The Plasmodium falciparum apicoplast cysteine desulfurase provides sulfur for both iron-sulfur cluster assembly and tRNA modification
Figures
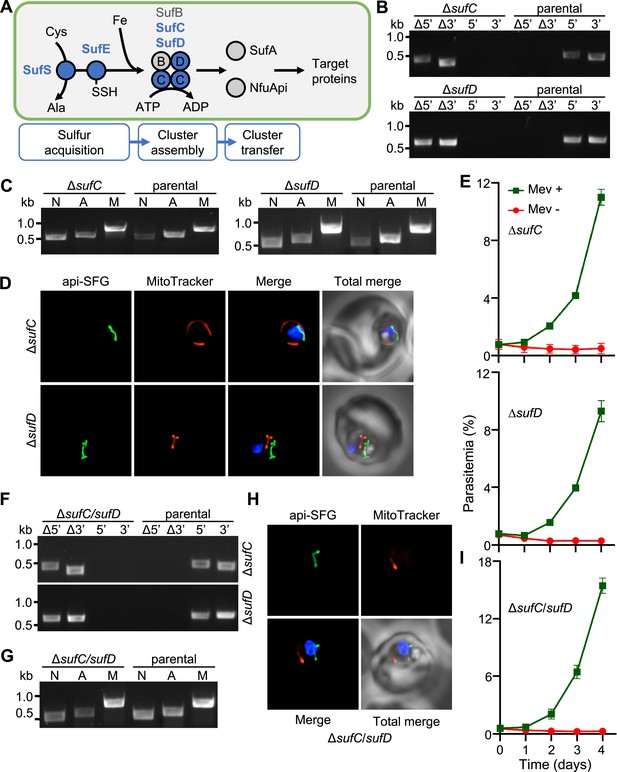
Apicoplast FeS assembly is not essential for apicoplast maintenance.
(A) FeS assembly in the P. falciparum apicoplast. In the sulfur acquisition step, SufS liberates sulfur from cysteine (Cys), resulting in a bound persulfide. SufE transfers sulfur from SufS to SufB of the SufBC2D FeS assembly complex. SufA and NfuApi receive FeS cofactors from the SufBC2D complex and transfer them to downstream FeS-dependent proteins. The proteins investigated in this study are colored blue. Ala, alanine; Fe, iron. (B) Genotyping PCR confirming deletion of sufC (top panel) and ΔsufD (bottom panel). (C) Successful amplification of sufB (lane A) gene in the ΔsufC (left panel) and ΔsufD (right panel) parasites indicates the presence of the apicoplast genome. (D) Representative epifluorescence microscopy images of ΔsufC (top panel) and ΔsufD (bottom panel) parasites show a single intact apicoplast. (E) Mevalonate (Mev)-dependent growth of ΔsufC (top panel) and ΔsufD (bottom panel) parasites over 4 days. (F) Genotyping PCR confirms deletion of both sufC (top panel) and sufD (bottom panel) in ΔsufC/sufD double knockout parasites. (G) Successful amplification of sufB (lane A) demonstrates intact apicoplast in ΔsufC/sufD parasites. (H) Representative epifluorescence microscopy images of ΔsufC/sufD parasites shows an apicoplast with typical intact morphology. (I) Mevalonate (Mev)-dependent growth of ΔsufC/sufD parasites over 4 days. In panels (B) and (F), successful gene deletion is demonstrated by the presence of PCR products for the Δ5’ and Δ3’ loci, but not the unmodified loci (5’ and 3’) found in the PfMev (parental) line. Expected PCR amplicon sizes are in Figure 1—figure supplement 1C. In (C) and (G), PCR detection of the ldh, sufB, and cox1 genes of the parasite nuclear (N), apicoplast (A), and mitochondrial (M) genomes, respectively, were attempted from transgenic as well as PfMev (parental) parasite lines. In (D) and (H), the api-SFG protein (green) labels apicoplast, MitoTracker (red) stains the mitochondrion, and nuclear DNA is stained with DAPI (blue). Each image shows a field representing 10 µm × 10 µm. For panels (E) and (I), asynchronous parasites were cultured with or without 50 μM Mev and parasitemia was monitored every 24 hr via flow cytometry. Data points show daily mean parasitemia ± standard error of the mean (SEM) from 2 independent biological replicates, each with four technical replicates. DNA markers in (B), (C), (F), and (G) are in kilobases (kb).
-
Figure 1—source data 1
Uncropped agarose gel images of PCR analyses presented in Figure 1B, C, F, and G.
- https://cdn.elifesciences.org/articles/84491/elife-84491-fig1-data1-v2.zip
-
Figure 1—source data 2
Growth assay parasitemia counts for ∆sufC (top table) and ∆sufD (bottom table) used for Figure 1E.
- https://cdn.elifesciences.org/articles/84491/elife-84491-fig1-data2-v2.xlsx
-
Figure 1—source data 3
∆sufC/sufD growth assay parasitemia counts used for Figure 1(I).
- https://cdn.elifesciences.org/articles/84491/elife-84491-fig1-data3-v2.xlsx
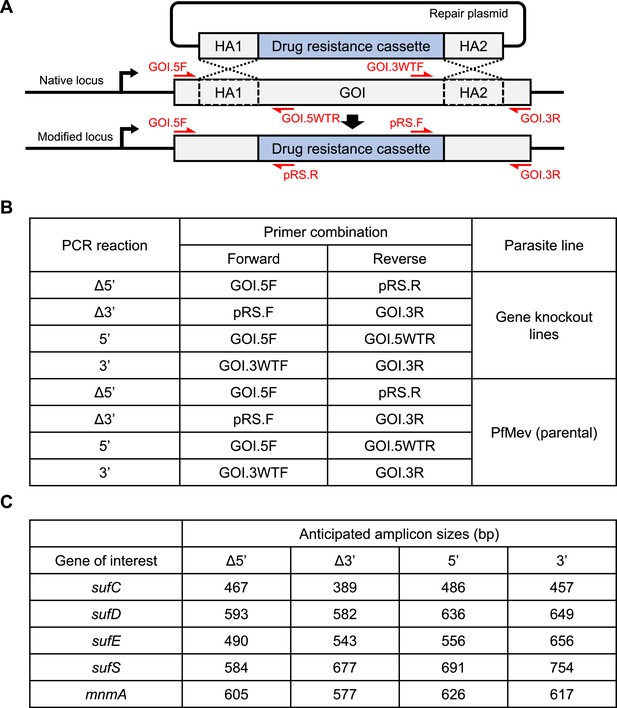
Generation and characterization of knockout parasite lines.
(A) Schematic illustration of a double crossover homologous recombination event between the native locus of gene of interest (GOI) and the repair plasmid with two homology arms (HAs). The Cas9-endonuclease promotes a double-stranded break in the native locus while the HAs from the repair plasmid recombine through a double crossover recombination event resulting in the generation of the recombinant locus (‘modified locus’). Individual segments are not to scale. The positions and directions of the primers (red arrow) used for confirmation of gene knockout are indicated. Primer sequences are available in Supplementary file 1d. (B) Table showing the primer pairs and the template used for each PCR. (C) Table showing the expected amplicon sizes for the genotyping PCRs shown in Figure 1B, F, Figure 2A, Figure 3I, Figure 5A, Figure 6A, and Figure 7B.
-
Figure 1—figure supplement 1—source data 1
Table used for Figure 1—figure supplement 1B.
- https://cdn.elifesciences.org/articles/84491/elife-84491-fig1-figsupp1-data1-v2.xlsx
-
Figure 1—figure supplement 1—source data 2
Table used for Figure 1—figure supplement 1C.
- https://cdn.elifesciences.org/articles/84491/elife-84491-fig1-figsupp1-data2-v2.xlsx
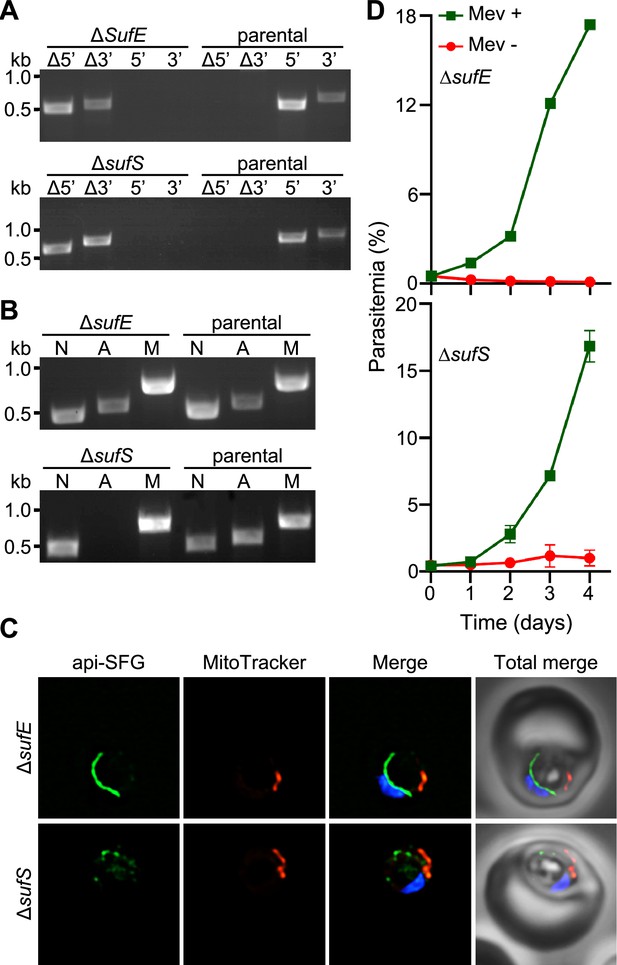
Apicoplast cysteine desulfurase, SufS, is essential for apicoplast maintenance.
(A) Genotyping PCR confirming deletion of sufE (top panel) or sufS (bottom panel) in PfMev parasites. In both ΔsufE and ΔsufS parasite lines, gene deletions were validated by the presence of PCR products for the Δ5’ and Δ3’ loci, but not for the unmodified loci (5’ and 3’) found in the PfMev (parental) line. Genotyping PCRs and expected amplicon sizes are described in Figure 1—figure supplement 1. (B) Attempted PCR amplification of ldh, sufB, and cox1 genes of the parasite nuclear (N), apicoplast (A), and mitochondrial (M) genomes, respectively, from the ΔsufE, ΔsufS, and PfMev (parental) parasites. Successful amplification of sufB in the ΔsufE (top panel) parasites indicates the presence of the apicoplast genome, while failure to amplify this gene in ΔsufS parasites (bottom panel) indicates loss of the apicoplast genome. (C) Representative epifluorescence microscopy images of ΔsufE (top panel) and ΔsufS (bottom panel) parasites. In the ΔsufE parasites, a single intact apicoplast is seen. Whereas in ΔsufS multiple discrete vesicles were seen, indicating disruption of the apicoplast organelle. The api-SFG protein (green) labels the apicoplast, MitoTracker (red) stains the mitochondrion, and nuclear DNA is stained with DAPI (blue). Each image is a field representing 10 µm × 10 µm. (D) Mevalonate (Mev)-dependent growth of ΔsufE (top panel) and ΔsufS (bottom panel) parasites. Asynchronous parasites from each line were cultured with or without 50 μM Mev and parasitemia was monitored every 24 hr by flow cytometry for 4 days. Data points showing daily mean parasitemia ± SEM from two independent biological replicates, each with four technical replicates. In panels (A) and (B), DNA markers are in kilobases (kb).
-
Figure 2—source data 1
Uncropped agarose gel images of PCR analyses presented in Figure 2A and B.
- https://cdn.elifesciences.org/articles/84491/elife-84491-fig2-data1-v2.zip
-
Figure 2—source data 2
∆sufE (top table) and ∆sufS (bottom table) growth assay parasitemia counts used for Figure 2D.
- https://cdn.elifesciences.org/articles/84491/elife-84491-fig2-data2-v2.xlsx
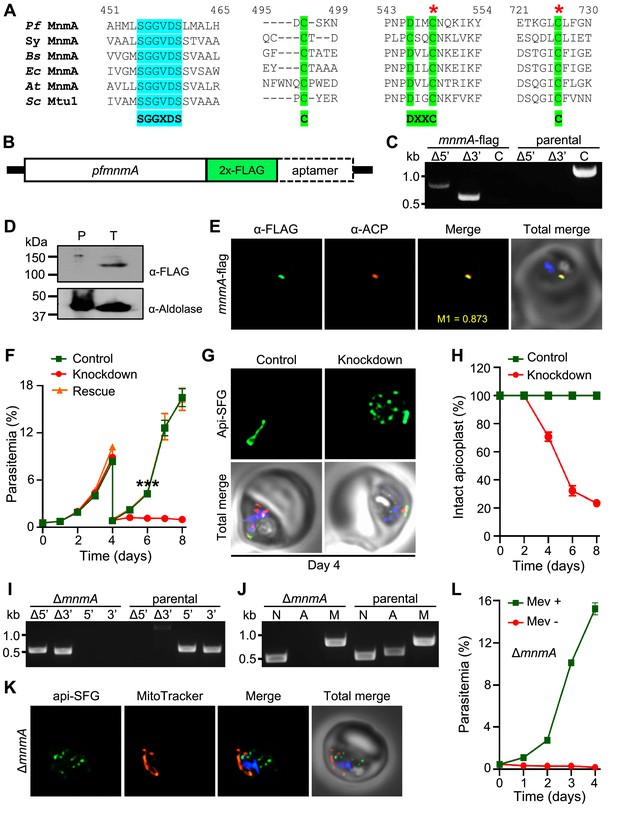
Plasmodium falciparum MnmA is essential for apicoplast maintenance and parasite survival.
(A) Snapshots from the multiple sequence alignment of MnmA orthologs from P. falciparum (Pf MnmA), Synechococcus sp. (Sy MnmA), B. subtilis (Bs MnmA), E. coli (Ec MnmA), A. thaliana (At MnmA), and S. cerevisiae (Sc Mtu1) showing the conserved sequence motifs in the catalytic domains. The PP-loop motif SGGXDS shown in teal and the D-type motif C-DXXC-C in bright green. The red asterisks indicate catalytic cysteines. (B) Expected modified locus following introduction of 2xFLAG at 3’-end of pfmnmA and 10xAptamer in the 3’UTR. (C) Genotyping of mnmA-flag parasites confirms plasmid integration as evidenced by the presence of PCR amplicons for the recombinant Δ5’ and Δ3’ loci but not for the unmodified locus (C), as compared to the PfMevattB (parental) line. Expected PCR amplicon sizes are provided in Figure 3—figure supplement 4B. (D) Immunoblot of saponin-isolated PfMevattB (parental, P) and mnmA-flag (tagged, T) parasite lysates with anti-FLAG antibody (top panel). Anticipated molecular weight for the tagged protein is 120 kDa. Anti-aldolase immunoblot shows relative loading levels (bottom panel). Protein markers are in kilodaltons (kDa). The uncropped version is shown in Figure 3—figure supplement 4C. (E) Representative immunofluorescence microscopy images show Pf MnmA-2xFLAG (green) colocalization with the apicoplast marker ACP (acyl carrier protein, red). Manders’ coefficient (M1, green in red) quantifies the degree of colocalization. (F) Anhydrous tetracycline (aTc)-dependent growth of mnmA-flag parasites. Asynchronous parasites were grown with 0.5 µM aTc (control), without 0.5 µM aTc (knockdown), or with 50 μM mevalonate (Mev, rescue). Parasitemia was monitored via flow cytometry every 24 hr for 8 days. On day 4, parasite cultures were diluted 1:10. Data points show daily mean parasitemia ± SEM from two independent biological replicates, each with four technical replicates; two-way ANOVA (Sidak-Bonferroni method), ***p<0.001. (G) Representative epifluorescence microscopy images of day four samples of mnmA-flag parasites grown in control (aTc added) and knockdown (aTc removed) conditions from the experiment shown in (F). Under control conditions, the apicoplast remains intact; under knockdown conditions, multiple discrete vesicles are seen, demonstrating a disrupted apicoplast. The full image panel is shown in Figure 3—figure supplement 6A. Representative images for mnmA-flag parasites grown in the rescue condition (aTc removed, Mev added) from the experiment shown in (F) are presented in Figure 3—figure supplement 6B. (H) Graph showing the percentage of mnmA-flag parasites with an intact apicoplast when grown in control (aTc added) and knockdown (aTc removed) conditions in the experiment shown in (F). Percentage of intact apicoplast in the rescue condition (aTc removed, Mev added) also mirrors the trend observed in the knockdown condition. Data shown in Figure 3—figure supplement 7. Live epifluorescence microscopy images were taken every 48 hr and apicoplast morphology (intact or disrupted) was determined based on apicoplast-localized api-SFG. Error bars represent standard deviation from at least 18 images taken for each time point and growth condition from two independent experiments. (I) Genotyping PCR confirming mnmA deletion in ΔmnmA parasites. In the ΔmnmA parasite line, gene deletion was validated by the presence of PCR products for the Δ5’ and Δ3’ loci, but not for the unmodified loci (5’ and 3’) found in the PfMev (parental) line. Genotyping PCRs and expected amplicon sizes are described in Figure 1—figure supplement 1. (J) Attempted PCR amplification of ldh, sufB, and cox1 genes of the parasite nuclear (N), apicoplast (A), and mitochondrial (M) genomes, respectively, from the ΔmnmA and PfMev (parental) parasites. Lack of a PCR amplicon for sufB in the ΔmnmA parasite line suggests loss of the apicoplast genome. (K) Representative epifluorescence microscopy images of ΔmnmA parasites. Multiple discrete vesicles are seen, demonstrating a disrupted apicoplast. (L) Mevalonate (Mev)-dependent growth of the ΔmnmA parasites. Asynchronous parasites were cultured with or without 50 μM Mev for 4 days. Parasitemia was monitored every 24 hr by flow cytometry. Data points show daily mean parasitemia ± SEM from two independent biological replicates, each with four technical replicates. In panels (G) and (K), api-SFG protein (green) labels the apicoplast, the mitochondrion is stained with MitoTracker (red), and nuclear DNA is stained with DAPI (blue). In (E), (G), and (K), each image depicts a field of 10 µm × 10 µm. In (C), (I), and (J), DNA markers are in kilobases (kb).
-
Figure 3—source data 1
Uncropped agarose gel images of PCR analyses presented in Figure 3C, I, and J.
- https://cdn.elifesciences.org/articles/84491/elife-84491-fig3-data1-v2.zip
-
Figure 3—source data 2
mnmA-flag growth assay parasitemia counts used for Figure 3F.
The yellow highlighted row represents the expected parasitemia count after 1:10 dilution of day 4 parasites.
- https://cdn.elifesciences.org/articles/84491/elife-84491-fig3-data2-v2.xlsx
-
Figure 3—source data 3
Counts of intact apicoplasts presented in Figure 3(H).
- https://cdn.elifesciences.org/articles/84491/elife-84491-fig3-data3-v2.xlsx
-
Figure 3—source data 4
∆mnmA growth assay parasitemia counts used for Figure 3L.
- https://cdn.elifesciences.org/articles/84491/elife-84491-fig3-data4-v2.xlsx
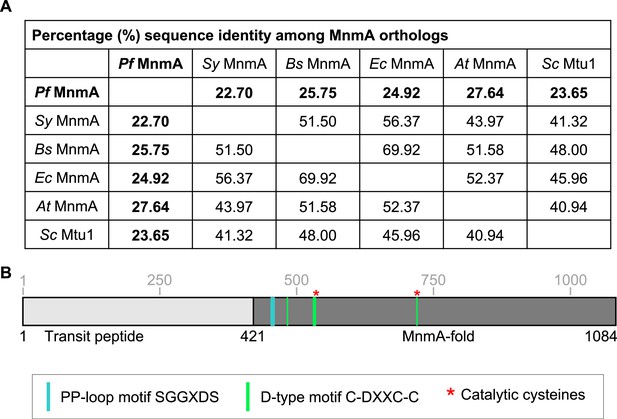
Sequence identity among MnmA orthologs.
(A) Table showing the pairwise sequence identity of MnmA orthologs from P. falciparum (Pf MnmA), Synechococcus sp. (Sy MnmA), B. subtilis (Bs MnmA), E. coli MnmA (Ec MnmA), A. thaliana (At MnmA), and S. cerevisiae (Sc Mtu1). The multiple sequence alignment is shown in Figure 3—figure supplement 1—source data 1. (B) Schematic of Pf MnmA showing the conserved sequence motifs and the catalytic cysteines. Schematic drawn to scale.
-
Figure 3—figure supplement 1—source data 1
Multiple sequence alignment used for Figure 3A and Figure 3—figure supplement 1.
- https://cdn.elifesciences.org/articles/84491/elife-84491-fig3-figsupp1-data1-v2.pdf
-
Figure 3—figure supplement 1—source data 2
Table used for Figure 3—figure supplement 1A.
- https://cdn.elifesciences.org/articles/84491/elife-84491-fig3-figsupp1-data2-v2.xlsx
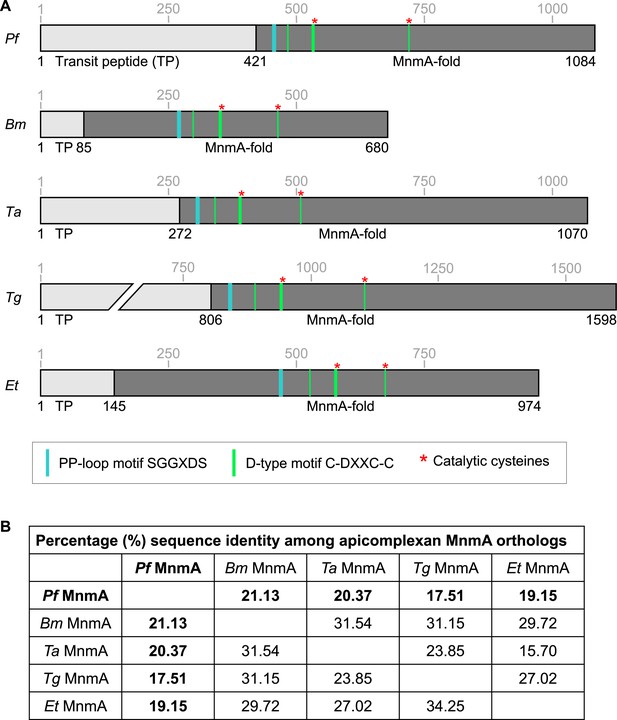
MnmA orthologs from pathogenic apicomplexans.
(A) Schematics of MnmA orthologs from P. falciparum (Pf), Babesia microti (Bm), Theileria annulata (Ta), T. gondii (Tg), and Eimeria tenella (Et) showing the conserved sequence motifs and the catalytic cysteines. (B) Table showing the pairwise sequence identity of MnmA orthologs from P. falciparum (Pf MnmA), B. microti (Bm MnmA), T. annulata (Ta MnmA), T. gondii (Tg MnmA), and E. tenella (Et MnmA). The multiple sequence alignment is shown in Figure 3—figure supplement 2—source data 1.
-
Figure 3—figure supplement 2—source data 1
Multiple sequence alignment used for Figure 3—figure supplement 2.
- https://cdn.elifesciences.org/articles/84491/elife-84491-fig3-figsupp2-data1-v2.pdf
-
Figure 3—figure supplement 2—source data 2
Table used for Figure 3—figure supplement 2B.
- https://cdn.elifesciences.org/articles/84491/elife-84491-fig3-figsupp2-data2-v2.xlsx
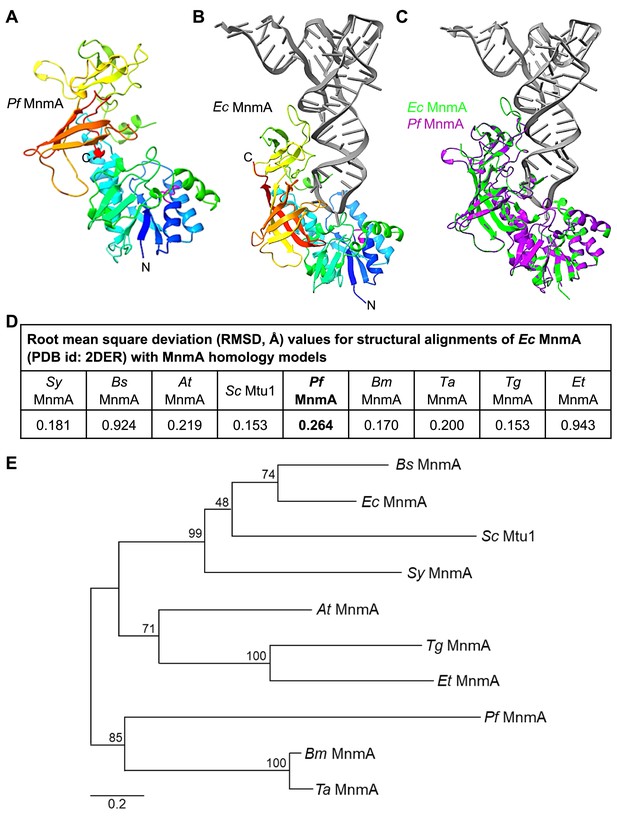
The C-terminal domain of P. falciparum MnmA is predicted to have the same protein fold as bacterial MnmA.
(A) A homology model of the Pf MnmA C-terminal domain (residues 422–1084) is shown colored by sequence position (blue to red). The PP-loop motif SGGXDS (Ser455-Ser460) is shown in magenta. (B) The crystal structure of Ec MnmA with the tRNA in gray (PDB id: 2DER) is shown with the same coloring scheme used in panel (A). The PP-loop motif SGGXDS (Ser13-Ser18) is shown in magenta. (C) Superimposition of the structures shown in panels (A) and (B). (D) Table showing the root mean square deviation (RMSD) of alpha carbon positions between the homology models of MnmA orthologs and the Ec MnmA crystal structure. Sy, Synechococcus sp.; Bs, B. subtilis; At, A. thaliana; Sc, S. cerevisiae; Bm, B. microti; Ta, T. annulata; Tg, T. gondii; Et, E. tenella. (E) The phylogenetic relationship of the MnmA proteins shows no particular clustering among the MnmA orthologs that directly receive sulfur from a corresponding cysteine desulfurase. Bootstrap analyses were performed for 1000 replicates and the percentage of trees in which the associated taxa clustered together is shown next to the branches. The tree is drawn to scale, with branch lengths measured in the number of substitutions per site. The multiple sequence alignment use for this analysis is shown in Figure 3—figure supplement 3—source data 2.
-
Figure 3—figure supplement 3—source data 1
Table used for Figure 3—figure supplement 3D.
- https://cdn.elifesciences.org/articles/84491/elife-84491-fig3-figsupp3-data1-v2.xlsx
-
Figure 3—figure supplement 3—source data 2
Multiple sequence alignment used for Figure 3—figure supplement 3E.
- https://cdn.elifesciences.org/articles/84491/elife-84491-fig3-figsupp3-data2-v2.pdf
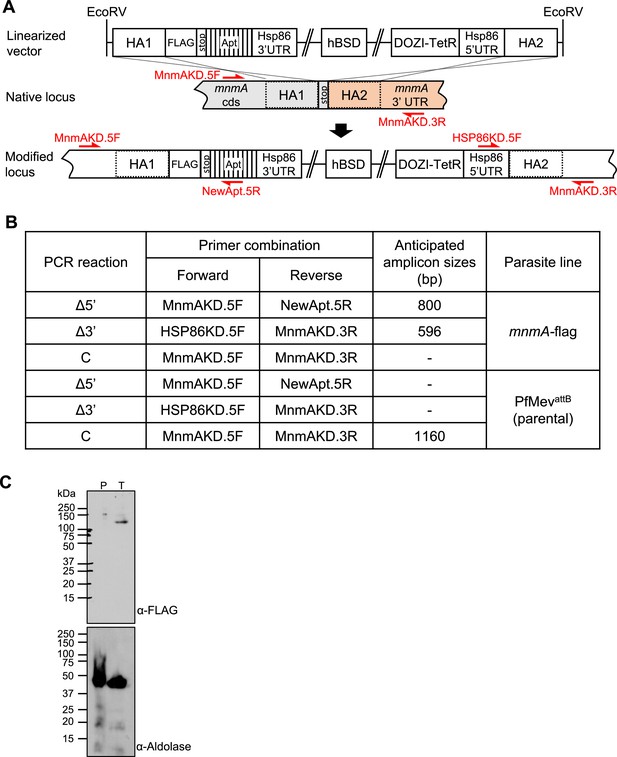
Generation of the MnmA localization/knockdown construct.
(A) Schematic showing the insertion of 2x-FLAG at the 3’-end and aptamers in the 3’UTR of pfmnmA. The pKD-mnmA-2xFLAG-10xapt plasmid was linearized with EcoRV digestion. The homology arms (HA) on the plasmid corresponding to the native mnmA locus are shown, indicating how homologous recombination occurs. Primer positions for insertion confirmation PCR are shown with red arrows. Primer sequences are available in Supplementary file 1c. Individual segments are not to scale. HA, homology arm; cds, coding sequence; UTR, untranslated region; hBSD, codon-harmonized blasticidin deaminase. (B) Table showing the anticipated amplicon sizes for insertion confirmation PCRs shown in Figure 3C. (C) Full panel of immunoblot shown in Figure 3D. Immunodetection of saponin-isolated PfMevattB (parental, P) and mnmA-flag (tagged, T) parasite lysates. These lysates were probed with an anti-FLAG antibody (top panel). The anticipated molecular weight for the tagged protein is 120 kDa. These lysates were also probed with an anti-aldolase antibody to show relative loading levels (bottom panel). Protein markers are in kilodaltons (kDa).
-
Figure 3—figure supplement 4—source data 1
Table used for Figure 3—figure supplement 4B.
- https://cdn.elifesciences.org/articles/84491/elife-84491-fig3-figsupp4-data1-v2.xlsx
-
Figure 3—figure supplement 4—source data 2
Full immunoblot shown in Figure 3—figure supplement 4C.
The red boxes correspond to the cropped images in Figure 3—figure supplement 4C.
- https://cdn.elifesciences.org/articles/84491/elife-84491-fig3-figsupp4-data2-v2.zip
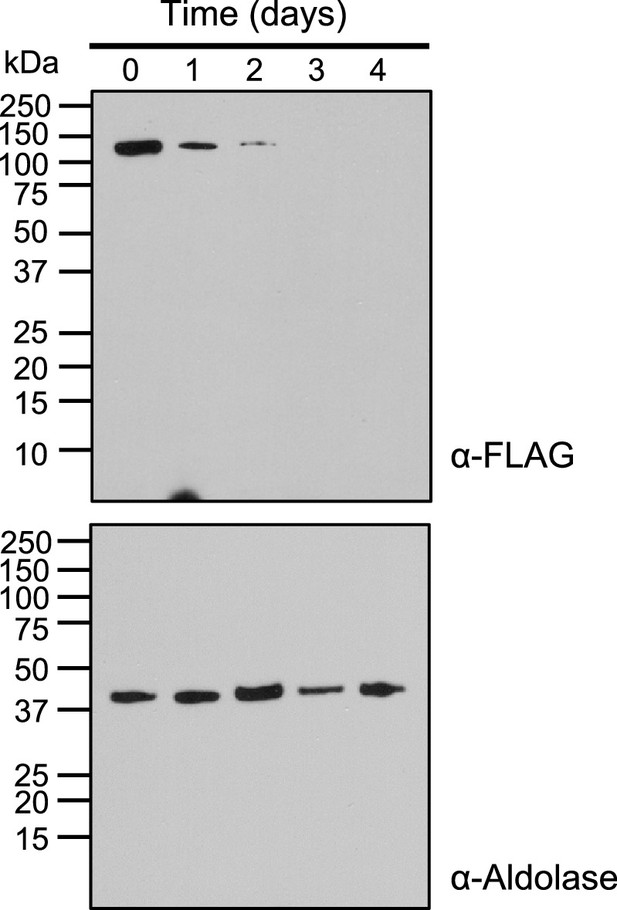
Effect of aTc removal on Pf MnmA in the mnmA-flag line.
Immunodetection of mnmA-flag in lysates of saponin-isolated parasites. Parasites were grown under non-permissive conditions (aTc removed) with samples collected daily for western blot. MnmA-2xFLAG protein was detected with anti-FLAG antibody (top panel). The blot was washed and reprobed with an anti-aldolase antibody to show relative loading levels (bottom panel). Protein markers are in kilodaltons (kDa).
-
Figure 3—figure supplement 5—source data 1
Full immunoblot shown in Figure 3—figure supplement 5.
The red boxes correspond to the cropped images in Figure 3—figure supplement 5. Blots were inverted for correct orientation.
- https://cdn.elifesciences.org/articles/84491/elife-84491-fig3-figsupp5-data1-v2.zip
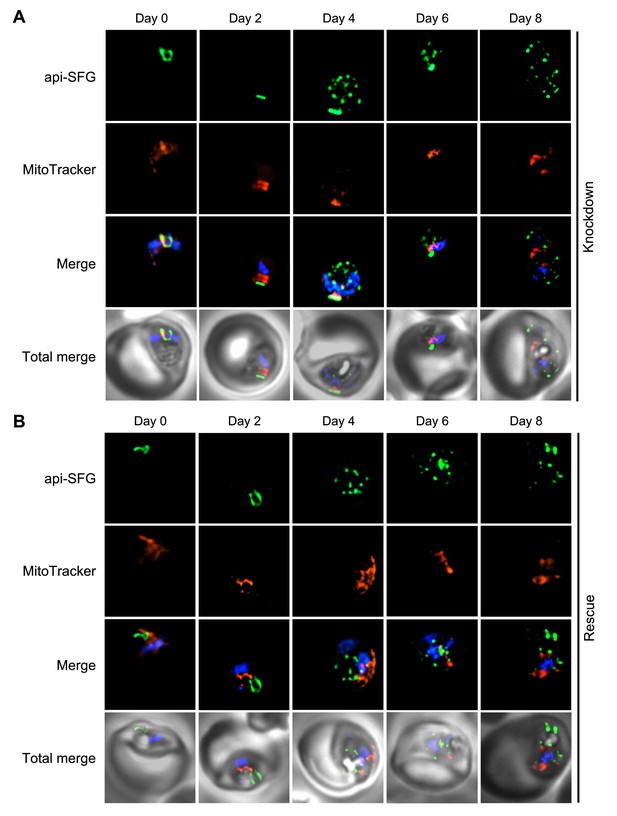
Live epifluorescence microscopy shows the effect on apicoplast morphology in the mnmA-flag line after aTc removal.
(A) Live epifluorescence microscopy of day 0, 2, 4, 6, and 8 samples from knockdown growth condition for the mnmA-flag parasite line. (B) Live epifluorescence microscopy of day 0, 2, 4, 6, and 8 samples from the rescue growth condition for the mnmA-flag parasite line. In both panels (A) and (B), from day 4 onward, multiple discrete vesicles were observed instead of a the single intact apicoplast organelle observed prior to day 4. The api-SFG protein (green) marks the apicoplast, the mitochondrion is labeled by MitoTracker (red), and nuclear DNA is stained with DAPI (blue). Representative images are shown. Each image depicts a field of 10 µm × 10 µm.
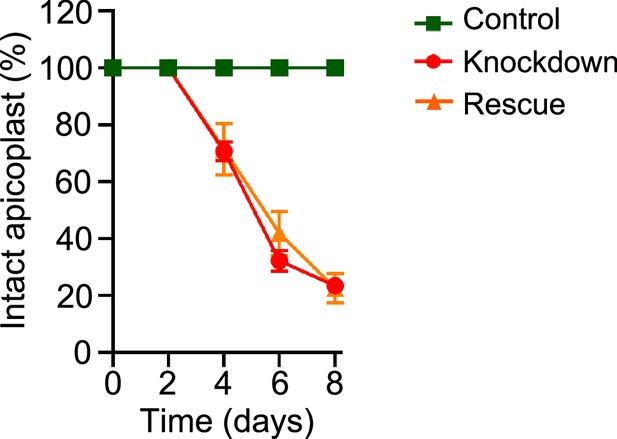
Percentage of intact apicoplast in the mnmA-flag line after aTc removal.
Graph showing the percentage of mnmA-flag parasites with an intact apicoplast when grown in control (aTc added), knockdown (aTc removed), and rescue (aTc removed, Mev added) conditions in the experiment shown in Figure 3F. Live epifluorescence microscopy images were taken every 48 hr and apicoplast morphology (intact or disrupted) was determined based on apicoplast-localized api-SFG. Error bars represent standard deviation from at least 18 images taken for each time point and growth condition from two independent experiments.
-
Figure 3—figure supplement 7—source data 1
Counts of intact apicoplasts presented in Figure 3—figure supplement 7.
- https://cdn.elifesciences.org/articles/84491/elife-84491-fig3-figsupp7-data1-v2.xlsx
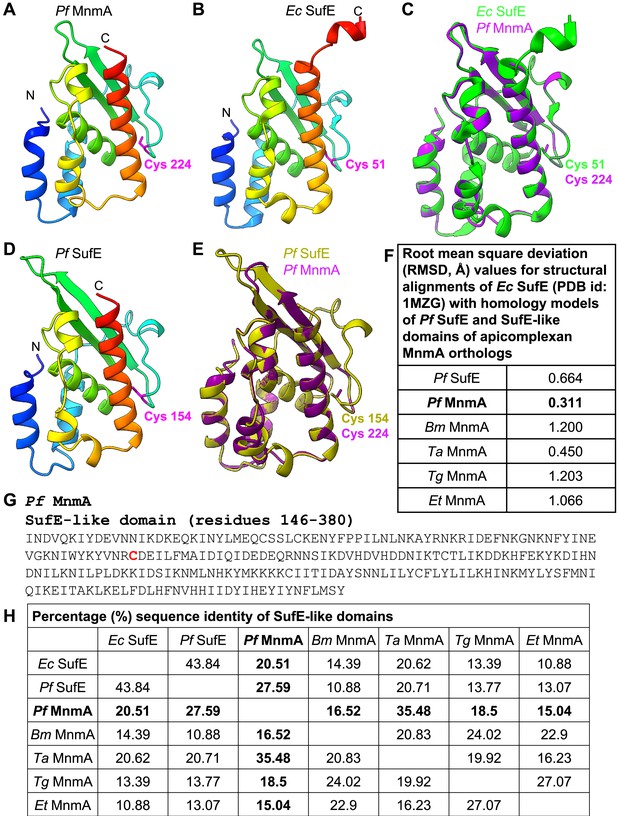
The N-terminal region of Pf MnmA contains a predicted SufE-like domain.
(A) A homology model of the Pf MnmA N-terminal domain (residues 146–380) is shown colored by sequence position (blue to red). The catalytic cysteine (Cys224) predicted to receive sulfane sulfur (Outten et al., 2003) is shown in stick representation (magenta). (B) The crystal structure of Ec SufE (PDB id: 1MZG) is shown with the same coloring scheme used in panel (A). The catalytic cysteine (Cys51) is shown in stick representation (magenta). (C) Superimposition of the structures shown in panels (A) and (B). (D) A homology model of Pf SufE (residues 101–249) is shown with the same coloring scheme used in panel (A). The catalytic cysteine (Cys154) is shown in stick representation (magenta). (E) Superimposition of the structures shown in panels (A) and (D). (F) Table showing the root mean square deviation (RMSD) values between the homology models of SufE-like domains and the Ec SufE crystal structure. Pf, P. falciparum; Bm, B. microti; Ta, T. annulata; Tg, T. gondii; Et, E. tenella. (G) The amino acid sequence of the N-terminal domain of Pf MnmA (residues 146–380). The catalytic cysteine (Cys224) predicted to receive sulfane sulfur is in bold red font. (H) Table showing the pairwise sequence identity of SufE proteins and SufE-like domains based on the multiple sequence alignment shown in Figure 3—figure supplement 8—source data 3.
-
Figure 3—figure supplement 8—source data 1
Table used for Figure 3—figure supplement 8F.
- https://cdn.elifesciences.org/articles/84491/elife-84491-fig3-figsupp8-data1-v2.xlsx
-
Figure 3—figure supplement 8—source data 2
Table used for Figure 3—figure supplement 8H.
- https://cdn.elifesciences.org/articles/84491/elife-84491-fig3-figsupp8-data2-v2.xlsx
-
Figure 3—figure supplement 8—source data 3
Multiple sequence alignment used for Figure 3—figure supplement 8H.
- https://cdn.elifesciences.org/articles/84491/elife-84491-fig3-figsupp8-data3-v2.pdf
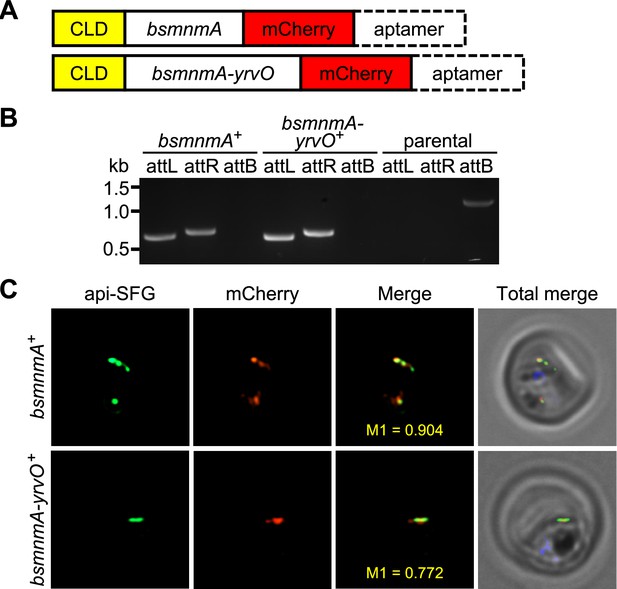
Bacillus subtilis MnmA and MnmA-YrvO can be expressed in the parasite apicoplast.
(A) Schematics illustrating the expected gene product in the bsmnmA+ and bsmnmA-yrvO+ parasite lines following successful genetic modification. CLD, conditional localization domain. (B) Amplification of the attL and attR regions from bsmnmA+ and bsmnmA-yrvO+ transgenic parasites indicates successful plasmid integration in both lines. Amplification of the attB region from the PfMevattB parasite line (parental) was used as a control. DNA markers are in kilobases (kb). Expected PCR amplicon sizes are provided in Figure 4—figure supplement 1B. (C) Representative epifluorescence microscopy images of bsmnmA+ (top panel) and bsmnmA-yrvO+ (bottom panel) transgenic parasites. The bsmnmA and bsmnmA-yrvO fusion protein contains a C-terminal mCherry tag (red) and colocalizes with the apicoplast api-SFG marker (green). The nuclear DNA is stained with DAPI (blue). The degree of colocalization of the bsmnmA or bsmnmA-yrvO fusion proteins with the api-SFG apicoplast marker are shown in the merge images and the Manders’ coefficients (M1; red in green) are provided. Each image depicts a field of 10 μm ×10 μm.
-
Figure 4—source data 1
Uncropped agarose gel images of PCR analyses presented in Figure 4B.
- https://cdn.elifesciences.org/articles/84491/elife-84491-fig4-data1-v2.zip
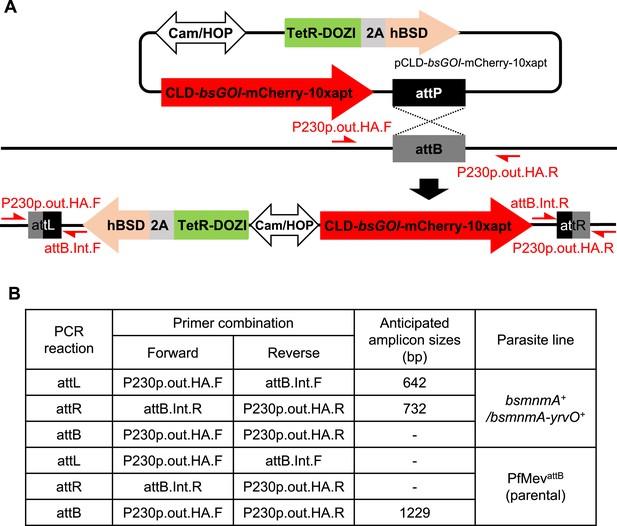
Generation and characterization of parasite lines expressing B. subtilis MnmA and MnmA-YrvO.
(A) Schematic illustration showing insertion of the pCLD-bsGOI-mCherry-10xapt plasmids into the attB locus of PfMevattB parasites. The TetR-DOZI inducible system regulator and harmonized blasticidin-S-deaminase (hBSD) are separated by a T2A viral skip peptide. The CLD-bsGOI-mCherry-10xapt and TetR-DOZI-2A-hBSD cassettes are expressed under a single bidirectional promoter. This plasmid was co-transfected with the pINT plasmid, which expresses integrase for catalyzing attB/attP integration. Primer pairs (in red) for PCR amplification of the attB region of the parental line and the recombinant attL and attR regions are shown. Primer sequences are available in Supplementary file 1c. bsGOI, B. subtilis genes of interest: mnmA or mnmA-yrvO fusion. (B) Table showing the anticipated amplicon sizes for insertion confirmation PCRs shown in Figure 4B.
-
Figure 4—figure supplement 1—source data 1
Table used for Figure 4—figure supplement 1B.
- https://cdn.elifesciences.org/articles/84491/elife-84491-fig4-figsupp1-data1-v2.xlsx
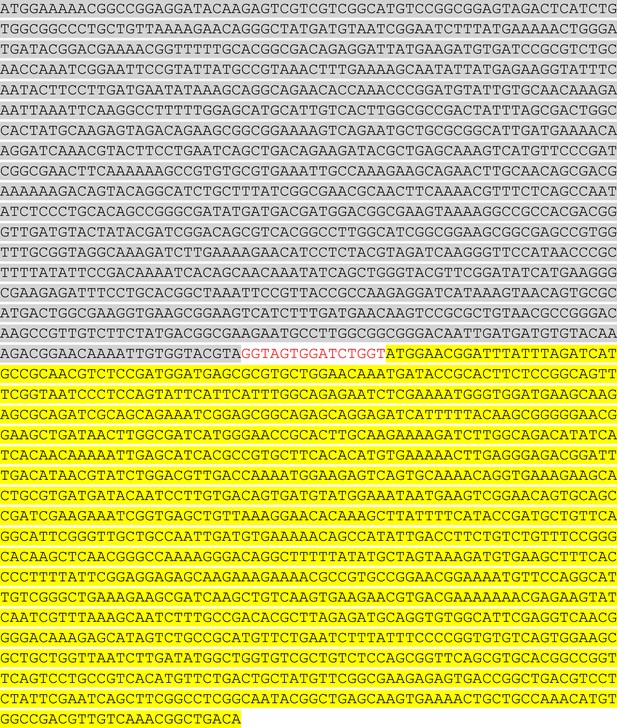
The engineered sequence encoding the B. subtilis MnmA-YrvO fusion protein.
The two genes were linked with a 15 bp linker (red font) and was used for generation of pCLD-bsmnmA-yrvO-mCherry-10xapt plasmid. Highlighted in gray is the sequence for the B. subtilis mnmA, with the sequence for the B. subtilis yrvO highlighted in yellow.
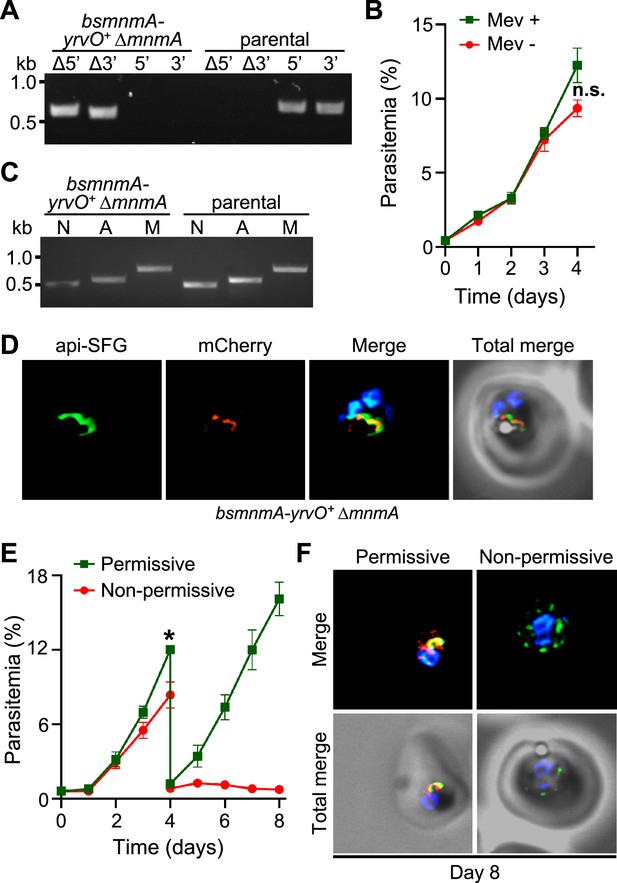
Bacillus subtilis MnmA-YrvO fusion protein can complement P. falciparum MnmA.
(A) Genotyping PCR confirms mnmA deletion in bsmnmA-yrvO+ ∆mnmA parasites. Successful deletion was validated by the presence of PCR amplicons for the Δ5’ and Δ3’ loci, but not for the unmodified loci (5’ and 3’) found in the bsmnmA-yrvO+ (parental) parasites. Genotyping PCRs and expected amplicon sizes are described in Figure 1—figure supplement 1. (B) Mevalonate (Mev)-independent growth of bsmnmA-yrvO+ ∆mnmA parasites. Asynchronous parasites were grown with or without 50 μM Mev. Every 24 hr parasitemia was monitored by flow cytometry for 4 days. Data points show daily mean parasitemia ± SEM from two independent biological replicates, each with four technical replicates; n.s., non-significant, two-way ANOVA (Sidak-Bonferroni method), p>0.05. (C) Attempted PCR amplification of ldh, sufB, and cox1 genes of the parasite nuclear (N), apicoplast (A), and mitochondrial (M) genomes, respectively, from bsmnmA-yrvO+ ΔmnmA and bsmnmA-yrvO+ (parental) parasites. Successful amplification of sufB in bsmnmA-yrvO+ ΔmnmA parasites indicates the presence of the apicoplast genome. (D) Representative epifluorescence microscopy images of bsmnmA-yrvO+ ΔmnmA parasites showing an intact apicoplast. (E) Growth of the bsmnmA-yrvO+ ΔmnmA parasites. Asynchronous parasites were grown under permissive (with 0.5 µM aTc and without 0.5 µM Shield1) or non-permissive (without 0.5 µM aTc and with 0.5 µM Shield1) conditions. Parasitemia was monitored via flow cytometry every 24 hr for 8 days. On day 4, parasite cultures were diluted 1:10. Data points showing daily mean parasitemia ± SEM from two independent biological replicates, each with four technical replicates; two-way ANOVA (Sidak-Bonferroni method), *p<0.05. (F) Representative epifluorescence microscopy images of day 8 bsmnmA-yrvO+ ΔmnmA parasites from (E). The apicoplast remains intact under permissive conditions, whereas multiple discrete vesicles were observed under non-permissive conditions. The Bs MnmA-YrvO-mCherry (red) is only visible under permissive conditions. The full image panel is available in Figure 5—figure supplement 1. In (D) and (F), the Bs MnmA-YrvO fusion protein contains a C-terminal mCherry fluorescent protein (red). Api-SFG protein (green) labels the apicoplast, and nuclear DNA is stained with DAPI (blue). Each image depicts a field of 10 µm × 10 µm. In (A) and (C), DNA markers are in kilobases (kb).
-
Figure 5—source data 1
Uncropped agarose gel images of PCR analyses presented in Figure 5A and C.
- https://cdn.elifesciences.org/articles/84491/elife-84491-fig5-data1-v2.zip
-
Figure 5—source data 2
bsmnmA-yrvO+∆mnmA growth assay parasitemia counts used for Figure 5B.
- https://cdn.elifesciences.org/articles/84491/elife-84491-fig5-data2-v2.xlsx
-
Figure 5—source data 3
bsmnmA-yrvO+∆mnmA growth assay parasitemia counts used for Figure 5E.
The yellow highlighted row represents the expected parasitemia count after 1:10 dilution of day 4 parasites.
- https://cdn.elifesciences.org/articles/84491/elife-84491-fig5-data3-v2.xlsx
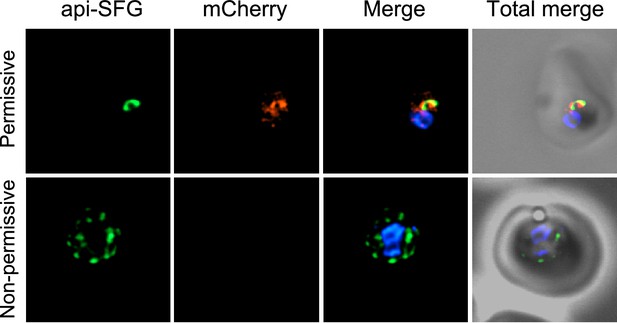
Live epifluorescence microscopy of day 8 samples from permissive and non-permissive growth conditions for bsmnmA-yrvO+ ΔmnmA.
The apicoplast remained intact under permissive conditions, while multiple discrete vesicles were observed under non-permissive conditions, indicative of a disrupted apicoplast organelle. The Bs mnmA-YrvO fusion protein contains a C-terminal mCherry fluorescent protein tag (red) which is only visible under permissive conditions. The api-SFG protein (green) labels the apicoplast, and nuclear DNA is stained with DAPI (blue). Representative images are shown. Each image depicts a field of 10 µm × 10 µm.
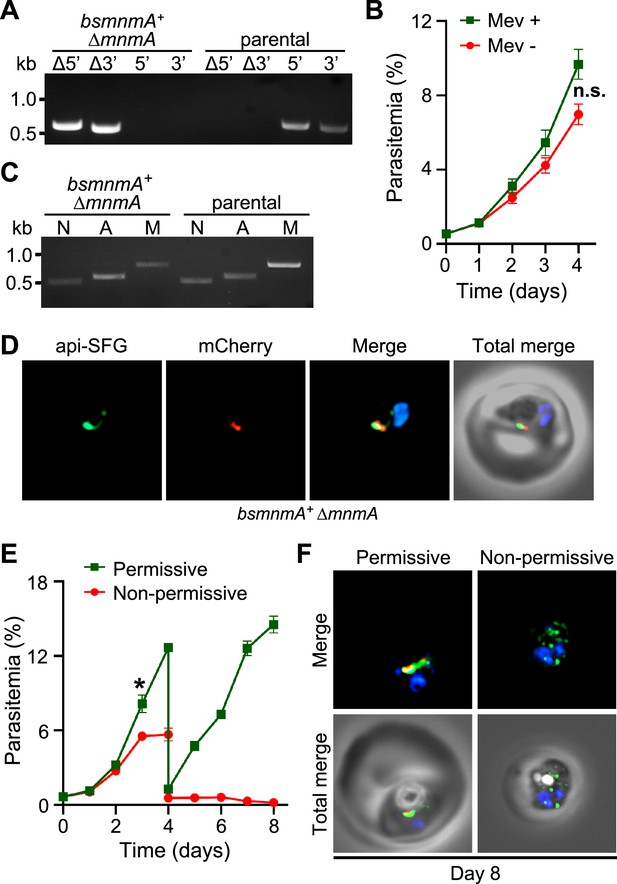
Bacillus subtilis MnmA can complement loss of P. falciparum MnmA.
(A) Genotyping PCR confirms mnmA deletion in bsmnmA+ ∆mnmA parasites, as evidenced by the presence of PCR amplicons for the Δ5’ and Δ3’ loci, but not for the unmodified loci (5’ and 3’) found in the bsmnmA+ (parental) parasites. Genotyping PCRs and expected amplicon sizes are described in Figure 1—figure supplement 1. (B) Mevalonate (Mev)-independent growth of bsmnmA + ∆mnmA parasites. Asynchronous parasites were grown with or without 50 μM Mev and parasitemia was monitored every 24 hr by flow cytometry for 4 days. Data points represent daily mean parasitemia ± SEM from two independent biological replicates, each with four technical replicates; n.s., non-significant, two-way ANOVA (Sidak-Bonferroni method), p>0.05. (C) PCR detection of ldh, sufB, and cox1 genes of the parasite nuclear (N), apicoplast (A), and mitochondrial (M) genomes, respectively, in bsmnmA+ ∆mnmA and bsmnmA+ (parental) parasites. Successful amplification of sufB in the bsmnmA+ ∆mnmA parasites indicates the presence of the apicoplast genome. (D) Representative epifluorescence microscopy images of bsmnmA+ ∆mnmA parasites shows an intact apicoplast. (E) Asynchronous bsmnmA+ ∆mnmA parasites were grown under permissive (with 0.5 µM aTc and without 0.5 µM Shield1) or non-permissive (without 0.5 µM aTc and with 0.5 µM Shield1) conditions. Parasitemia was monitored via flow cytometry every 24 hr for 8 days. On day 4, parasite cultures were diluted 1:10. Data points represent daily mean parasitemia ± SEM from two independent biological replicates, each with four technical replicates; two-way ANOVA (Sidak-Bonferroni method), *p<0.05. (F) Representative epifluorescence microscopy images of day 8 bsmnmA+ ∆mnmA parasites from (E). The apicoplast remained intact under permissive conditions, while multiple discrete vesicles were observed under non-permissive conditions, indicative of a disrupted apicoplast organelle. The Bs MnmA -mCherry (red) is only visible under permissive conditions. The full image is available in Figure 6—figure supplement 1. In (D) and (F), The Bs MnmA protein is tagged C-terminally with mCherry (red), api-SFG protein (green) labels the apicoplast, and nuclear DNA is stained with DAPI (blue). Each image depicts a field of 10 µm × 10 µm. In (A) and (C), DNA markers are in kilobases (kb).
-
Figure 6—source data 1
Uncropped agarose gel images of PCR analyses presented in Figure 6A and C.
- https://cdn.elifesciences.org/articles/84491/elife-84491-fig6-data1-v2.zip
-
Figure 6—source data 2
bsmnmA+ ∆mnmA growth assay parasitemia counts used for Figure 6B.
- https://cdn.elifesciences.org/articles/84491/elife-84491-fig6-data2-v2.xlsx
-
Figure 6—source data 3
bsmnmA+ ∆mnmA growth assay parasitemia counts used for Figure 6E.
The yellow highlighted row represents the expected parasitemia count after 1:10 dilution of day 4 parasites.
- https://cdn.elifesciences.org/articles/84491/elife-84491-fig6-data3-v2.xlsx
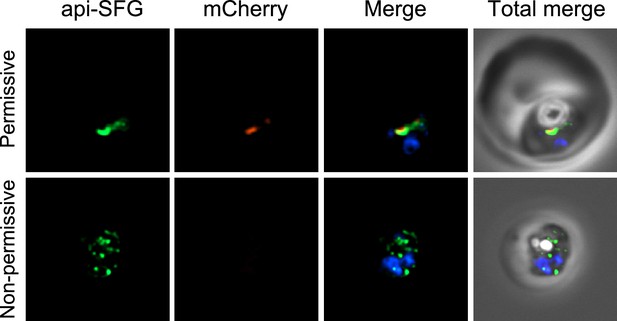
Live epifluorescence microscopy of day 8 samples from permissive and non-permissive growth conditions for bsmnmA + ΔmnmA parasites.
The apicoplast remained intact under permissive conditions, while multiple discrete vesicles were observed under non-permissive conditions, indicative of a disrupted apicoplast organelle. The Bs MnmA protein contains a C-terminal mCherry fluorescent protein tag (red) which is only visible under permissive conditions. The api-SFG protein (green) labels the apicoplast, and nuclear DNA is stained with DAPI (blue). Representative images are shown. Each image depicts a field of 10 µm × 10 µm.
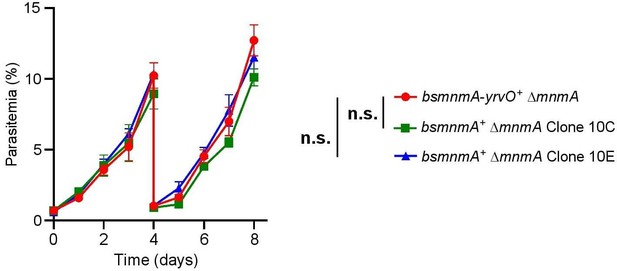
Growth comparison between the bsmnmA+ ∆mnmA parasite line and bsmnmA-yrvO+ ∆mnmA parasite line.
Asynchronous parasites were grown in complete medium with 0.5 µM aTc for 8 days. Parasite growth was monitored via flow cytometry every 24 hr. On day 4, parasite cultures were diluted 1:10. Data presented are from two independent biological replicates, each with four technical replicates, error bars represent the standard error of the mean; n.s., non-significant, two-way ANOVA (Sidak-Bonferroni method), p>0.05.
-
Figure 6—figure supplement 2—source data 1
Growth assay parasitemia counts used for Figure 6—figure supplement 2.
All parasites were cultured in presence of anhydrotetracycline (aTc). The yellow highlighted row represents the expected parasitemia count after 1:10 dilution of day 4 parasites.
- https://cdn.elifesciences.org/articles/84491/elife-84491-fig6-figsupp2-data1-v2.xlsx
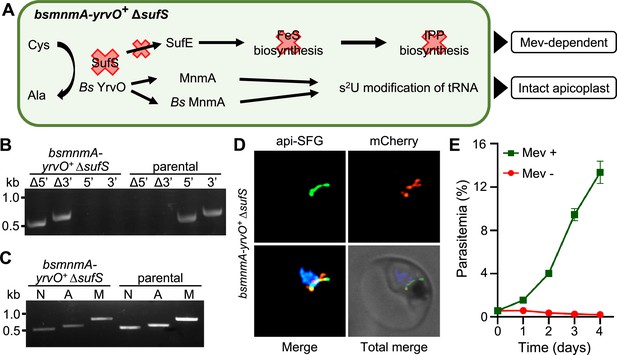
Plasmodium falciparum SufS provides sulfur for both the sulfur utilization factor (SUF) pathway and MnmA-catalyzed tRNA thiolation.
(A) The expected outcome of sufS deletion in the bsmnmA-yrvO+ line is explained in the schematic. In absence of parasite SufS, sulfur for FeS biosynthesis is unavailable resulting in no FeS synthesis. Since the isopentenyl pyrophosphate (IPP) precursor biosynthesis pathway contains FeS-dependent enzymes, this pathway cannot function, rendering parasites reliant on exogenous mevalonate (Mev) to survive. Sulfur liberated by Bs YrvO is sufficient for the MnmA-mediated s2U tRNA modification and efficient protein translation. (B) Genotyping PCR confirms deletion of sufS in bsmnmA-yrvO+ ∆sufS parasites. Successful deletion was validated by the presence of PCR amplicons for the Δ5’ and Δ3’ loci, but not for the unmodified loci (5’ and 3’) found in the bsmnmA-yrvO+ (parental) parasites. Genotyping PCRs and expected amplicon sizes are described in Figure 1—figure supplement 1. (C) Attempted PCR amplification of ldh, sufB, and cox1 genes from the parasite nuclear (N), apicoplast (A), mitochondrial (M) genomes, respectively, in bsmnmA-yrvO+ ∆sufS and bsmnmA-yrvO+ (parental) parasites. Successful amplification of sufB in the bsmnmA-yrvO+ ∆sufS parasite line indicates the presence of the apicoplast genome. (D) Representative epifluorescence microscopy images of the bsmnmA-yrvO+ ∆sufS parasite line expressing api-SFG protein (green) and the Bs MnmA-YrvO-mCherry (red). The nuclear DNA is stained with DAPI (blue). A single intact apicoplast was seen in this parasite. Each image depicts a field of 10 µm × 10 µm. (E) Mevalonate (mev)-dependent growth of bsmnmA-yrvO+ ∆sufS parasites. Asynchronous were cultured with or without 50 μM Mev for 4 days. Flow cytometry was used to monitor parasitemia every 24 hr. Data points represent daily mean parasitemia ± SEM from two independent biological replicates, each with four technical replicates. In panels (B) and (C), DNA markers are in kilobases (kb).
-
Figure 7—source data 1
Uncropped agarose gel images of PCR analyses presented in Figure 7B, C.
- https://cdn.elifesciences.org/articles/84491/elife-84491-fig7-data1-v2.zip
-
Figure 7—source data 2
bsmnmA-yrvO+ ∆sufS growth assay parasitemia counts used for Figure 7E.
- https://cdn.elifesciences.org/articles/84491/elife-84491-fig7-data2-v2.xlsx
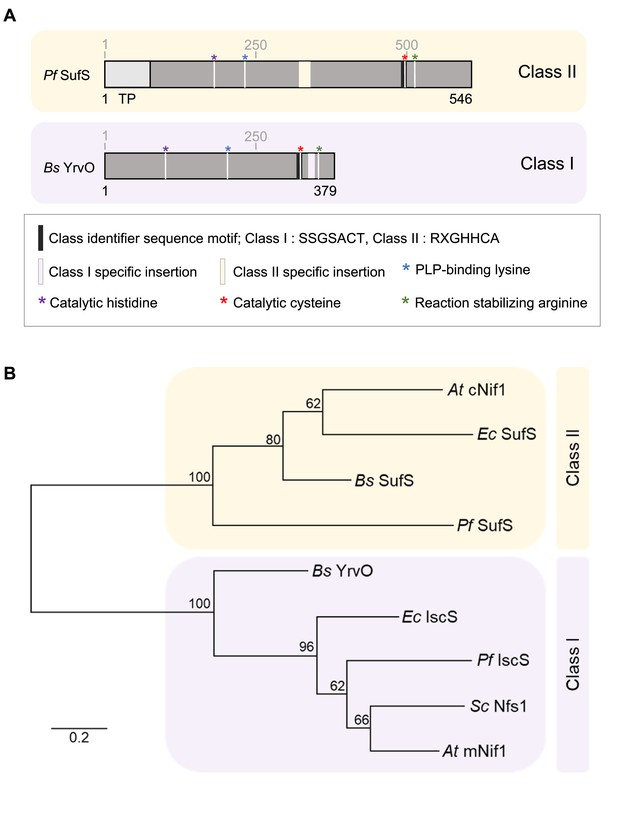
Phylogenetic relationship between P. falciparum SufS and B. subtilis YrvO.
(A) Schematics of P. falciparum SufS and B. subtilis YrvO showing the conserved motifs and catalytic residues. The schematics are drawn to scale. The pyridoxal 5'-phosphate (PLP) cofactor covalently binds to conserved lysine (K) and conserved arginine (R) involved in stabilizing the reaction intermediate. Black box represents conserved sequence motifs around the catalytic cysteine act as an identifier for two different classes of cysteine desulfurases: SSGSACT in Class I (shaded light purple) and RXGHHCA in Class II (shaded light yellow). (B) The phylogenetic relationship of cysteine desulfurases from P. falciparum (Pf SufS), B. subtilis (Bs SufS, Bs YrvO), E. coli (Ec SufS, Ec IscS), A. thaliana (At cNif1, At mNif1), and S. cerevisiae (Sc Nfs1). The cysteine desulfurase orthologs are seen to cluster into two main branches, which are defined as Class I (light purple shade) and II (light yellow shade). Bootstrap analyses were performed for 1000 replicates and the percentage of trees in which the associated taxa clustered together is shown next to the branches. The tree is drawn to scale, with branch lengths measured in the number of substitutions per site.
-
Figure 7—figure supplement 1—source data 1
Multiple sequence alignment of cysteine desulfurase orthologs.
(A) Multiple sequence alignment used for Figure 7—figure supplement 1. (B) Table showing the percentage sequence identity among cysteine desulfurase orthologs based on the sequence alignment shown in panel (A).
- https://cdn.elifesciences.org/articles/84491/elife-84491-fig7-figsupp1-data1-v2.pdf
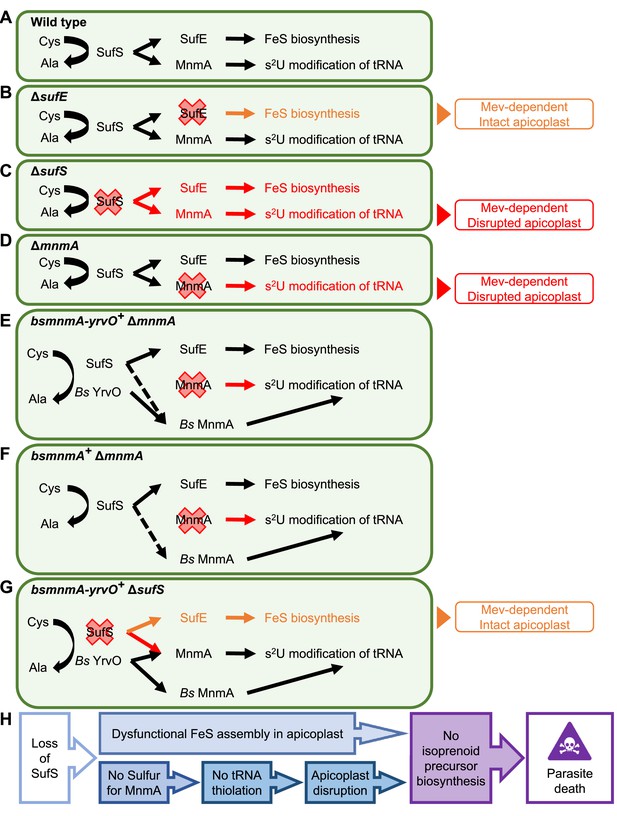
Dual role of SufS in the P. falciparum apicoplast.
(A) Dual role of SufS in FeS synthesis and s2U modification of tRNAs in the apicoplast of P. falciparum. (B) In ΔsufE parasites, deletion of sufE results in a mevalonate (Mev)-dependent phenotype with an intact apicoplast. This phenotype is likely caused by the deletion of SufE resulting in the loss of FeS biosynthesis. (C) In ΔsufS parasites, disruption of both FeS biosynthesis and MnmA-dependent tRNA thiolation results in a mevalonate (Mev)-dependent phenotype with a disrupted apicoplast. (D) In ΔmnmA parasites, deletion of mnmA results in a mevalonate (Mev)-dependent phenotype with a disrupted apicoplast. Deletion of MnmA likely causes tRNA thiolation defect leading to translational aberration and ultimately apicoplast disruption. (E) In bsmnmA-yrvO+ ΔmnmA parasites, both SufS and Bs YrvO have cysteine desulfurase activity that transfers sulfur to Bs MnmA in the absence of the endogenous parasite MnmA. (F) In bsmnmA+ ΔmnmA parasites, Bs MnmA complements the loss of the endogenous parasite MnmA. The possibility that SufS can transfer sulfur to Bs MnmA is represented by the dotted arrow. (G) In bsmnmA-yrvO+ ΔsufS parasites, Bs YrvO can transfer sulfur to Bs MnmA and/or parasite MnmA, but not to SufE and the FeS biosynthesis pathway. In this scenario, parasites would have intact apicoplasts due to proper tRNA thiolation but would be Mev-dependent due to loss of FeS biosynthesis. (H) Events leading to parasite death because of loss of SufS activity in the apicoplast. In panels, (A–G), Cys, cysteine; Ala, alanine.
Tables
Reagent type (species) or resource | Designation | Source or reference | Identifiers | Additional information |
---|---|---|---|---|
Gene (Plasmodium falciparum) | SufC | PlasmoDB (https://plasmodb.org) | PF3D7_1413500 | P. falciparum SufC gene |
Gene (P. falciparum) | SufD | PlasmoDB (https://plasmodb.org) | PF3D7_1103400 | P. falciparum SufD gene |
Gene (P. falciparum) | SufE | PlasmoDB (https://plasmodb.org) | PF3D7_0206100 | P. falciparum SufE gene |
Gene (P. falciparum) | SufS | PlasmoDB (https://plasmodb.org) | PF3D7_0716600 | P. falciparum SufS gene |
Gene (P. falciparum) | MnmA | PlasmoDB (https://plasmodb.org) | PF3D7_1019800 | P. falciparum MnmA gene |
Cell line (P. falciparum) | PfMev | Swift et al., 2020 https://doi.org/10.1371/journal.ppat.1008316 | Parasites with engineered cytosolic mevalonate pathway. Available from the Prigge laboratory, Johns Hopkins Bloomberg School of Public Health | |
Cell line (P. falciparum) | PfMevattB | Swift et al., 2021 https://doi.org/10.15252/embj.2020107247 | PfMev parasites with an attB site in the P230p locus. Available from the Prigge lab | |
Genetic reagent (P. falciparum) | ∆sufC | This paper | sufC gene deletion line in PfMev parental background. Available from the Prigge lab | |
Genetic reagent (P. falciparum) | ∆sufD | This paper | sufD gene deletion line in PfMev parental background. Available from the Prigge lab | |
Genetic reagent (P. falciparum) | ∆sufE | This paper | sufE gene deletion line in PfMev parental background. Available from the Prigge lab | |
Genetic reagent (P. falciparum) | ∆sufS | This paper | sufS gene deletion line in PfMev parental background. Available from the Prigge lab | |
Genetic reagent (P. falciparum) | ∆mnmA | This paper | mnmA gene deletion line in PfMev parental background. Available from the Prigge lab | |
Genetic reagent (P. falciparum) | ∆sufC/sufD | This paper | sufC and sufD double gene deletion line in PfMev parental background. Available from the Prigge lab | |
Genetic reagent (P. falciparum) | mnmA-flag | This paper | Parasites expressing C-terminally FLAG tagged endogenous Pf MnmA in the PfMevattB parental background. An aptamer array in the 3’ untranslated region (UTR) of mnmA allows inducible control. Available from the Prigge lab | |
Genetic reagent (P. falciparum) | bsmnmA+ | This paper | Parasites expressing the B. subtilis MnmA-mCherry fusion protein in the PfMevattB parental background. An aptamer array in the 3’ UTR of bsmnmA-mCherry allows inducible control. Available from the Prigge lab | |
Genetic reagent (P. falciparum) | bsmnmA-yrvO+ | This paper | Parasites expressing the B. subtilis MnmAYrvO-mCherry fusion in the PfMevattB parental background. An aptamer array in the 3’ UTR of bsmnmAyrvO-mCherry allows inducible control. Available from the Prigge lab | |
Genetic reagent (P. falciparum) | bsmnmA+ ∆mnmA | This paper | mnmA gene deletion line in bsmnmA+ parental background. Available from the Prigge lab | |
Genetic reagent (P. falciparum) | bsmnmA-yrvO+ ∆mnmA | This paper | mnmA gene deletion line in bsmnmA-yrvO + parental background. Available from the Prigge lab | |
Genetic reagent (P. falciparum) | bsmnmA-yrvO+ ∆sufS | This paper | sufS gene deletion line in bsmnmA-yrvO + parental background. Available from the Prigge lab | |
Chemical compound, drug | Racemic Mevalonolactone | MilliporeSigma, MO, USA | Catalogue no. (Cat#) M4667 | 50 µM |
Chemical compound, drug | WR99210 | Jacobus Pharmaceuticals, NJ, USA | 2.5 nM | |
Chemical compound, drug | DSM1 | BEI Resources, VA, USA | Cat# MRA-1161 | 1.5 μM |
Chemical compound, drug | Blasticidin S HCl | Corning Inc, NY, USA | Cat# 30–100-RB | 1.25 or 2.5 µg/mL |
Chemical compound, drug | Anhydrous tetracycline (aTc) | Cayman Chemical, MI, USA | Cat# 10009542 | 0.5 µM |
Chemical compound, drug | Shield1 | Aobious Inc, MA, USA | Cat# AOB1848 | 0.5 µM |
Software, algorithm | Prism V8.4 | GraphPad Software, CA, USA | Parasite growth assay data analysis | |
Software, algorithm | Volocity | PerkinElmer, MA, USA | Deconvolution and colocalization analysis of microscopy images | |
Software, algorithm | MEGA11 | Tamura et al., 2021 https://doi.org/10.1093/molbev/msab120 | Multiple sequence alignment and phylogenetic analysis | |
Software, algorithm | Phyre2 | https://www.sbg.bio.ic.ac.uk/servers/phyre2 | Homology model prediction server | |
Software, algorithm | UCSF ChimeraX (version: 1.3rc202111110135) | https://www.rbvi.ucsf.edu/chimerax | Molecular visualization program for structural model and structural alignment | |
Antibody | Anti-mouse horseradish peroxidase (HRP)-conjugated antibody (Sheep polyclonal) | MilliporeSigma, MO, USA | Cat# GENA931 | Immunoblot (1:10,000) |
Antibody | Anti-aldolase antibody (Mouse monoclonal) | David J. Sullivan, Johns Hopkins Bloomberg School of Public Health | Immunoblot (1:25,000) | |
Antibody | Anti-ACP antibody (Rabbit polyclonal) | Gallagher and Prigge, 2010 https://doi.org/10.1002/prot.22582 | Immunofluorescence assays (1:500) | |
Antibody | Anti-mouse Alexa 488 (Goat polyclonal) | Thermo Fisher Scientific, MA, USA | Cat# A11029 | Immunofluorescence assays (1:1000) |
Antibody | Anti-rabbit Alexa 594 (Goat polyclonal) | Thermo Fisher Scientific, MA, USA | Cat# A11037 | Immunofluorescence assays (1:1000) |
Other | ProLong Gold 4′,6-diamidino-2-phenylindole (DAPI) antifade reagent | Thermo Fisher Scientific, MA, USA | Cat# P36935 | Mountant, Immunofluorescence |
Other | DAPI | Thermo Fisher Scientific, MA, USA | Cat# 62248 | DNA-specific stain, 1 µg/mL |
Other | MitoTracker CMX-Ros | Thermo Fisher Scientific, MA, USA | Cat# M7512 | Mitochondrion-specific stain, 30 nM |
Other | Bm MnmA | PiroplasmaDB (https://piroplasmadb.org) | BMR1_03g03155 | Babesia microti MnmA protein sequence |
Other | Ta MnmA | PiroplasmaDB (https://piroplasmadb.org) | TA12620 | Theileria annulata MnmA protein sequence |
Other | Tg MnmA | ToxoDB (https://toxodb.org) | TGME49_309110 | Toxoplasma gondii MnmA protein |
Other | Et MnmA | ToxoDB (https://toxodb.org) | ETH_00027940 | Eimeria tenella MnmA protein sequence |
Other | Bs MnmA | Uniprot (https://uniprot.org) | O35020 | Bacillus subtilis MnmA protein sequence |
Other | Bs YrvO | Uniprot (https://uniprot.org) | O34599 | B. subtilis YrvO protein sequence |
Other | Bs SufS | Uniprot (https://uniprot.org) | O32164 | B. subtilis SufS protein sequence |
Other | Ec SufE | Uniprot (https://uniprot.org) | P76194 | Escherichia coli SufE protein sequence |
Other | Ec MnmA | Uniprot (https://uniprot.org) | P25745 | E. coli MnmA protein sequence |
Other | Ec SufS | Uniprot (https://uniprot.org) | P77444 | E. coli SufS protein sequence |
Other | Ec IscS | Uniprot (https://uniprot.org) | P0A6B7 | E. coli IscS protein sequence |
Other | Sy MnmA | Uniprot (https://uniprot.org) | Q2JY34 | Synechococcus sp. MnmA protein sequence |
Other | At MnmA | Uniprot (https://uniprot.org) | Q9SYD2 | Arabidopsis thaliana MnmA protein sequence |
Other | At cNif1 | Uniprot (https://uniprot.org) | Q93WX6 | Protein sequence of A. thaliana Nif1 protein in the chloroplast |
Other | At mNif1 | Uniprot (https://uniprot.org) | O49543 | Protein sequence of A. thaliana Nif1 protein in the mitochondrion |
Other | Sc Mtu1 | Uniprot (https://uniprot.org) | Q12093 | Saccharomyces cerevisiae Mtu1 protein sequence |
Other | Sc Nfs1 | Uniprot (https://uniprot.org) | P25374 | S. cerevisiae Nfs1 protein sequence |
Additional files
-
Supplementary file 1
Supplementary information for reproducing and interpreting data presented in the manuscript.
(a) Sulfur-dependent pathways present in P. falciparum. (b) Summary of attempted transfections in P. falciparum to knockout genes of interest. (c) Plasmids used for generation of gene deletion lines. (d) Primers used in this study. Restriction enzyme sites are single underlined, linker regions are double underlined.
- https://cdn.elifesciences.org/articles/84491/elife-84491-supp1-v2.docx
-
MDAR checklist
- https://cdn.elifesciences.org/articles/84491/elife-84491-mdarchecklist1-v2.pdf