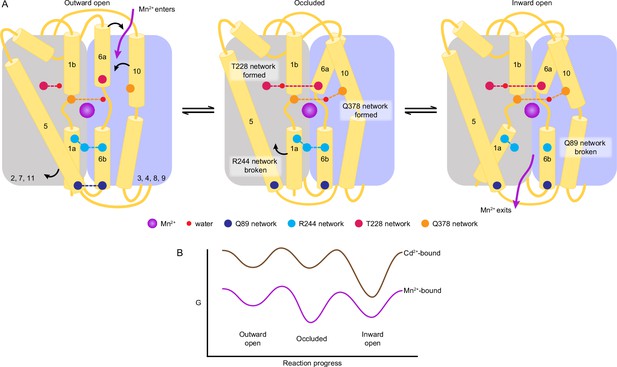
A structure-guided model of the conformational cycle and thermodynamic landscape of metal transport by Nramps.
(A) After Mn2+ enters through the outer vestibule between TM6a and TM10 in the outward-open state, a bulk conformation change closes the outer gate. The occluded conformation arises though rearrangements of TM6a and TM10 facilitated by formation of the T228 and Q378 networks, respectively. The inner gate partially opens in the occluded state as the R244 network breaks and TM5 moves. To achieve the inward-open conformation, disruption of the Q89 network frees TM1a to swing up to fully open the inner vestibule for Mn2+ release into the cytosol. (B) Our data indicate that the most stable Mn2+-bound state is the occluded state, and the three main states are readily accessible to facilitate transport. In contrast, Cd2+ binding stabilizes the inward-open state.
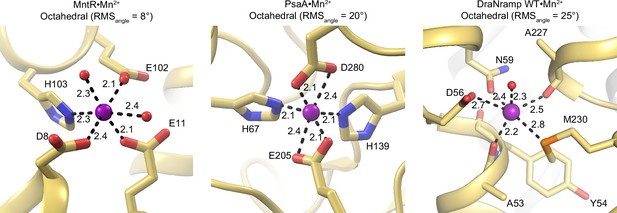
Comparison of coordination spheres of different Mn2+-binding proteins: MntR, a Mn2+ regulator (PDB ID: 1ON1) (Glasfeld et al., 2003); PsaA, the solute-binding protein domain of Mn2+-transporting ATP-binding cassette transporter (PDB ID: 3ZTT) (Couñago et al., 2014); and the DraNramp WT•Mn2+ occluded structure (this work).
In all structures, Mn2+ binds in an octahedral coordination. The geometry is more distorted (RMSangle=25°) and bond distances are longer in DraNramp, perhaps to enable Mn2+ transport and the associated protein dynamics. In comparison, the geometry is close to ideal (RMSangle=8°) and bond distances shorter in MntR, where Mn2+ binding, but not transport, is essential for regulating downstream signaling.
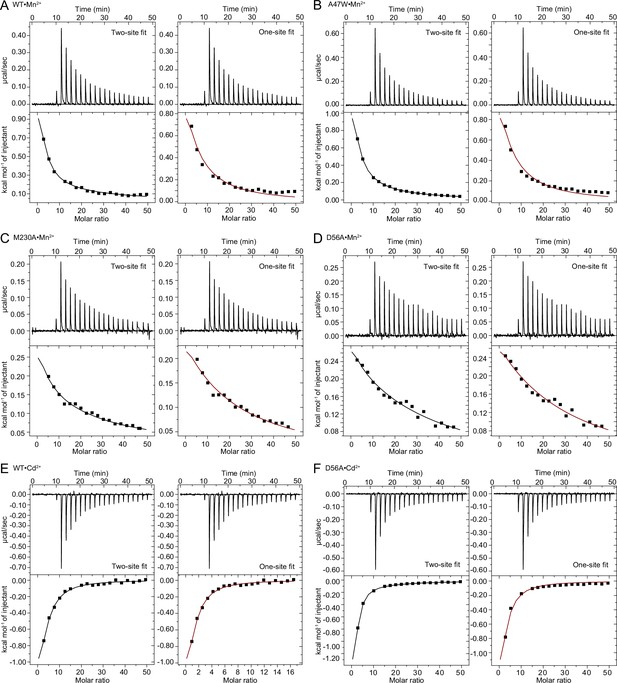
Comparison of one-site and two-site fit to ITC binding isotherms for each case where both types of fits gave results.
We show fits to a two-site sequential binding model (fixed n=2; left) or a one-site model with a fixed n=1 (right) for each case where both types of fits gave a result (Appendix 1—tables 1 and 2). For constructs•ligand pairs WT•Mn2+ (A), A47W•Mn2+ (B), M230A•Mn2+ (C), and D56A•Cd2+ (F), the two-site fit is better than the one-site fit, which corroborates findings from the crystal structures. For pairs WT•Cd2+ (E) and D56A•Mn2+ (D), the fits are similar, and we used additional biochemical and structural information to select the most appropriate fit.
Videos
Conformational rearrangement and distinct coordination geometry adopted by DraNramp during Mn2+ import.
The global conformational changes are highlighted first, with cartoon representations of each of the six structures in the Mn2+ transport cycle starting from the outward-open Mn2+-bound conformation rotating through the occluded Mn2+-bound, inward-open Mn2+-bound, and then the metal-free states transitioning back to outward-open Mn2+-bound form. The second set of scenes then focusses on the orthosteric site, cycling through the structures in the same order to illustrate the distinct coordination geometries of the bound Mn2+, and the corresponding positions of its interacting ligands (protein residues and water) in the metal-free states.
Tables
Data collection and refinement statistics for four new DraNramp structures.
StructureConformationBound metal ion substratePDB ID | WTsoakOccluded none8E5V | WT•Mn2+OccludedMn2+8E60 | M230A•Mn2+Inward openMn2+8E6I | WT•Cd2+Inward openCd2+8E6M |
---|---|---|---|---|
Data Collection | ||||
Beamline | GMCA 23IDB | GMCA 23IDB | GMCA 23IDB | NECAT 24IDC |
Wavelength (Å) | 1.033 | 1.033 | 1.033 | 0.984 |
Resolution range (Å) | 41.23–2.36 (2.44–2.36) | 41.28–2.38 (2.46–2.38) | 45.32–2.52 (2.61–2.52) | 45.54–2.48 (2.57–2.48) |
Space group | P 2 21 21 | P 2 21 21 | P 2 21 21 | P 2 21 21 |
Unit cell (a, b, c) | 58.95, 71.04, 98.77 | 59.08, 71.10, 98.75 | 58.67, 71.35, 98.59 | 59.14, 71.37, 99.05 |
Unit cell (α, β, γ) | 90, 90, 90 | 90, 90, 90 | 90, 90, 90 | 90, 90, 90 |
Number of crystals | 1 | 1 | 3 | 1 |
Total reflections | 58744 (5928) | 57472 (5733) | 146077 (14913) | 76829 (7275) |
Unique reflections | 17477 (1718) | 16468 (1646) | 14548 (1427) | 15351 (1507) |
Redundancy | 3.4 (3.4) | 3.5 (3.5) | 10.0 (10.4) | 5.0 (4.8) |
Completeness (%) | 98.71 (98.85) | 95.11 (96.92) | 99.90 (99.79) | 99.03 (99.47) |
Mean I/σ (I) | 8.89 (0.97) | 8.92 (0.89) | 8.47 (0.75) | 9.90 (1.12) |
Wilson B-factor | 49.97 | 50.55 | 54.29 | 49.52 |
Rmerge | 0.106 (1.292) | 0.109 (1.241) | 0.269 (2.475) | 0.158 (1.626) |
Rmeas | 0.127 (1.511) | 0.127 (1.447) | 0.284 (2.603) | 0.178 (1.831) |
Rpim | 0.067 (0.759) | 0.063 (0.722) | 0.090 (0.800) | 0.078 (0.816) |
CC1/2 | 0.99 (0.37) | 0.99 (0.39) | 0.98 (0.34) | 0.99 (0.34) |
Refinement | ||||
Resolution range (Å) | 41.23–2.36 (2.44–2.36) | 41.28–2.38 (2.46–2.38) | 45.32–2.52 (2.61–2.52) | 45.54–2.48 (2.57–2.48) |
No. reflections | 17441 (1714) | 16438 (1636) | 14547 (1425) | 15291 (1507) |
No. reflections in Rfree | 1743 (171) | 1642 (164) | 1454 (143) | 1530 (151) |
Rwork | 0.217 (0.340) | 0.207 (0.316) | 0.225 (0.313) | 0.202 (0.319) |
Rfree | 0.245 (0.350) | 0.259 (0.358) | 0.266 (0.349) | 0.250 (0.354) |
Number of atoms | 3449 | 3385 | 3451 | 3321 |
Protein | 2945 | 2933 | 2934 | 2905 |
Ligand | 443 | 405 | 448 | 362 |
Water | 61 | 47 | 69 | 54 |
Protein Residues | 392 | 393 | 392 | 388 |
Ramachandran plot | ||||
Favored (%) | 98.46 | 98.47 | 98.21 | 98.96 |
Allowed (%) | 1.54 | 1.53 | 1.79 | 1.04 |
Outliers (%) | 0 | 0 | 0 | 0 |
Rotamer outliers (%) | 0.33 | 1.00 | 1.01 | 1.01 |
Clashscore | 8.25 | 8.97 | 7.15 | 5.57 |
RMS (bonds) | 0.002 | 0.002 | 0.002 | 0.002 |
RMS (angles) | 0.43 | 0.46 | 0.43 | 0.46 |
Average B-factor | 65.12 | 64.98 | 66.61 | 64.82 |
Protein | 63.36 | 63.23 | 65.04 | 62.68 |
Ligand | 77.99 | 78.48 | 77.70 | 83.11 |
Water | 56.34 | 58.24 | 61.33 | 57.45 |
No. of TLS groups | 9 | 8 | 3 | 3 |
-
Values in parentheses are for highest-resolution shell. Data for M230A•Mn2+ merge reflections from three crystals. Data for the other structures were obtained from a single crystal. See Supplementary file 1a for details on soaking or co-crystallization procedures for bound metal ion substrates.
Reagent type (species) or resource | Designation | Source or reference | Identifiers | Additional information |
---|---|---|---|---|
Gene (Deinococcus radiodurans) | DraNramp | Genomic DNA | Uniprot: Q9RTP8 | |
Strain, strain background (Escherichia coli) | C41(DE3) | Lucigen | 60442–1 | Chemically competent cells |
Strain, strain background (Escherichia coli) | DH5α | Invitrogen | 18265–017 | Chemically competent cells |
Recombinant DNA reagent | pET21a | Novagen | 69740–3 | Vector backbone for cloning DraNramp |
Chemical compound, drug | n-Dodecyl-β-D-Maltopyranoside | Anatrace | D310S | 1% for solubilizing membrane, 0.03% used in wash buffer |
Chemical compound, drug | n-Decyl-β-D-Maltopyranoside | Anatrace | D322S | 0.1% used in exchange buffer |
Chemical compound, drug | Lauryl maltose neopentyl glycol | Anatrace | NG310 | 0.01% in elution buffer, 0.003% used in SEC buffer |
Chemical compound, drug | Monoolein | Anatrace | LCP18 | 1:1.5 (protein: monoolein) for LCP crystallization |
Chemical compound, drug | Fura-2 Pentapotassium Salt, cell impermeant | Life Technologies | F-1200 | 5 mM mixed with in proteoliposome |
Chemical compound, drug | 1-Palmitoyl-2-oleoyl-sn-glycero-3-phospho-(1'-rac-glycerol) (POPG) | Avanti Polar Lipids | 850457 C | lipid mixture (3 POPE: 1 POPG) and protein in 400:1 ratio for proteoliposome preparation |
Chemical compound, drug | 1-Palmitoyl-2-oleoyl-sn-glycero-3-phosphoethanolamine (POPE) | Avanti Polar Lipids | 840757 C | lipid mixture (3 POPE: 1 POPG) and protein in 400:1 ratio for proteoliposome preparation |
Chemical compound, drug | Valinomycin | Sigma-Aldrich | V0627 | Creates membrane potential by transporting K+ in proteoliposome assay |
Commercial assay, kit | Ni Sepharose High Performance Resin | Cytiva | 95055–838 | IMAC resin |
Commercial assay, kit | Superdex 200 10/300 GL | Cytiva | 89497–272 | SEC column |
Commercial assay, kit | PD-10 Desalting Column | Cytiva | 95017–001 | Buffer exchange |
Software, algorithm | XDS | PMID:20124692 | RRID:SCR_015652 | Data Porcessing |
Software, algorithm | PHASER | PMID:19461840 | RRID:SCR_014219 | Model Builiding |
Software, algorithm | PHENIX | PMID:22505256 | RRID:SCR_014224 | Refinement |
Software, algorithm | coot | PMID:20383002 | RRID:SCR_014222 | Refinement and model building |
Software, algorithm | PyMOL | Schrödinger | RRID:SCR_000305 | Figure making |
Software, algorithm | HMMER | hmmer.org | RRID:SCR_005305 | Collected sequences with jackhmmer and aligned with hmmalign |
Software, algorithm | MUSCLE | PMID:15318951 | RRID:SCR_011812 | Aligning sequences |
Software, algorithm | RAxML-NG | PMID:31070718 | RRID:SCR_022066 | Tree building |
Software, algorithm | PropKa | PMID:26596171 | Identifying protonation states | |
Software, algorithm | CHARMM-GUI | PMID:25130509 | Building MD system | |
Software, algorithm | NAMD | PMID:20675161 | RRID:SCR_014894 | Running MD simulations |
Software, algorithm | mdtraj | PMID:26488642 | Analyzing MD data | |
Software, algorithm | VMD | PMID:8744570 | RRID:SCR_001820 | Analyzing MD data |
ITC analysis of all Mn2+ binding isotherms, with chosen model and values shaded.
Protein construct | Two-site sequential binding model (fixed n=2) | One-site fit with fixed n=1 | ||||||||||
---|---|---|---|---|---|---|---|---|---|---|---|---|
Kd1(µM) | ΔH1(kcal/mol) | -TΔS1(kcal/mol) | ΔG1(kcal/mol) | Kd2(µM) | ΔH2(kcal/mol) | -TΔS2(kcal/mol) | ΔG2(kcal/mol) | Kd(µM) | ΔH(kcal/mol) | -TΔS(kcal/mol) | ΔG(kcal/mol) | |
WT | 220 | 3.2 | –8.1 | -5 | 961 | 5.4 | –9.2 | –3.8 | 650 | 8.8 | –13.1 | –4.3 |
125 | 5.7 | –11.1 | –5.4 | 2700 | 17.1 | –20.5 | –4.6 | 380 | 12.6 | –16.9 | –4.3 | |
220 | 8 | –12.8 | -5 | 2250 | 13.7 | –17.3 | –3.4 | 500 | 15.4 | –19.6 | –4.2 | |
190±30 | 5.6±1.3 | –10.6±1.4 | –5.1±0.1 | 1970±520 | 12.1±3.3 | –15.7±3.1 | –4.0±0.3 | 510±80 | 12.3±1.9 | –16.5±1.8 | –4.2±0.03 | |
A47W | 130 | 6.3 | –11.3 | -5 | 3100 | 20.6 | –23.8 | –3.2 | 380 | 13.6 | –18.1 | –4.5 |
120 | 6.2 | –11.3 | –5.1 | 1800 | 11.4 | –14.9 | –3.5 | 300 | 11.9 | –16.6 | –4.7 | |
125±5 | 6.2±0.5 | –11.3±0.0 | –5.0±0.1 | 2450±650 | 16±4.5 | –19.3±4.4 | –3.3±0.1 | 340±40 | 12.7±0.8 | –17.3±0.7 | –4.6±0.1 | |
D56A | 305 | 2.4 | –7.1 | –4.7 | 4800 | 20.4 | –23.5 | –3.1 | 2200 | 16.3 | –19.6 | –3.3 |
140 | 1.8 | –6.8 | -5 | 2700 | 22.6 | –25.9 | –3.3 | 2500 | 22.6 | –26.2 | –3.6 | |
230±80 | 2.1±0.3 | –6.9±0.1 | –4.8±0.1 | 3800±1,100 | 21.5±1.1 | –24.7±0.1 | –3.2±0.1 | 2350±150 | 19.4±3 | –22.9±3.3 | –3.4±0.1 | |
M230A | 280 | 3 | –7.7 | –4.7 | 3300 | 14.9 | –18.1 | –3.2 | 1500 | 13.3 | –16.9 | –3.6 |
150 | 2.9 | -8 | –5.1 | 5800 | 29.9 | –33.1 | –3.2 | 970 | 12.5 | –16.3 | –3.8 | |
215±65 | 2.9±0.05 | –7.8±0.1 | –4.9±0.2 | 4600±1,300 | 22.4±7.5 | –25.6±7.5 | –3.2±0 | 1240±270 | 12.9±0.4 | –16.6±0.3 | –3.7±0.1 | |
G223W | Did not fit | 460 | 5.8 | –10.1 | –4.3 | |||||||
430 | 3 | –7.4 | –4.4 | |||||||||
440±15 | 4.4±1.4 | –8.7±1.3 | –4.3±0.1 | |||||||||
D296A | Did not fit | 430 | 8.9 | –13.4 | –4.5 | |||||||
320 | 9.5 | –14.3 | –4.8 | |||||||||
370 | 10.1 | –14.6 | –4.5 | |||||||||
370±30 | 9.5±0.3 | –14.1±0.3 | –4.6±0.1 | |||||||||
D369A | Did not fit | 390 | 9.5 | –14 | –4.5 | |||||||
480 | 8.9 | –13.4 | –4.5 | |||||||||
380 | 8.3 | –12.8 | –4.5 | |||||||||
420±30 | 8.9±0.3 | –13.4±0.3 | –4.5±0 | |||||||||
A47W-D296A | Did not fit | 300 | 6.1 | –10.7 | –4.6 | |||||||
210 | 13.2 | –17.5 | –4.3 | |||||||||
255±45 | 9.6±3.5 | –14.1±3.4 | –4.4±0.1 | |||||||||
A47W-D369A | Did not fit | 210 | 7.5 | –12.5 | -5 | |||||||
400 | 6.5 | –11.1 | –4.6 | |||||||||
300±95 | 7±0.5 | –11.8±0.7 | –4.8±0.1 | |||||||||
D56A-D296A | Did not fit | 170 | 5.3 | –10.4 | –5.1 | |||||||
330 | 4.8 | –9.5 | –4.7 | |||||||||
250±80 | 5.0±0.2 | –9.9±0.4 | –4.9±0.2 | |||||||||
D56A-D369A | Did not fit | 280 | 4.9 | –9.5 | –4.6 | |||||||
145 | 4 | –8.9 | –4.9 | |||||||||
210±70 | 4.4±0.4 | –9.2±0.3 | –4.7±0.1 | |||||||||
M230A-D296A | Did not fit | 320 | 7.1 | –11.6 | –4.5 | |||||||
140 | 3.7 | –8.9 | –5.2 | |||||||||
230±90 | 5.4±1.7 | –10.2±1.3 | –4.8±0.3 | |||||||||
M230A-D369A | Did not fit | 750 | 11.5 | –15.4 | –3.9 | |||||||
790 | 7.9 | –11.9 | -4 | |||||||||
770±20 | 9.7±1.8 | –13.6±1.7 | –3.9±0.05 |
-
Appendix 1—table 1—source data 1
Source files (Origin files) of ITC experiments of manganese binding to each DraNramp construct.
- https://cdn.elifesciences.org/articles/84006/elife-84006-app1-table1-data1-v2.zip
ITC analysis of all Cd2+-binding isotherms, with chosen model and values shaded.
Protein construct | Two-site sequential binding model (fixed n=2) | One-site fit with fixed n=1 | ||||||||||
---|---|---|---|---|---|---|---|---|---|---|---|---|
Kd1(µM) | ΔH1(kcal/mol) | -TΔS1 (kcal mol–1) | Kd1(µM) | ΔH1(kcal/mol) | ΔH2 (kcal mol–1) | Kd1(µM) | ΔH1(kcal/mol) | Kd(µM) | Kd1(µM) | ΔH1(kcal/mol) | ΔG (kcal mol–1) | |
WT | 85 | –4.5 | –0.9 | –5.5 | 260 | –2.6 | -2 | –4.6 | 105 | –2.3 | –2.9 | –5.2 |
50 | –4.7 | –0.9 | –5.6 | 220 | –4.1 | –0.6 | –4.7 | 105 | -9 | 3.5 | –5.5 | |
30 | –2.9 | –2.9 | –5.8 | 180 | –4.7 | –0.3 | -5 | 115 | –7.6 | 2.1 | –5.5 | |
55±15 | –4.0±0.5 | –1.6±0.6 | –5.6±0.1 | 220±20 | –3.8±0.6 | –1.0±0.5 | –4.8±0.1 | 110±3 | 6±2 | –2.8±1.9 | –5.4±0.1 | |
A47W | 220 | –4.4 | –0.2 | –4.6 | 130 | 0.6* | –5.6 | -5 | 165 | –4.8 | –0.2 | -5 |
Did not fit | 140 | –3.3 | –1.7 | -5 | ||||||||
150±10 | –4.0±0.7 | –0.9±0.5 | –5.0±0.0 | |||||||||
D56A | 100 | –6.1 | 0.6 | –5.5 | 3000 | –11.2 | 7.7 | –3.5 | 190 | –9.2 | 4.1 | –5.1 |
55 | –4.5 | –1.2 | –5.7 | 1500 | –6.2 | 2.3 | –3.9 | 130 | –6.9 | 1.5 | –5.4 | |
80±20 | –5.3±0.8 | –0.6±0.9 | –5.6±0.1 | 2250±750 | –8.7±2.5 | 5.0±0.5 | –3.7±0.1 | 160±30 | –8.0±1 | –2.8±1.3 | –5.2±0.1 | |
M230A | Did not fit | 142 | –6.1 | 0.9 | –5.2 | |||||||
182 | –3.1 | –1.7 | –4.8 | |||||||||
160±20 | –4.6±1.5 | –0.4±0.3 | –5.0±0.2 | |||||||||
G223W | No Binding | |||||||||||
D296A | Did not fit | 122 | –3.5 | –1.8 | –6.8 | |||||||
120 | –3.7 | –1.5 | –5.2 | |||||||||
120±1 | –3.6±0.1 | –1.6±0.1 | –6.0±0.8 | |||||||||
D369A | 100 | –1.6 | –3.5 | –5.1 | 5350 | 2.8* | –5.9 | –3.1 | 80 | –1.4 | –4.1 | –5.5 |
90 | –2.5 | –2.6 | –5.7 | 4850 | 5* | -8 | -3 | 60 | –2.1 | –3.8 | –5.9 | |
95±5 | –5.3±0.8 | –3.1±0.4 | –5.4±0.1 | 5100±250 | 3.9±1.0* | –6.9±1.0 | –3.0±0.1 | 70±10 | –1.7±0.3 | –3.9±0.1 | –5.7±0.1 | |
A47W-D296A | No Binding | |||||||||||
A47W-D369A | No Binding | |||||||||||
D56A-D296A | No Binding | |||||||||||
D56A-D369A | No Binding | |||||||||||
M230A-D296A | No Binding | |||||||||||
M230A-D369A | No Binding |
-
*
Positive values of ΔH corresponding to an exothermic mode of Cd2+ binding to protein indicates inappropriate fit
-
Appendix 1—table 2—source data 1
Source files (Origin files) of ITC experiments of cadmium binding to each DraNramp construct.
- https://cdn.elifesciences.org/articles/84006/elife-84006-app1-table2-data1-v2.zip
Additional files
-
Supplementary file 1
Additional tables with methodological details and results from data analyses.
(a) Construct, precipitant, and soaking solutions used for each structure. (b) Data collection and refinement statistics for the four supporting new DraNramp structures. (c) Cα RMSD in Å for all DraNramp structure pairs (number of aligned residues in parentheses). (d) Data collection statistics for anomalous maps. (e) Distances to metal (Å) for coordinating atoms at the orthosteric site. (f) Coordination number and geometry of metal ions in the orthosteric site. (g) Binding affinity of metals to various DraNramp constructs. (h) Primers for Mutagenesis (5’ to 3’ sequence). (i) Summary of molecular dynamics simulations.
- https://cdn.elifesciences.org/articles/84006/elife-84006-supp1-v2.docx
-
MDAR checklist
- https://cdn.elifesciences.org/articles/84006/elife-84006-mdarchecklist1-v2.docx
-
Appendix 1—table 1—source data 1
Source files (Origin files) of ITC experiments of manganese binding to each DraNramp construct.
- https://cdn.elifesciences.org/articles/84006/elife-84006-app1-table1-data1-v2.zip
-
Appendix 1—table 2—source data 1
Source files (Origin files) of ITC experiments of cadmium binding to each DraNramp construct.
- https://cdn.elifesciences.org/articles/84006/elife-84006-app1-table2-data1-v2.zip