Bacterial lifestyle switch in response to algal metabolites
Figures
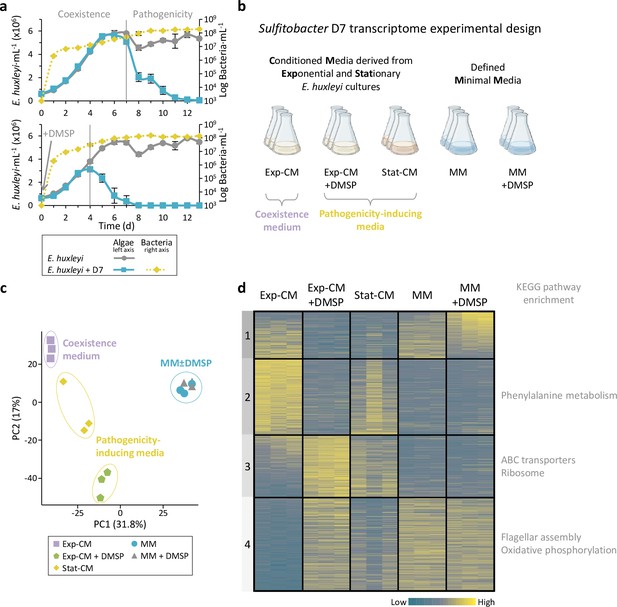
Transcriptional profiling of Sulfitobacter D7 in response to dimethylsulfoniopropionate (DMSP) and additional Emiliania huxleyi infochemicals reveals the signaling role of DMSP.
(a) Time course of E. huxleyi CCMP379 and bacterial abundance (full and dashed lines, left and right axes, respectively) in algal mono-cultures or during co-culturing with Sulfitobacter D7. Top panel: Co-cultures display two phases with distinct bacterial lifestyles: coexistence and pathogenicity. Bottom panel: DMSP was added at day 0 to a final concentration of 100 µM. Results represent average ± SD (n=3). Figure 1a has been adapted from Figure 5 of Barak-Gavish et al., 2018. (b) Design of Sulfitobacter D7 transcriptome experiment aiming to explore gene expression profiles in response to E. huxleyi-derived media and in response to DMSP. Growth media consisted of conditioned media (CM) derived from E. huxleyi at exponential growth or stationary phase (Exp-CM and Stat-CM, respectively), and an additional treatment in which 100 µM of DMSP was added (Exp-CM + DMSP). These media differentially induce the coexistence and pathogenicity lifestyles of Sulfitobacter D7. In order to identify DMSP-responsive genes we inoculated Sulfitobacter D7 in defined minimal media (MM), lacking E. huxleyi-derived exudates, without and with 100 µM DMSP (MM and MM + DMSP, respectively). Sulfitobacter D7 was inoculated into each media and harvested for RNA profiling after 24 hr of growth. Initial conditions of the media and bacterial growth are elaborated in Table 1. (c) Principle component analysis of Sulfitobacter D7 detected genes in all treatments (2588 genes). Triplicates of each treatment are shown. (d) Heatmap of gene expression of all differentially expressed genes in the comparisons Exp-CM + DMSP vs. Exp-CM, Stat-CM vs. Exp-CM, and MM + DMSP vs. MM (1179 genes). Clusters were determined based on k-means analysis. Significant functional enrichment in each cluster, based on Kyoto encyclopedia of genes and genomes (KEGG) pathways, is denoted. Each row represents one gene, and the color intensity corresponds to the standardized expression across all samples (triplicates of each treatment are shown). Expression values are scaled by row. Genes in cluster 1 are ordered based on the mean expression values in the MM + DMSP treatment. Genes in cluster 2–4 are ordered based on the mean expression values in the Exp-CM treatment.
-
Figure 1—source data 1
Time course of E. huxleyi CCMP379 and Sulfitobacter D7 abundances.
- https://cdn.elifesciences.org/articles/84400/elife-84400-fig1-data1-v1.xlsx
-
Figure 1—source data 2
RNA sequencing data of Sulfitobacter D7 transcriptome.
- https://cdn.elifesciences.org/articles/84400/elife-84400-fig1-data2-v1.xlsx
-
Figure 1—source data 3
Sulfitobacter D7 expression of genes belonging to KEGG pathways that are enriched in clusters 2–4 of the heatmap in Figure 1d.
- https://cdn.elifesciences.org/articles/84400/elife-84400-fig1-data3-v1.xlsx
-
Figure 1—source data 4
Number of sequencing reads of Sulfitobacter D7 transcriptome experiment.
- https://cdn.elifesciences.org/articles/84400/elife-84400-fig1-data4-v1.xlsx
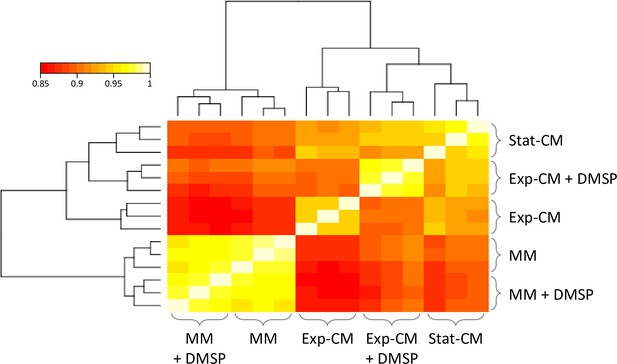
Gene expression similarity between the treatments of Sulfitobacter D7 transcriptome.
Pearson correlation and dendrogram of hierarchical clustering between all samples in Sulfitobacter D7 transcriptome according to gene expression values. Replicates of same treatment show high correlation and cluster together. Conditioned media (CM) and minimal media (MM) treatments cluster separately. DMSP, dimethylsulfoniopropionate; Exp-CM, exponential CM; Stat-CM, stationary CM.
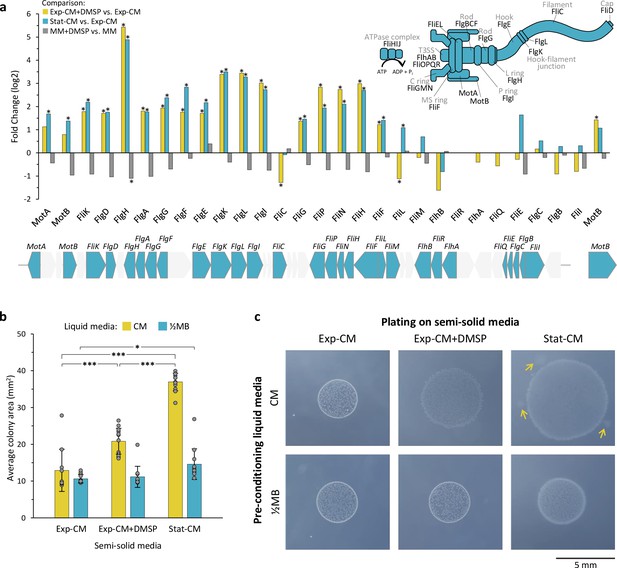
Induction of flagellar genes and increased motility in pathogenicity-inducing media.
(a) Fold change of flagellar gene expression in the comparisons: Exp-CM +DMSP vs. Exp-CM (yellow), Stat-CM vs. Exp-CM (blue), and MM +DMSP vs. MM (gray). Genes marked with * are significantly differentially expressed. The flagellar genes are localized in a gene cluster on the Sulfitobacter D7 chromosome, as shown below the graph. Genes in gray are not related to the flagellum. The function of each gene is indicated in the flagellum assembly scheme on the top-right. Expression values are presented in Figure 2—source data 1. (b) Bacterial motility inferred by the colony area of Sulfitobacter D7 plated on semi-solid agar media. Bacteria were pre-conditioned in liquid CM (Exp-CM, Exp-CM + DMSP, and Stat-CM) for 24 hr and plated on the corresponding semi-solid CM plates (yellow bars). For control, bacteria were pre-conditioned on liquid marine broth (½MB) and plated on semi-solid CM plates (blue bars). Colony area was determined after 6 days of growth. Results represent average ± SD (n=10–12 colonies per treatment). Statistical differences were tested using two-way analysis of variance, followed by Tukey’s post-hoc test. * p-value <0.05, *** p-value <0.0001. (c) Representative bacterial colonies from each treatment showing the difference in colony area and morphology. The arrows depict bacterial motility extensions from the core colony. The extensions were not included in the colony area measurements. DMSP, dimethylsulfoniopropionate; CM, conditioned media; MM, minimal media; Exp-CM, exponential CM; Stat-CM, stationary CM.
-
Figure 2—source data 1
Sulfitobacter D7 expression of flagellar genes.
- https://cdn.elifesciences.org/articles/84400/elife-84400-fig2-data1-v1.xlsx
-
Figure 2—source data 2
Bacterial motility inferred by the colony area of Sulfitobacter D7 plated on semi-solid agar media.
- https://cdn.elifesciences.org/articles/84400/elife-84400-fig2-data2-v1.xlsx
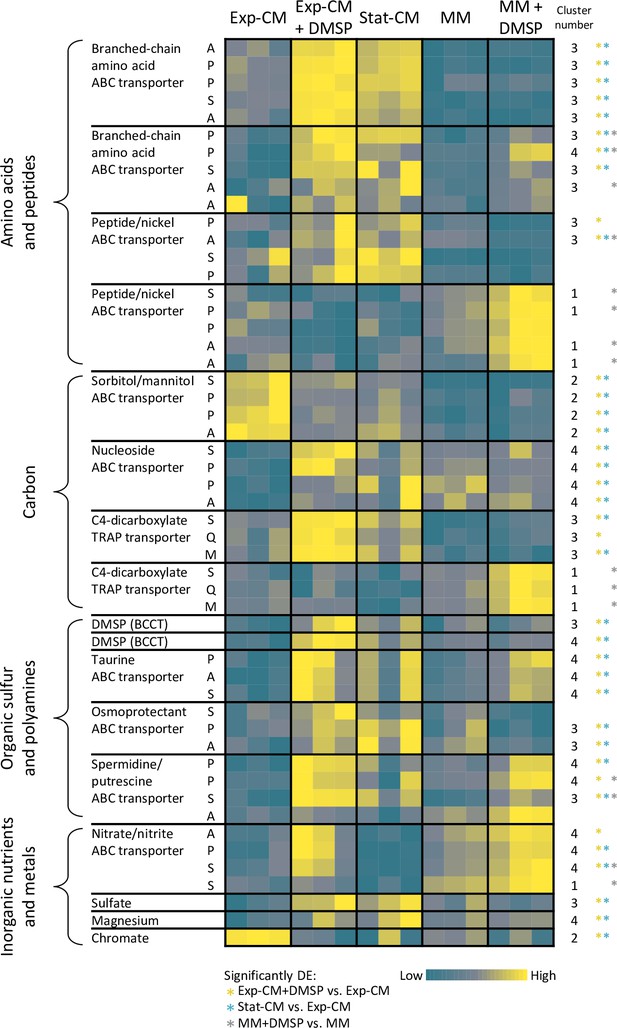
Remodeling of Sulfitobacter D7 transport systems in response to dimethylsulfoniopropionate (DMSP) and Emiliania huxleyi-derived metabolites.
Heatmap of gene expression of representative transport genes for various metabolite classes. Each row represents one gene, and the blocks represent a complete transport system in which at least two genes were differentially expressed (DE) in the comparisons indicated on the bottom. The column ‘cluster number’ corresponds to the heatmap cluster in Figure 1d in which the gene is found. Colored * denotes in which comparison of the gene was significantly DE. Color intensity corresponds to the standardized expression across all samples (triplicates of each treatment are shown). Expression values are scaled by row. Expression and fold-change values are presented in Figure 3—source data 1. ABC, ATP-binding cassette; TRAP, tripartite ATP-independent periplasmic; BCCT, betaine/carnitine/choline transporter. The letters correspond to the transport system components: A, ATP-binding; S, substrate-binding; P, permease; Q, dctQ subunit; M, dctM subunit.
-
Figure 3—source data 1
Sulfitobacter D7 expression of DE transport genes.
- https://cdn.elifesciences.org/articles/84400/elife-84400-fig3-data1-v1.xlsx
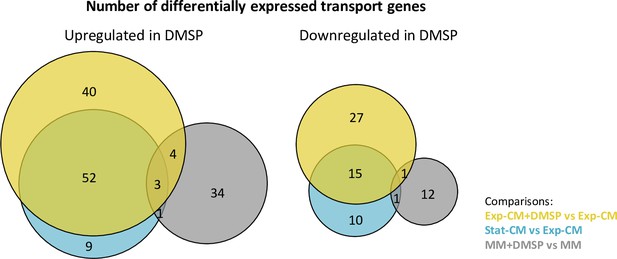
Differential expression of Sulfitobacter D7 transport genes in coexistence and pathogenic states and in response to dimethylsulfoniopropionate (DMSP).
Venn diagrams showing the number of differentially expressed transport genes in the indicated treatment comparisons. The left diagram corresponds to genes that were upregulated in samples with higher DMSP concentration, and the right side is downregulated genes. Venn diagrams were generated using BioVenn (Hulsen et al., 2008). CM, conditioned media; MM, minimal media; Exp-CM, exponential CM; Stat-CM, stationary CM.
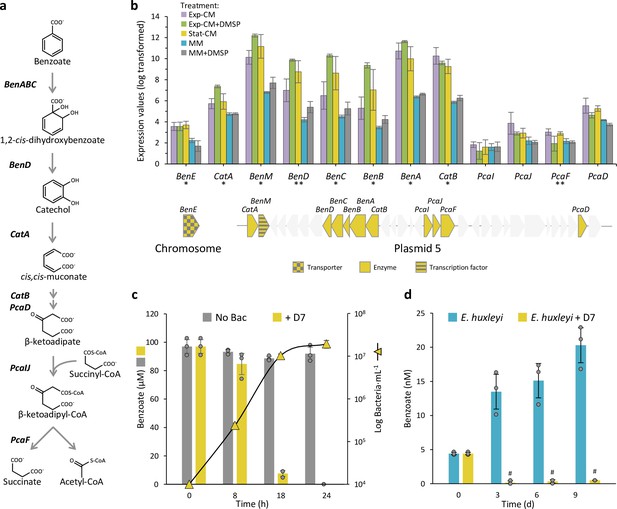
Sulfitobacter D7 encodes for a benzoate degradation pathway and uptakes Emiliania huxleyi-produced benzoate during interaction.
(a) The benzoate degradation pathway in Sulfitobacter D7. The genes that encode for the enzymes mediating the subsequent transformations of benzoate to succinate and acetyl-CoA are denoted in bold. (b) Expression values of benzoate-related genes, which are localized on Sulfitobacter D7 plasmid 5 in a gene cluster, as indicated below the graph. The benzoate transporter, BenE, is encoded on the chromosome. Genes in gray are not related to benzoate. Results represent average ± SD (n=3). Expression and fold-change values are presented in Figure 4—source data 1. Genes marked with * were significantly differentially expressed (DE) between all conditioned media (CM) and minimal media (MM) treatments (Exp-CM vs MM, Exp-CM vs MM + DMSP, Exp-CM +DMSP vs MM, Exp-CM +DMSP vs MM +DMSP, Stat-CM vs MM, and Stat-CM vs MM +DMSP). Genes marked with ** were significantly DE between all CM and MM treatments but one comparison (for BenD Exp-CM vs MM +DMSP; for PcaF Exp-CM +DMSP vs MM). (c) Benzoate concentration (bars, left axis) and bacterial growth (triangles, right axis) in MM supplemented with 100 µM benzoate, as a sole carbon source, without inoculation (gray) and upon inoculation of Sulfitobacter D7 (yellow). Results represent average ± SD (n=3). p-Value <0.0001 for the difference in benzoate concentration between the ‘No bac’ and ‘+D7’ treatments. No bacterial growth was observed in un-inoculated MM. (d) Extracellular benzoate concentration in the media of E. huxleyi CCMP379 cultures during growth in mono-culture (blue) or during co-culturing with Sulfitobacter D7 (yellow). # Benzoate was detected below the limit of quantification. Results represent average ± SD (n=3). p-Value <0.0001 for the difference in benzoate concentration between the ‘E. huxleyi’ and ‘E. huxleyi +D7’ treatments. Statistical differences in (c) and (d) were tested using a mixed effects model, with treatment and time as fixed effects, and replicate as a random effect. BenABC, benzoate 1,2-dioxygenase subunit alpha, beta, and reductase component, respectively; BenD, cis-1,2-dihydroxybenzoate dehydrogenase; CatA, catechol 1,2-dioxygenase; CatB, muconate cycloisomerase; PcaD, 3-oxoadipate enol-lactonase; PcaIJ, 3-oxoadipate CoA-transferase, alpha and beta subunits, respectively; PcaF, 3-oxoadipyl-CoA thiolase, DMSP, dimethylsulfoniopropionate; CM, conditioned media; MM, minimal media; Exp-CM, exponential CM; Stat-CM, stationary CM.
-
Figure 4—source data 1
Sulfitobacter D7 expression of benzoate transport and metabolism genes.
- https://cdn.elifesciences.org/articles/84400/elife-84400-fig4-data1-v1.xlsx
-
Figure 4—source data 2
Benzoate concentration and bacterial growth in mono-cultures.
- https://cdn.elifesciences.org/articles/84400/elife-84400-fig4-data2-v1.xlsx
-
Figure 4—source data 3
Extracellular benzoate concentration in the media of Emiliania huxleyi CCMP379 cultures.
- https://cdn.elifesciences.org/articles/84400/elife-84400-fig4-data3-v1.xlsx
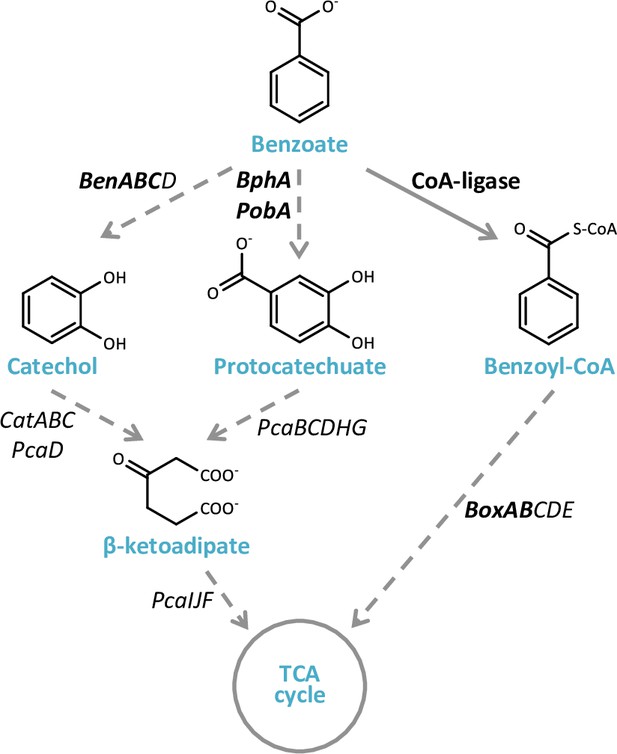
Bacterial benzoate degradation pathways.
Aerobic degradation of benzoate by bacteria can occur through three possible pathways: through catechol (Ben genes), through protocatechuate (BphA and PobA genes), and through benzoyl-CoA (Box genes). The first two pathways converge to β-ketoadipate, and in all the three pathways the end products are acetyl-CoA and succinate/succinyl-CoA, which are channeled to the tricarboxylic acid (TCA) cycle (Fuchs et al., 2011). The names of the genes encoding for the enzymes mediating the metabolic transformations are denoted next to the arrows. Full arrow represents a single enzymatic reaction, while dashed arrows represent multiple enzymatic reactions. The genes marked in bold were used as query genes for examining the prevalence of benzoate transport and catabolism genes in genomes of phytoplankton-associated bacteria (Figure 5a, Figure 5—source data 1). BenABC, benzoate 1,2-dioxygenase subunit alpha, beta, and reductase component, respectively; BenD, cis-1,2-dihydroxybenzoate dehydrogenase; CatA, catechol 1,2-dioxygenase; CatB, muconate cycloisomerase; PcaD, 3-oxoadipate enol-lactonase; PcaIJ, 3-oxoadipate CoA-transferase, alpha and beta subunits, respectively.
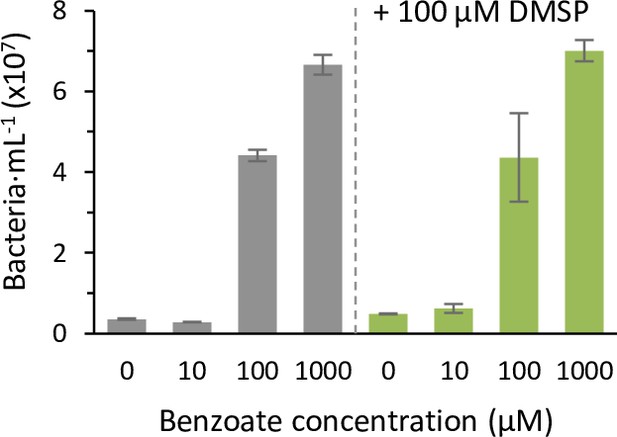
Benzoate promotes Sulfitobacter D7 growth.
Bacterial growth after 24 hr in minimal media supplemented with different concentrations of benzoate as sole carbon source, without or with addition of 100 µM dimethylsulfoniopropionate (DMSP; gray and green, respectively). Results represent average ± SD (n=3). Statistical differences were tested using two-way analysis of variance, followed by Tukey's post-hoc test. p-Value <0.01 for the difference between ‘100 µM’ and ‘1000 µM’ compared to ‘0 µM’. Addition of DMSP did not significantly affect bacterial growth.
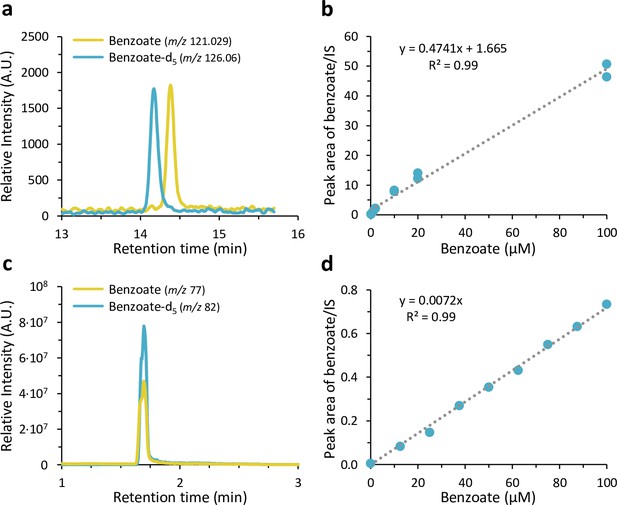
Detection and quantification of benzoate by two Liquid chromatography–mass spectrometry (LC-MS) methods.
(a-b) Ultra-high-performance liquid chromatography-electrospray ionization (UHPLC-ESI)-high resolution mass spectrometry analysis of benzoate. (a) Extracted ion chromatograms (EICs) of the molecular ions [M-H]− of benzoate (m/z 121.029; yellow) and benzoate-d5 (m/z 126.06; blue) as internal standard (IS) from a solid phase extract of artificial seawater that was spiked with both metabolites at 1 µM. The IS benzoate-d5 elutes 0.2 min earlier due to its uniformly deuterium-labeled aromatic ring. (b) Standard calibration curve of sodium benzoate and benzoate-d5 as IS from solid phase extracts of artificial seawater samples that were spiked in duplicates at different benzoate concentrations ranging from 0.2 µM to 100 µM. (c-d) UHPLC-ESI-tandem mass spectrometry (MS/MS) analysis of benzoate. (c) EICs of the fragment ions [M-H-CO2]− of benzoate (m/z 77) and benzoate-d5 (m/z 82) as IS in a calibration curve sample with 100 µM benzoate and 125 µM benzoate-d5. (d) Standard calibration curve of sodium benzoate and benzoate-d5 as IS at different benzoate concentrations ranging from 12.5 nM to 100 nM.
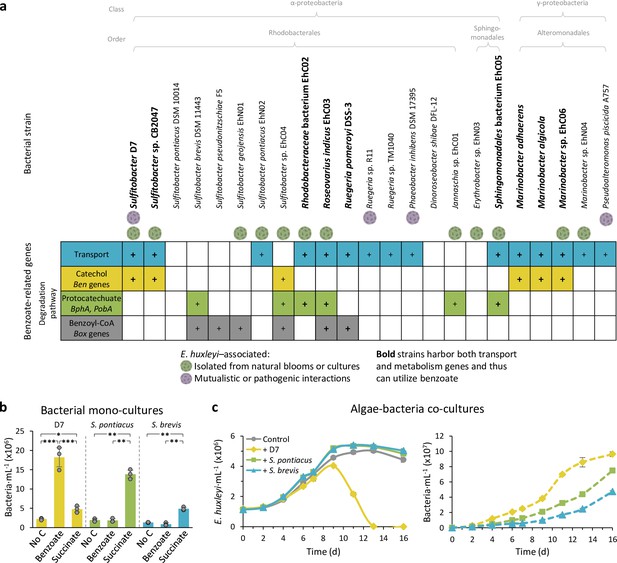
Benzoate utilization may impact the bacterial lifestyle switch.
(a) Presence of benzoate transport and degradation genes in genomes of phytoplankton-associated Proteobacteria. Emiliania huxleyi-associated bacteria are denoted. Colored tiles depict the presence of the genes. Bacterial strains highlighted in bold possess genes for both transport and degradation of benzoate. Bacterial benzoate degradation pathways are elaborated in Figure 4—figure supplement 1. The full data of the presence of all query genes in the genomes of the bacteria is presented in Figure 5—source data 1. The query genes are listed in Key resources table. (b) Bacterial growth after 24 hr in minimal media with no carbon source or supplemented with 100 µM benzoate or succinate, as a sole carbon source, of Sulfitobacter D7 (yellow) and two additional Sulfitobacter strains (Sulfitobacter pontiacus DSM 10014 [green] and Sulfitobacter brevis DSM 11443 [blue]) that do not possess the genes required for benzoate utilization. Results represent average ± SD (n=3). Statistical differences were tested using two-way analysis of variance, followed by Tukey’s post-hoc test. * p-Value <0.05, ** p-value <0.01 *** p-value <0.0001. (c) Time course of E. huxleyi CCMP379 and bacterial abundance (left and right panels, respectively) in algal mono-cultures (gray) or during co-culturing with Sulfitobacter D7 (yellow), S. pontiacus (green), or S. brevis (blue). Results represent average ± SD (n=3). Statistical differences were tested using repeated-measures analysis of variance, followed by a Tukey post hoc test. p-Value <0.0001 for the difference in E. huxleyi and bacterial growth between all treatments, except for E. huxleyi growth in the treatments ‘+S. pontiacus’ compared to ‘+S. brevis’, which was not significant.
-
Figure 5—source data 1
Presence of benzoate degradation and transport genes in genomes of phytoplankton-associated proteobacteria.
- https://cdn.elifesciences.org/articles/84400/elife-84400-fig5-data1-v1.xlsx
-
Figure 5—source data 2
Bacterial growth in MM with different carbon sources.
- https://cdn.elifesciences.org/articles/84400/elife-84400-fig5-data2-v1.xlsx
-
Figure 5—source data 3
Emiliania huxleyi CCMP379 and bacterial abundances.
- https://cdn.elifesciences.org/articles/84400/elife-84400-fig5-data3-v1.xlsx
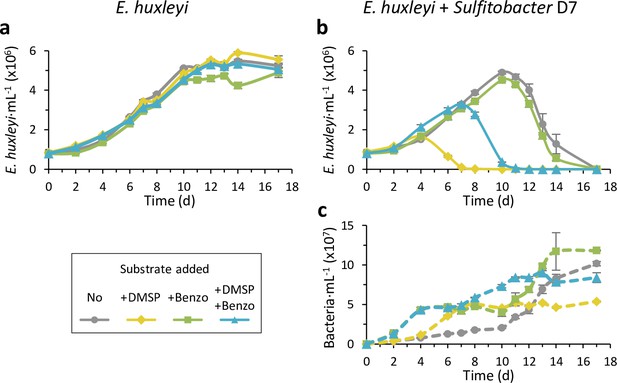
Benzoate hinders the dimethylsulfoniopropionate (DMSP)-induced pathogenicity of Sulfitobacter D7 against Emiliania huxleyi.
Time course of E. huxleyi CCMP379 and bacterial abundance (smooth and dashed lines, respectively) in algal mono-cultures (a) or during co-culturing with Sulfitobacter D7 (b-c). No bacterial growth was observed in algal mono-cultures. Cultures were supplemented at day 0 with 100 µM of DMSP (yellow), benzoate (benzo, green), DMSP and benzoate (blue), or none (gray). The presence of benzoate hindered the pathogenicity-inducing effect of DMSP. Results represent average ± SD (n=3). Statistical differences were tested using repeated-measures analysis of variance, followed by a Tukey post hoc test. p-Value <0.0001 for the difference in E. huxleyi growth in the treatment of ‘+D7+DMSP’ and ‘D7 +DMSP + Benzoate’ compared to all other treatments. p-Value <0.0001 for the differences in bacterial growth in the treatments ‘+D7+benzoate’ and ‘+D7+Benzoate + DMSP’ compared to only ‘+D7’.
-
Figure 6—source data 1
Time course of Emiliania huxleyi CCMP379 abundance in mono-cultures supplemented with different substrates.
- https://cdn.elifesciences.org/articles/84400/elife-84400-fig6-data1-v1.xlsx
-
Figure 6—source data 2
Time course of Emiliania huxleyi CCMP379 and Sulfitobacter D7 abundance in co-cultures supplemented with different substrates.
- https://cdn.elifesciences.org/articles/84400/elife-84400-fig6-data2-v1.xlsx
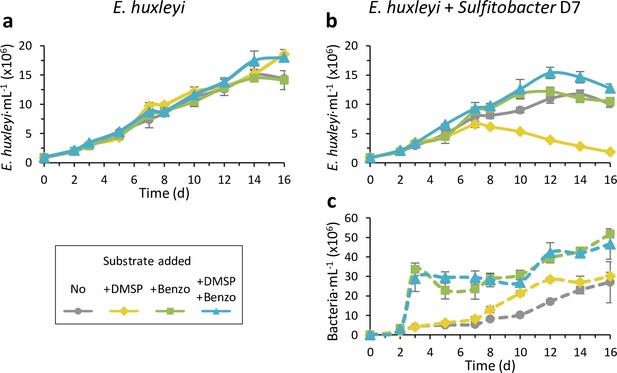
Benzoate is a key metabolite for maintaining Emiliania huxleyi-Sulfitobacter D7 coexistence.
Time course of E. huxleyi CCMP2090 and bacterial abundance (smooth and dashed lines, respectively) in algal mono-cultures (a) or during co-culturing with Sulfitobacter D7 (b-c). No bacterial growth was observed in algal mono-cultures. Cultures were supplemented at day 0 with 100 µM of dimethylsulfoniopropionate (DMSP; yellow), benzoate (benzo, green), DMSP and benzoate (blue), or none (gray). The presence of benzoate negated the pathogenicity-inducing effect of DMSP. Results represent average ± SD (n=3). p-value <0.0001 for the difference in E. huxleyi growth in the treatment of ‘+D7+DMSP’ compared to all other treatments. p-Value <0.05 for the differences in bacterial growth in the treatments ‘+D7+benzoate’ and ‘+D7+Benzoate + DMSP’ compared to only ‘+D7’.
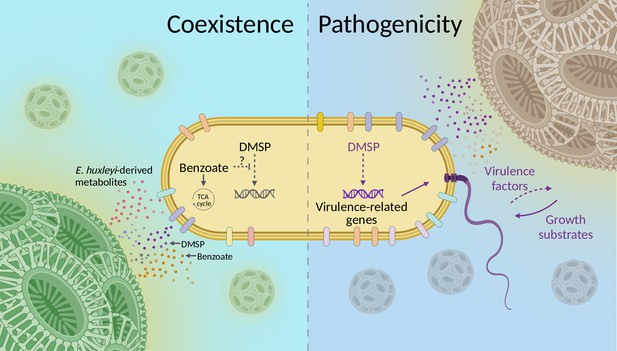
Conceptual model of the lifestyle switch of Sulfitobacter D7 in response to Emiliania huxleyi-derived metabolites.
During its interactions with E. huxleyi, Sulfitobacter D7 exhibits a lifestyle switch from coexistence to pathogenicity. In the coexistence phase, E. huxleyi secretes to the phycosphere various metabolites such as benzoate, dimethylsulfoniopropionate (DMSP), and other growth substrates, which bacteria can uptake and consume for growth. Based on the observation that benzoate hindered the pathogenicity-inducing effect of DMSP, we hypothesize that such energy-rich metabolic currencies hinder DMSP signaling in Sulfitobacter D7. When the algal physiological state is compromised, for example, stationary phase, the amount of available growth substrates decreases, due to bacterial consumption and less secretion by the alga. In this context, high concentration of algal DMSP acts as a signal that alters the transcriptional profiles of the bacterium and leads to high expression of pathogenicity-related genes, such as flagellar and transport genes, and yet unknown virulence factors that kill E. huxleyi cells. This leads to a surge of alga-derived growth substrates that are taken up efficiently by Sulfitobacter D7. The flagellum can mediate the dispersal of Sulfitobacter D7 and to forage for an alternative host.
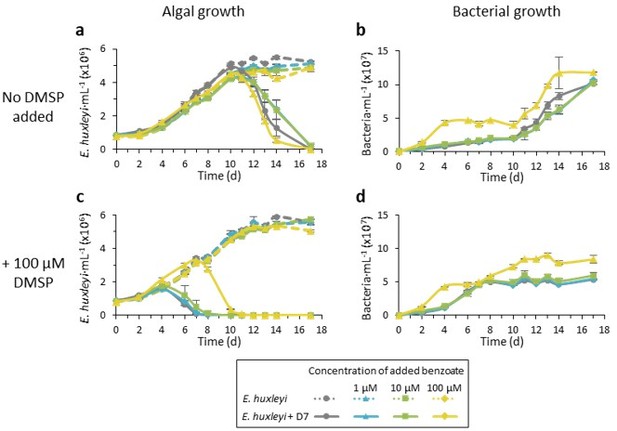
Time course of E. huxleyi CCMP379 mono-cultures (dashed lines) and during co-culturing with Sulfitobacter D7 (smooth lines).
Cultures we either not supplemented (a-b) or supplemented with 100 µM DMSP at day 0 (c-d). Benzoate was added to the cultures at day 0 in the following concentrations: 1 µM (blue), 10 µM (green), 100 µM (yellow) or none (grey).
Tables
Conditions of the media used for Sulfitobacter D7 transcriptome.
For conditioned media (CM)* | |||||
---|---|---|---|---|---|
Media | Sulfitobacter D7 abundance at t=24 hr (106∙mL–1)† | DMSP concentration (µM)‡ | Days of E. huxleyi growth | E. huxleyi abundance (cells∙mL–1) | % Dead cells‡ |
Exp-CM | 2.6±0.1 | 3 | 5 | 1∙106 | 8.5% |
Exp-CM + DMSP | 3.7±0.3 | 100 | 5 | 1∙106 | 8.5% |
Stat-CM | 17.5±0.8 | 10.5 | 12 | 3∙106 | 18% |
MM | 7.5±0.5 | 0 | – | – | – |
MM + DMSP | 18.6±1.3 | 100 | – | – | – |
-
DMSP, dimethylsulfoniopropionate; CM, conditioned media; MM, minimal media; Exp-CM, exponential CM; Stat-CM, stationary CM.
-
*
Conditions of exponential and stationary E. huxleyi cultures from which Exp-CM and Stat-CM were obtained, respectively (n=1).
-
†
Results represent average ± SD (n=3). At t=24 hr bacteria were harvested for transcriptome analysis (experimental setup, Figure 1b).
-
‡
DMSP and cell death measurements are described in Barak-Gavish et al., 2018 (n=1).
Number of Sulfitobacter D7 differentially expressed genes in pathogenicity vs. coexistence modes and in response to DMSP.
Exp-CM +DMSPvs. Exp-CM | Stat-CMvs. Exp-CM | MM +DMSPvs. MM | |
---|---|---|---|
Upregulated | 560 | 358 | 107 |
Downregulated | 408 | 237 | 63 |
Total | 968 | 495 | 170 |
-
DE genes were defined as genes with |fold change|>2 and adjusted P-value ≤0.05.
-
DMSP, dimethylsulfoniopropionate; CM, conditioned media; MM, minimal media; Exp-CM, exponential CM; Stat-CM, stationary CM.
Reagent type (species) or resource | Designation | Source or reference | Identifiers | Additional information |
---|---|---|---|---|
Gene (Acinetobacter sp. strain ADP1) | BenK1, Benzoate transporter | Collier et al., 1997 | AAC46425.1 | |
Gene (Pseudomonas putida KT2440) | BenK2, Benzoate transporter | Nishikawa et al., 2008 | AAN68773.1 | |
Gene (Pseudomonas putida KT2440) | BenE1, Benzoate transporter | Nishikawa et al., 2008 | AAN67649.1 | |
Gene (Pseudomonas putida KT2440) | BenE2, Benzoate transporter | Nishikawa et al., 2008 | AAN68775.1 | |
Gene (Pseudomonas putida KT2440) | BenF, Benzoate transporter | Nishikawa et al., 2008 | AAN68776.1 | |
Gene (Acinetobacter sp. strain ADP1) | BenA, Benzoate 1,2-dioxygenase subunit alpha | Collier et al., 1998 | AAC46436.2 | Benzoate degradation pathway: Catechol |
Gene (Acinetobacter sp. strain ADP1) | BenB, Benzoate 1,2-dioxygenase subunit beta | Collier et al., 1998 | AAC46437.1 | Benzoate degradation pathway: Catechol |
Gene (Acinetobacter sp. strain ADP1) | BenC, Benzoate 1,2-dioxygenase electron transfer component | Collier et al., 1998 | AAC46438.1 | Benzoate degradation pathway: Catechol |
Gene (Aspergillus niger) | BphA, Benzoate 4-monooxygenase | van Gorcom et al., 1990 | P17549.1 | Benzoate degradation pathway: Protocatechuate |
Gene (Acinetobacter sp. strain ADP1) | PobA, 4-hydroxybenzoate 3-monooxygenase | Brzostowicz et al., 2003 | WP_004926674.1 | Benzoate degradation pathway: Protocatechuate |
Gene (Azoarcus evansii) | Benzoate CoA-ligase | Mohamed et al., 2001; Gescher et al., 2002 | AAN39371.1 | Benzoate degradation pathway: Benzoyl-CoA |
Gene (Azoarcus evansii) | BoxA, Benzoyl-CoA oxygenase component A | Gescher et al., 2002 | AAN39377.1 | Benzoate degradation pathway: Benzoyl-CoA |
Gene (Azoarcus evansii) | BoxB, Benzoyl-CoA oxygenase component B | Gescher et al., 2002 | AAN39376.1 | Benzoate degradation pathway: Benzoyl-CoA |
Strain, strain background (Emiliania huxleyi) | CCMP379 | National Center for Marine Algae (NCMA) | ||
Strain, strain background (Emiliania huxleyi) | CCMP2090 | National Center for Marine Algae (NCMA) | ||
Strain, strain background (Sulfitobacter D7) | Barak-Gavish et al., 2018 | |||
Strain, strain background (Sulfitobacter pontiacus) | DSM 10014 | The Leibniz Institute DSMZ | ||
Strain, strain background (Sulfitobacter brevis) | DSM 11443 | The Leibniz Institute DSMZ | ||
Chemical compound, drug | (2-Carboxyethyl) dimethylsulfonium chloride 97% (DMSP) | Holland Moran | HC003BMH | |
Chemical compound, drug | Sodium benzoate | Sigma-Aldrich | B3420 | |
Software, algorithm | R | R Development Core Team, 2021 | ||
Software, algorithm | Partek Genomics Suite software, v7.0 | Partek Inc, 2020 | ||
Software, algorithm | Heatmapper | Babicki et al., 2016 | ||
Software, algorithm | g:Profiler | Raudvere et al., 2019 |