Cholinergic modulation shifts the response of CA1 pyramidal cells to depolarizing ramps via TRPM4 channels with potential implications for place field firing
Figures
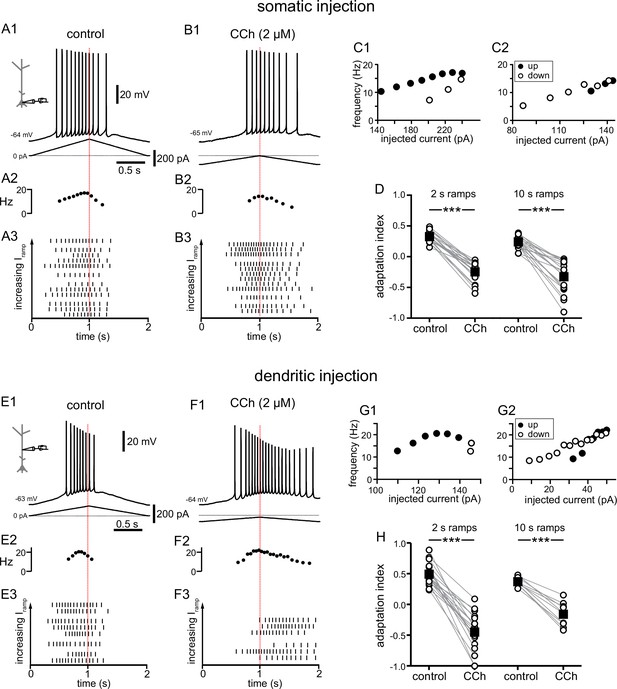
Carbachol shifts the center of mass of firing in response to depolarizing current ramps.
(A) Somatic recording, control. (A1) Voltage trace for a two second triangular current ramp (represented below) injected in the soma. (A2) Instantaneous frequency for example in A1 as it changes along the ramp. (A3) Raster plot of different trials recorded from the same example neuron in A1. The trials are ordered by the amplitude of the peak injected current, from lowest (bottom) to highest (top); trials with the same amount of current injection are clustered together. (B) Somatic recording, carbachol. (B1, B2, and B3) Same as A1, A2, and A3, but during superfusion with 2 µM CCh. (C1) Instantaneous frequency for example in A1, as it changes with injected current. Filled circles represent firing on the up-ramp; open circles, firing on the down-ramp. (C2) Instantaneous frequency for example in B1. A, B, and C are recordings from the same cell. (D) Summary data of the adaptation index for somatic recordings, before and during CCh, for two second ramps (n=21) and ten second ramps (n=19). Open circles connected by gray lines represent indices for individual cells, before and during CCh. Black squares with error bars represent group averages ± SEM. In control, all adaptation indices are positive, indicating more action potentials on the up-ramp. In CCh, most adaptation indices are negative, indicating more action potentials on the down-ramp. (E) Dendritic recording, control. (E1) Voltage trace for a two second triangular current ramp (represented below) injected in the dendrite, approximately 200 µm from the soma. (E2) Instantaneous frequency for E1 as it changes along the ramp. (E3) Raster plot of different trials recorded from same example neuron in E1. (F) Dendritic recording, carbachol. (F1, F2, and F3) Same as E1, E2, and E3, but during superfusion with 2 µM CCh. (G1) Instantaneous frequency for example in E1, as it changes with injected current. (G2) Instantaneous frequency for example in F1. E, F, and G are from the same cell. (H) Summary data of the adaptation index for dendritic ramps, before and during CCh, for two second ramps (n=19) and ten second ramps (n=10). *** p<0.0005, paired t-test. Red dotted lines in the left panels mark the middle of the current injection ramp. Source data in "Figure 1—source data 1". See also Figure 1—figure supplement 1 and Figure 1—figure supplement 2.
-
Figure 1—source data 1
Carbachol shifts the center of mass of firing in response to depolarizing current ramps.
- https://cdn.elifesciences.org/articles/84387/elife-84387-fig1-data1-v2.xlsx

Plots of instantaneous frequency versus current injection showing different effects of carbachol on firing rate adaptation.
(A and B) Two examples of somatic recordings where the firing rate adaptation in control is reversed in the presence of CCh, such that the clockwise hysteresis becomes counter-clockwise. (C and D) Two examples of somatic recordings where the firing rate adaptation in control is removed in the presence of CCh, such that the hysteresis disappears, and the motion becomes linear. Filled circles represent the firing frequencies on the up-ramp and open circles represent firing frequencies on the down-ramp.
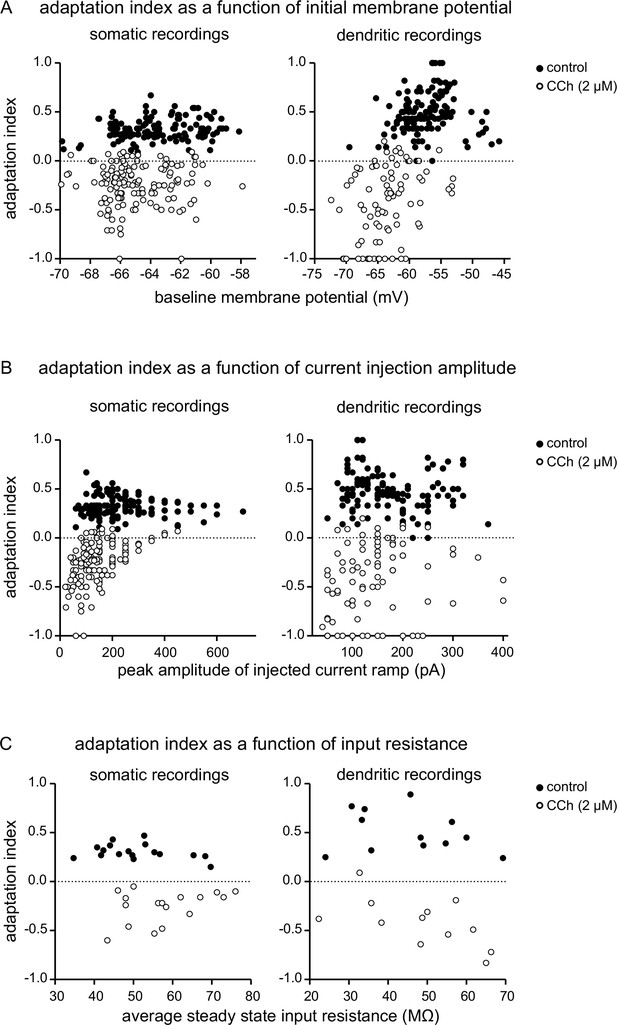
Effects of the membrane potential, injected current and input resistance on the adaptation index.
(A) Adaptation index as a function of membrane potential. Index values for each trial for all neurons are plotted against the membrane potential measured just before the current injection ramp for that trial, both for somatic recordings (n=132 in control conditions, filled circles; n=144 in the presence of CCh 2 µM, open circles) and for dendritic recordings (n=135 in control conditions, filled circles; n=87 during CCh, open circles). (B) Adaptation index as a function of the peak injected current. Index values for each trial for all recorded neurons are shown plotted against the peak amplitude of the triangular-shaped current injection for that trial, for both somatic and dendritic recordings (values for n and symbols are the same as in A). (C) Adaptation index as a function of input resistance. Average adaptation index values per cell for both somatic recordings (n=17) and dendritic recordings (n=12) are plotted against the average steady state input resistance measured during control conditions (filled circles) and during perfusion with CCh (open circles). Source data in "Figure 1—figure supplement 2—source data 1".
-
Figure 1—figure supplement 2—source data 1
Carbachol shifts the center of mass of firing in response to depolarizing current ramps.
- https://cdn.elifesciences.org/articles/84387/elife-84387-fig1-figsupp2-data1-v2.xlsx
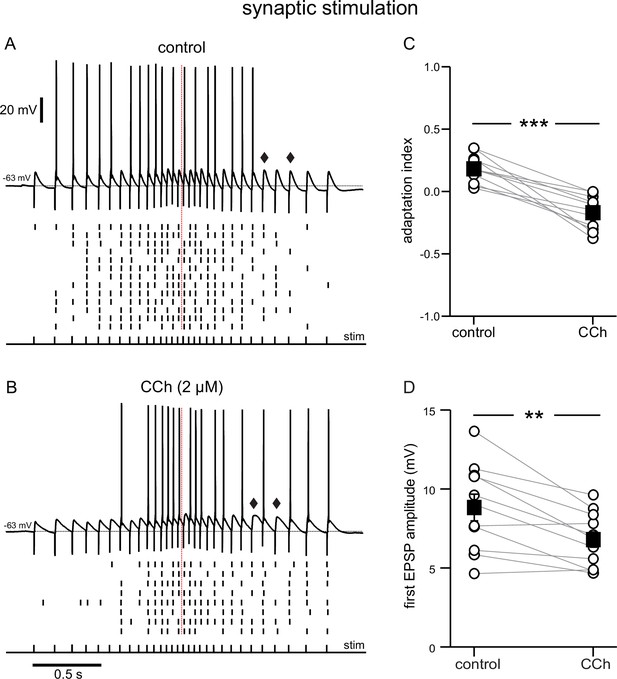
Cholinergic modulation of the response to electrical stimulation of Schaffer collaterals, recorded at the soma.
(A) Top, synaptic response in control conditions to an input frequency that increases and decreases symmetrically, from 6.7 to 25 Hz, as denoted by the pulses, labeled stim. Bottom, Raster plot of multiple trials repeated at the same stimulation intensity. (B) Same as A during superfusion of 2 µM CCh. A and B are from the same neuron, and at the same stimulation intensity. Diamonds in A and B point to a longer EPSP decay time in the presence of CCh. (C) Summary data of the adaptation index for synaptic ramps, before and during CCh. (D) Summary data of the average EPSP amplitude measured during trials where the cells did not fire in response to the first stimulus pulse, before and during CCh. In both cases, open circles connected by gray lines represent indices for individual cells; black squares with error bars represent group averages ± SEM *** p<0.0005, ** p=0.002, paired t-test. Red dotted lines in the panels on the left mark the middle of the synaptic stimulation. Source data in "Figure 2—source data 1".
-
Figure 2—source data 1
Cholinergic modulation of the response to electrical stimulation of Schaffer collaterals, recorded at the soma.
- https://cdn.elifesciences.org/articles/84387/elife-84387-fig2-data1-v2.xlsx
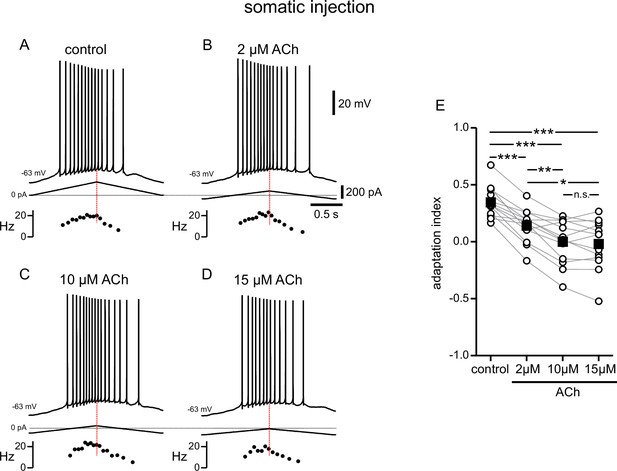
Modulation of responses to symmetric ramps by acetylcholine is concentration dependent.
(A) Voltage trace recorded in the soma for a two second triangular current ramp injected in the soma in control. Current traces and instantaneous frequency plots are just below the voltage traces. (B–D) Same as A, during superfusion with 2 µM ACh (B), 10 µM ACh (C), and 15 µM ACh (D). A through D are from the same cell. (E) Summary data of the adaptation index for control and the three concentrations of ACh, applied in order of increasing concentration (n=13). Open circles connected by gray lines represent indices for individual cells over all four conditions. Black squares with error bars represent group averages ± SEM. *** p<0.0005, ** p=0.001, * p=0.01, one-way repeated measures ANOVA with a Greenhouse-Geisser correction and post hoc pairwise comparisons with a Bonferroni adjustment. Red dotted lines in the panels on the left mark the middle of the current injection ramp. Source data in "Figure 3—source data 1".
-
Figure 3—source data 1
Modulation of responses to symmetric ramps by acetylcholine is concentration dependent.
- https://cdn.elifesciences.org/articles/84387/elife-84387-fig3-data1-v2.xlsx
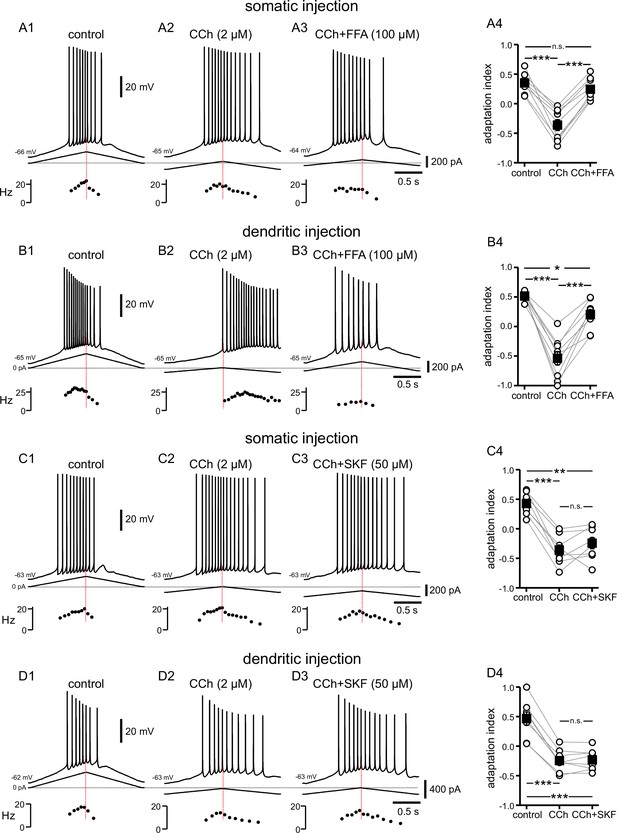
Cholinergic-associated shift in the timing of firing on ramp is mediated by TRP channels, but notably not TRPC channels.
(A) Somatic recordings for a two second triangular current ramp injected in the soma in control (A1), CCh (2 µM) (A2), and CCh + FFA (100 µM) (A3) applied subsequently to the same cell. Current traces and instantaneous frequency plots are just below the voltage traces. Summary data (A4) of the adaptation index for control, CCh, and CCh + FFA (n=9). (B) Same as in A, but for dendritic injection and recordings. Summary data (B4) of the adaptation index for control, CCh, and CCh + FFA (n=9). Open circles connected by gray lines represent indices for individual cells, over all three treatments. Black squares with error bars represent group averages ± SEM. (C) Somatic recordings for a two second triangular current ramp injected in the soma in control (C1), CCh (2 µM) (C2), and CCh + SKF96365 (50 µM) (C3) applied subsequently to the same cell. Current traces and instantaneous frequency plots are just below voltage traces. Red dotted line marks the middle of the ramp. Summary data (C4) of the adaptation index for control, CCh, and CCh + SKF96365 (n=8). (D) Same as in C, but for dendritic injection and recordings. Summary data (D4) of the adaptation index for control, CCh, and CCh + SKF96365 (n=8). *** p<0.0005, ** p=0.001, * p=0.02, n.s. not significant, one-way repeated measures ANOVA and post hoc pairwise comparisons with a Bonferroni adjustment. Source data in "Figure 4—source data 1".
-
Figure 4—source data 1
Cholinergic-associated shift in the timing of firing on ramp is mediated by TRP channels, but notably not TRPC channels.
- https://cdn.elifesciences.org/articles/84387/elife-84387-fig4-data1-v2.xlsx
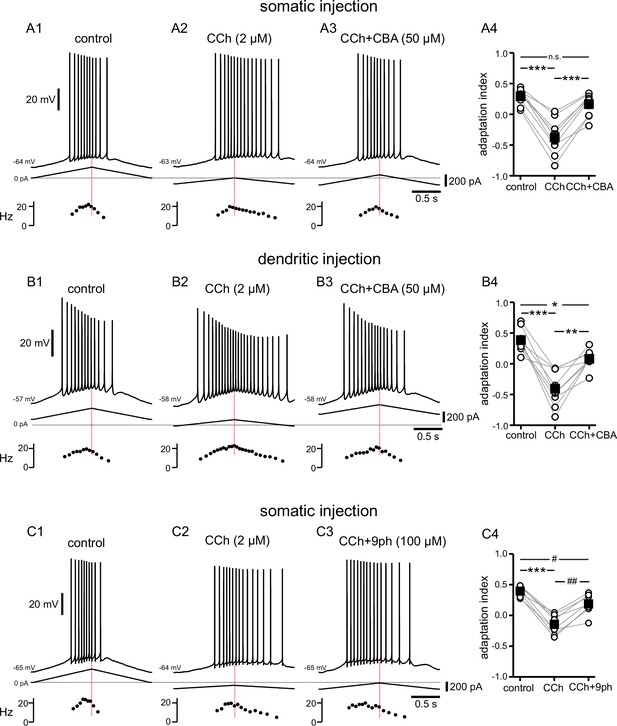
Cholinergic-mediated shift in the center of mass of firing is mediated by TRPM4 channels.
(A) Somatic recordings for a two second triangular current ramp injected in the soma in control (A1), CCh (2 µM) (A2), and CCh + CBA (50 µM) (A3) applied subsequently to the same cell. Current traces and instantaneous frequency plots are just below voltage traces. Red dotted line marks the middle of the ramp. Summary data (A4) of the adaptation index for control, CCh, and CCh + CBA (n=9). Open circles connected by gray lines represent indices for individual cells, over all three treatments. Black squares with error bars represent group averages ± SEM. (B) Same as in A, but for dendritic injection and recordings. Summary data (B4) of the adaptation index for control, CCh, and CCh + CBA (n=9). (C) Same as A, but for control (C1), CCh (2 µM) (C2), and CCh + 9-phenanthrol (100 µM) (C3) applied subsequently to the same cell. Summary data (C4) of the adaptation index for control, CCh, and CCh + 9-phenanthrol (n=8). *** p<0.0005, **p=0.002, * p=0.02, ## p=0.001, # p=0.03, n.s. not significant, one-way repeated measures ANOVA and post hoc pairwise comparisons with a Bonferroni adjustment. Source data in "Figure 5—source data 1".
-
Figure 5—source data 1
Cholinergic-associated shift in the timing of firing on ramp is mediated by TRP channels, but notably not TRPC channels.
- https://cdn.elifesciences.org/articles/84387/elife-84387-fig5-data1-v2.xlsx
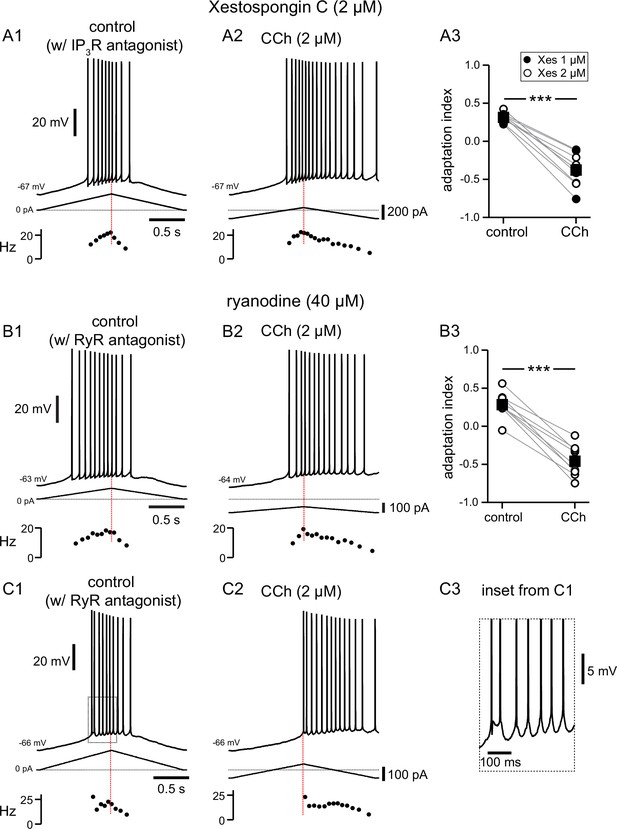
Pharmacological block of IP3Rs or RyRs has no effect on CCh-mediated shift in firing along ramp.
(A) Somatic recordings during intracellular dialysis with Xestospongin C (2 µM), for a two second triangular current ramp injected in the soma before (A1), and during superfusion with CCh (2 µM) (A2). Current traces and instantaneous frequency plots are just below voltage traces. Summary data (A3) of the adaptation index for Xestospongin C (1–2 µM) and CCh (n=9). Circles connected by gray lines represent ratios for individual cells, before and during CCh. Filled circles represent cells dialyzed with 1 µM Xestospongin C and open circles represent cells dialyzed with 2 µM Xestospongin C. Black squares with error bars represent group averages ± SEM. (B) Same as A, but intracellular dialysis is with ryanodine (40 µM). Summary data (B3) of the adaptation index for ryanodine and CCh (n=9). Individual cells represented by open circles. *** p<0.0005, paired t-test. In both sets of experiments, control data reflect the effect of either IP3R or RyR antagonist before the addition of CCh, because the dialysis started to be apparent quickly after break-in. Red dotted lines in the left panels mark the middle of the ramp. C1 and C2. Same as B1 and B2; C3 is an expanded version of the inset in C1. Source data in "Figure 6—source data 1".
-
Figure 6—source data 1
Pharmacological block of IP3Rs or RyRs has no effect on CCh-mediated shift in firing along ramp.
- https://cdn.elifesciences.org/articles/84387/elife-84387-fig6-data1-v2.xlsx
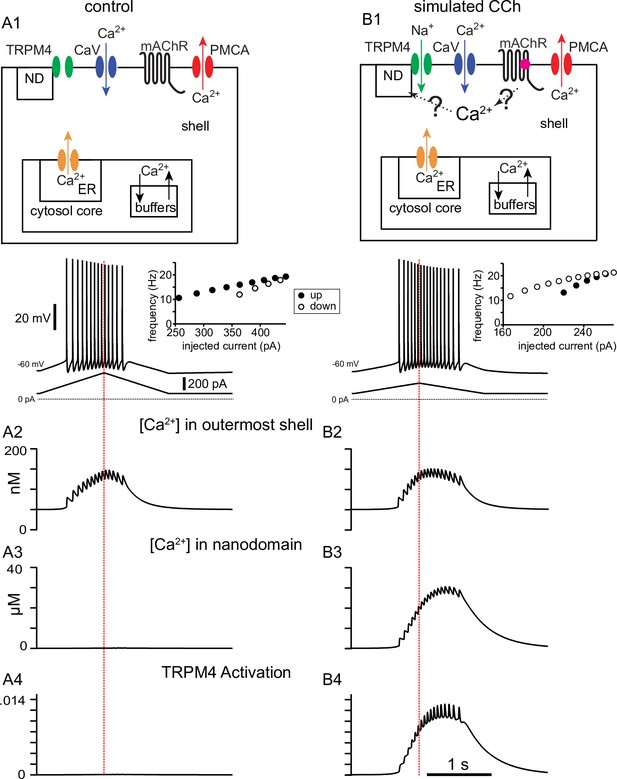
Simulated voltage responses to triangular current ramp injections, and underlying mechanisms.
(A) Simulated control response to triangular current ramp. (A1) Top. Simplified model calcium handling schematic. For simplicity, only one annulus (shell) is shown, and the rest are represented as the core. Ca2+ enters via voltage-gated Ca2+ channels (CaV) and is removed by the plasma membrane Ca2+ ATPase (PMCA) pump. Inside the cell, buffering and the endoplasmic reticulum (ER) are also modeled. The TRPM4 channel is not permeable to Ca2+ and senses [Ca2+] in a nanodomain (ND). In the control simulation, the nanodomain is contiguous with the cytosol. Bottom trace shows the voltage responses, plotted in the somatic compartment, to a triangular current ramp applied in the soma (shown below). (A2) Ca2+ concentration (in nM) in the outermost shell of the somatic compartment. (A3) The nanodomain Ca2+ concentration (same as the that of the outer shell in A2 in this case) is negligible when plotted in μM scale. (A4) TRPM4 activation is negligible in control. B. Simulated response to triangular current ramp during simulated application of CCh. (B1) As in A1 except for ligand (magenta dot) binding to the metabotropic acetylcholine receptor (mAChR), triggering Ca2+ influx into a nanodomain linked to the TRPM4 channel. The voltage trace shows that the center of mass of firing is shifted to later times. (B2) Ca2+ concentration (in nM) in the outermost shell of the somatic compartment. (B3) In contrast to control, the addition of a nanodomain with privileged Ca2+ access allows micromolar concentrations to be reached and sensed by TRPM4 channels. (B4) In contrast to control, TRPM4 is now activated during the ramp. Vertical dashed red line shows the peak of the current ramp.
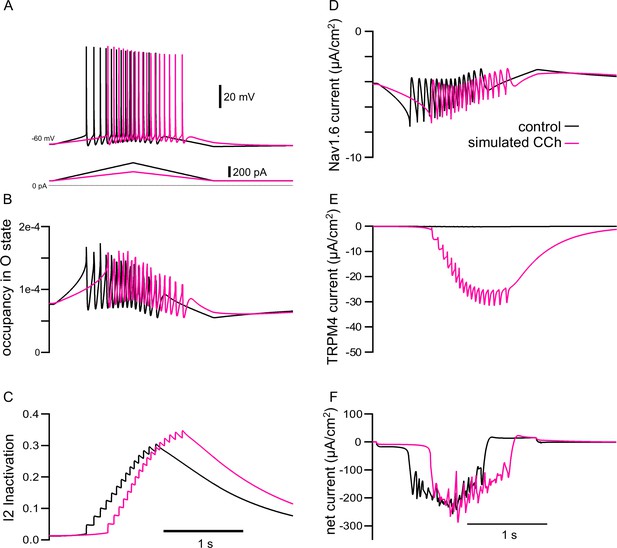
The model explains how simulated TRPM4 channel activation can reverse the adaptation mediated by long-term inactivation of NaV1.6 channels.
(A) Voltage traces from 7A1 and B1 overlaid; control (in black), CCh (in magenta). The triangular current injection ramps are shown below. (B) Occupancy of NaV1.6 channel in the open state (O) in the somatic compartment, with values during spikes removed. (C) Occupancy of NaV1.6 channel in the long-term inactivated state (I2). (D) Persistent NaV current (spiking values removed). (E) TRPM4 current. (F) Net ionic current. All values are shown for the somatic compartment.
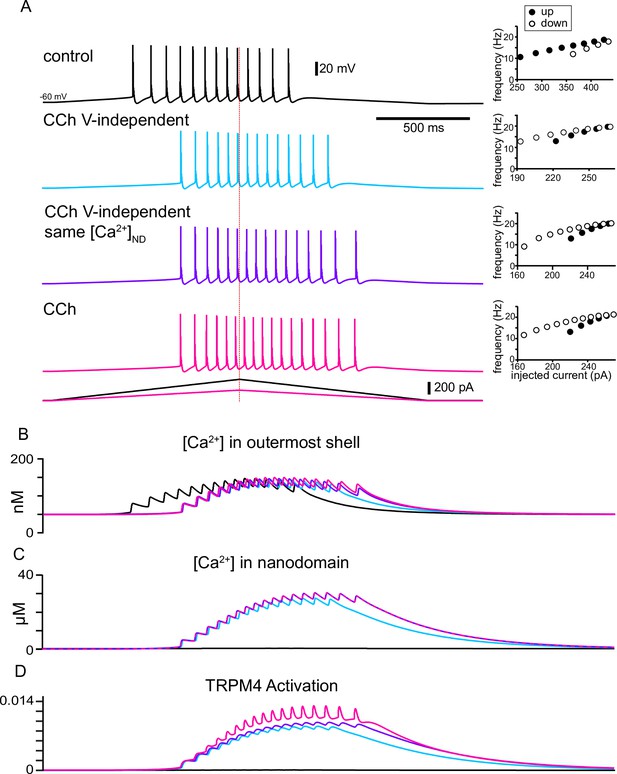
The model explains how activation of TRPM4 channels affects the firing pattern in response to triangular ramps that evoke similar firing frequencies.
(A) Simulated voltage traces (top) during a two second triangular current ramp (just below voltage traces). Insets show the reciprocal of the interspike interval (ISI) plotted as the instantaneous frequency at the ISI midpoint. Vertical dashed red line shows the peak of the current ramp. The slower frequencies at similar values of injected current and fewer ISIs on the down-ramp (open circles) versus the up-ramp (closed circles) in control show hysteresis due to adaptation mediated by long-term inactivation of the sodium channel. TRPM4 channel activation causes the center of mass of firing to be shifted to the right in all other traces compared to control because a nanodomain for TRPM4 activation with special access to Ca2+ was added in simulated CCh. The hysteresis is in the opposite direction because the activation of TRPM4 channels by Ca2+ accumulation obscures and overwhelms adaptation. In the light blue traces, the voltage-dependence of TRPM4 activation was not included, so there is less Ca2+ accumulation in the outer shell in (B) and in the nanodomain in (C), resulting in less activation of TRPM4 channels in (D). In order to isolate the effect of voltage-dependence from that of Ca2+ dependence, the Ca2+ waveforms in all nanodomains were recorded during the full CCh simulation shown in magenta, and played back into each nanodomain Ca2+ during the simulations shown in purple.
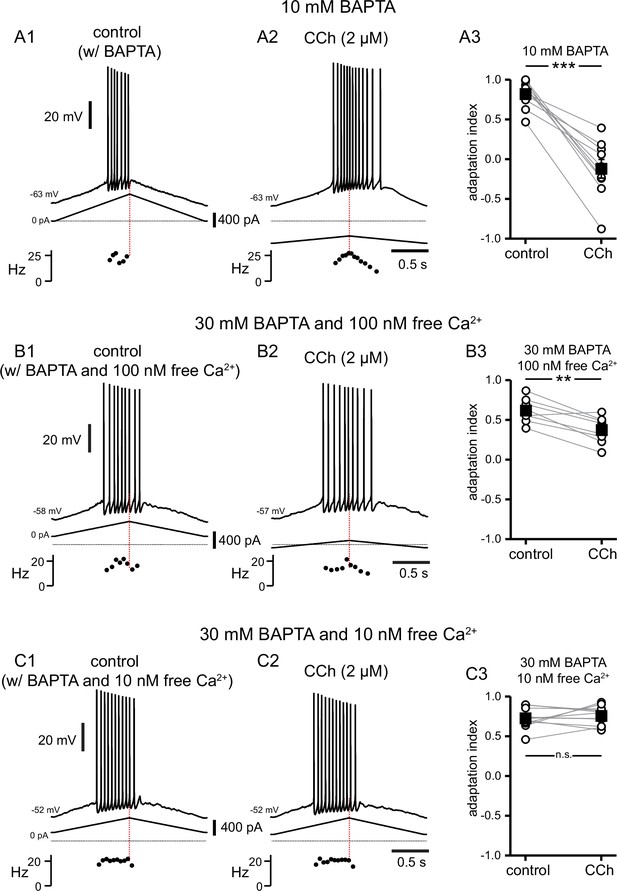
A high concentration of BAPTA is required to interfere with CCh-mediated shift in firing along ramp.
(A) Somatic recordings during intracellular dialysis with 10 mM BAPTA, for a two second triangular current ramp injected in the soma before (A1), and during superfusion with CCh (2 µM) (A2). Current traces and instantaneous frequency plots are just below voltage traces. Red dotted line marks the middle of the ramp. Summary data (A3) of the adaptation index for control and CCh (n=10). Open circles connected by gray lines represent ratios for individual cells, before and during CCh. Black squares with error bars represent group averages ± SEM. (B) Same as A, but intracellular dialysis is with 30 mM BAPTA and 100 nM free [Ca2+]. Summary data (B3) of the adaptation index for control and CCh (n=8). (C) Same as A, but intracellular dialysis is with 30 mM BAPTA and 10 nM free [Ca2+]. Summary data (C3) of the adaptation index for control and CCh (n=12). *** p<0.0005, ** p=0.001, n.s. not significant, paired t-test. Source data in "Figure 10—source data 1".
-
Figure 10—source data 1
A high concentration of BAPTA is required to interfere with CCh-mediated shift in firing along ramp.
- https://cdn.elifesciences.org/articles/84387/elife-84387-fig10-data1-v2.xlsx
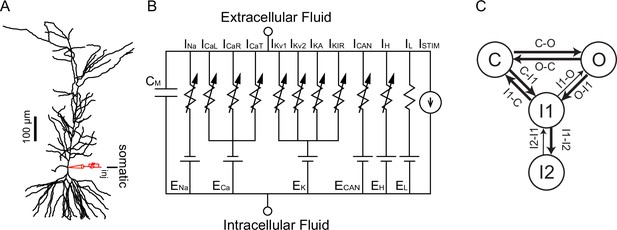
Model Schematics.
(A) Reconstructed morphology ported to NEURON simulation program, with somatic current injection site labeled. (B) Equivalent circuit diagram, with the reversal potentials for each channel indicated by EX. The following currents were modeled using Ohmic drive and conductances in parallel with a membrane capacitance (CM): a Na+ current (INa), L-type (ICaL), R-type (ICaR), and T-type (ICaT) Ca2+ currents, two delayed rectifiers (IKv1 and IKv2), an A-type K+ current (IA), an inward rectifying K+ current (IKIR), a nonspecific Ca2+-activated current (ICAN), a hyperpolarization-activated mixed cationic h-current (Ih), and a leak current (IL). (C) Markov Model of the NaV channel with an open state (O), a closed state (C), a short-term inactivated state (I1) and a long-term inactivated state (I2). See also Figure 11—figure supplement 1.
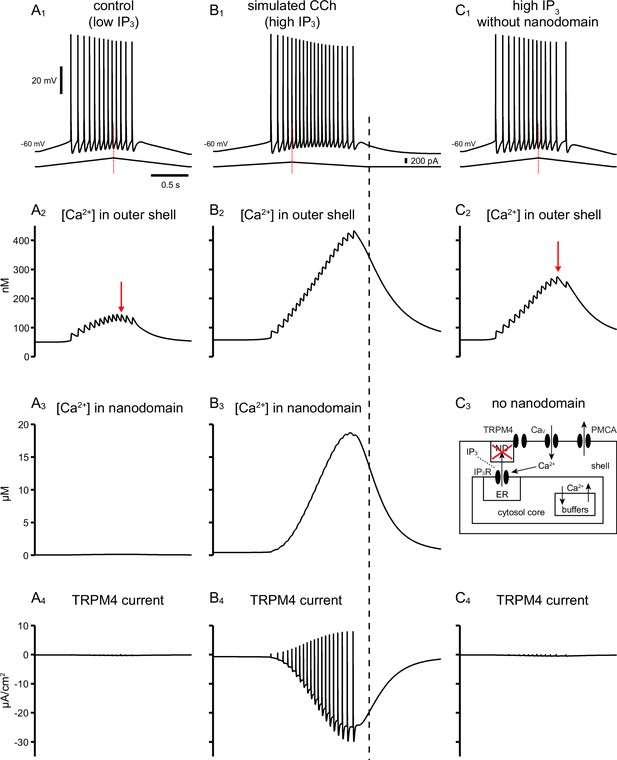
Old model in which the source of Ca2+ with privileged access to the nanodomain required to activated TRPM4 channels is IP3R.
This model captures the responses seen with cholinergic activation in the experiments. (A) Simulated somatic recordings in control (low IP3). (A1) Voltage traces and current ramp. (A2) Nanomolar Ca2+ concentration in outer shell beneath the cell membrane. (A3) The nanodomain Ca2+ concentration is not elevated with low levels of IP3 that simulate control. (A4) There is negligible TRPM4 activation. B. Simulated somatic recordings in CCh (high IP3). (B1) Voltage traces and current ramp. (B2) Nanomolar Ca2+ concentration in outer shell beneath the cell membrane is elevated due to increased spiking on the down-ramp. (B3) The nanodomain Ca2+ concentration reached micromolar concentrations with high levels of IP3 that simulate application of CCh. (B4) TRPM4 is robustly activated. Black dashed line marks the end of the injected current ramp, highlighting that the return to baseline of Ca2+ and TRPM4 current lags behind injected current ramp. (C) Simulated somatic recordings in CCh (high IP3) with no nanodomain. (C1) Voltage traces and current ramp. Note that more ramp current is required to achieve the same firing rates as were achieved in B1. (C2) Nanomolar Ca2+ concentration in outer shell beneath the cell membrane.( C3) No nanodomain. (C4) There is negligible TRPM4 activation. In A2 and C2, the red arrows point to when the [Ca2+] in the outer shell starts decreasing while the neuron keeps firing, as opposed to B2.
Tables
Reagent type (species) or resource | Designation | Source or reference | Identifiers | Additional information |
---|---|---|---|---|
Strain, strain background | Male Sprague Dawley rats | Envigo (Inovit) | Hsd:Sprague Dawley SD | 7–12 weeks old when sacrificed for experiments |
Chemical compound, drug | NBQX | Alomone Labs | cat# N-186 | AMPA receptor antagonist |
Chemical compound, drug | DL-APV | HelloBio | cat# HB0252 | NMDA receptor antagonist |
Chemical compound, drug | Gabazine | Alomone Labs | cat# G-216 | GABAA receptor antagonist |
Chemical compound, drug | CGP55845 | Abcam | cat# ab120337 | GABAB receptor antagonist |
Chemical compound, drug | Carbachol | Tocris | cat# 2810 | Acetylcholine receptor agonist |
Chemical compound, drug | Acetylcholine | Sigma-Aldrich | cat# A6625 | Ligand for acetylcholine receptors |
Chemical compound, drug | flufenamic acid | Sigma-Aldrich | cat# F9005 | TRP channel antagonist |
Chemical compound, drug | SKF96365 | Alomone Labs | cat# S-175 | TRPC channel antagonist |
Chemical compound, drug | CBA | Tocris | cat# 6724 | TRPM4 channel antagonist |
Chemical compound, drug | 9-phenanthrol | Tocris | cat# 4999 | TRPM4 channel antagonist |
Chemical compound, drug | Xestospongin C | Abcam | cat# ab120914 | IP3R antagonist |
Chemical compound, drug | low molecular weight heparin | Sigma-Aldrich | cat# H3149 | IP3R antagonist |
Chemical compound, drug | D-myo-IP3 | Cayman Chemical | cat# 60960 | IP3R agonist |
Chemical compound, drug | Adenophostin A | MilliporeSigma | cat# 115500 | IP3R agonist |
Chemical compound, drug | Ryanodine | HelloBio | cat# HB1320 | RyR antagonist at [µM] |
Chemical compound, drug | Ruthenium red | Tocris | cat# 1439 | RyR antagonist |
Chemical compound, drug | Dantrolene | Tocris | cat# 0507 | RyR antagonist |
Chemical compound, drug | caffeine | Tocris | cat# 2793 | RyR agonist |
Chemical compound, drug | Thapsigargin | Alomone Labs | cat# T-650 | SERCA inhibitor |
Chemical compound, drug | BAPTA tetra-potassium salt (K4-BAPTA) | Sigma-Aldrich | cat# A9801 | fast Ca2+ chelator |
Software, algorithm | MAXCHELATOR, WEBMAXC EXTENDED | https://somapp.ucdmc.ucdavis.edu/pharmacology/bers/maxchelator/webmaxc/webmaxcE.htm | Online free metal calculator | |
Software, algorithm | SPSS | IBM | RRID:SCR_002865 | Statistics software |
Software, algorithm | NEURON | Hines and Carnevale, 1997; Carnevale and Hines, 2006 | doi:doi.org/10.1162/neco.1997.9.6.1179; doi.org/10.1017/CBO9780511541612 | Computational modelling and simulation software |
Other | Laerd Statistics | https://statistics.laerd.com/ | Online tutorial and software guide |