Long-timescale anti-directional rotation in Drosophila optomotor behavior
Figures
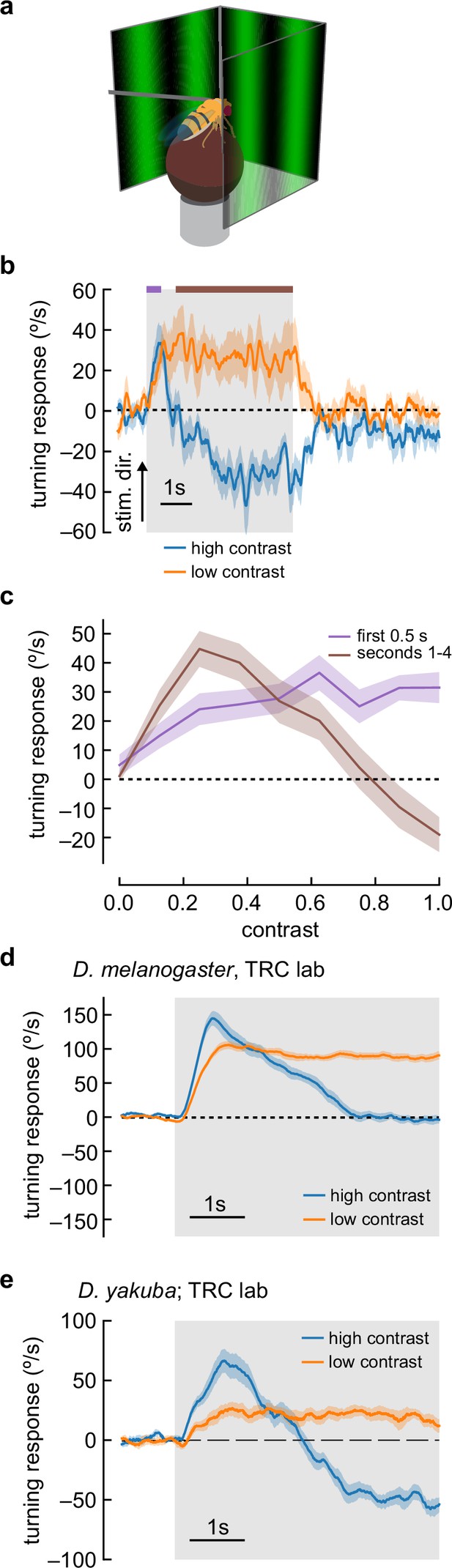
Flies turn opposite to the stimulus direction in high-contrast conditions.
(a) We measured fly turning behavior as they walked on an air-suspended ball. Stimuli were presented over 270° around the fly. (b) We presented drifting sinusoidal gratings for 5 s (shaded region) with either high contrast (c=1.0) or low contrast (c=0.25). When high-contrast sinusoidal gratings were presented, flies initially turned in the same direction as the stimulus, then started turning in the opposite direction after ~1 s of stimulation. Under low-contrast conditions, flies turned continuously in the same direction as the stimulus. In these experiments, the sine waves had a wavelength of 60° and a temporal frequency of 1 Hz. Shaded patches represent ±1 SEM. N=10 flies. (c) We swept contrast between 0 and 1 and measured the mean turning response during the first 0.5 s (purple, purple bar in b) and during the last 4 s of the stimulus (brown, brown line in b). The response in the first 0.5 s increased with increasing contrast, while the response in the last 4 s increased from c=0 to c=0.25, and then decreased with increasing contrast, until flies turned in the direction opposite the stimulus direction at the highest contrasts. N=20 flies. (d) We repeated the presentation of drifting sinusoidal gratings, this time in the lab of author TRC, using a similar behavioral apparatus. Stimulus parameters were as described in (b). In these experiments, the population average shows that flies proceeded to zero net turning at high contrasts, but some individual flies exhibited anti-directional turning responses. N=20 flies. (e) We repeated the experiments with D. yakuba, also in the lab of TRC, and observed that this species exhibited a robust anti-directional turning response to high-contrast gratings and a classical syn-directional turning response to low-contrast gratings. N=11 flies.
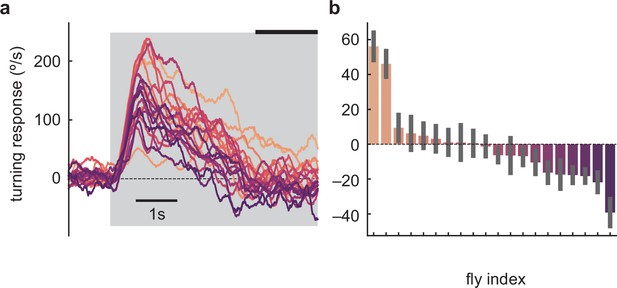
Individual D. melanogaster flies in TRC lab experiments show anti-directional turning.
(a) Mean time traces of individual fly responses to the high-contrast stimulus, averaged over trials. The flies are those in Figure 1d. (b) Long-timescale responses of individual flies, averaged over the last 1.5 s of the 5 s stimulus in panel (a) (indicated by thick black line). Mean and SEM shown are over the trials presented to that fly.
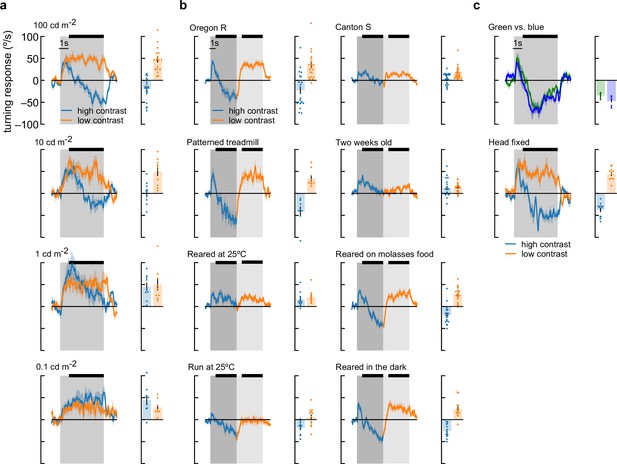
Flies perform anti-directional turning under a wide range of stimulus and growing conditions.
(a) Fly turning behavior at different mean screen brightness. We swept brightness from 100 cd/m2 to 0.1 cd/m2 and measured turning responses to high- and low-contrast stimuli. Flies performed the most anti-directional behavior in response to high-brightness stimuli. At 1 cd/m2, flies never turned in the opposite direction of the stimulus, and at 0.1 cd/m2, flies turned continuously in the same direction as the stimulus, even in high-contrast conditions. We also measured average turning during the last 4 s of stimulation (black bar above time traces). Average fly behavior shown as bars on the right, with individual fly behavior shown as dots. Shaded patches in the time trace plots represent ±1 SEM, as do vertical lines on bar plots. N=19, 10, 9, 8 flies, top to bottom. (b) Our wildtype flies were Oregon-R strain (Gohl et al., 2011) raised at 20°. They were grown on glucose-based food media with 12 hr light-dark cycles. Experiments were run at high temperature, 12–60 hr after eclosion. We used uniform, red balls to avoid visual feedback from walking. The response of these wildtype flies to a contrast-switching stimulus (as in Figure 2c) is shown in the upper left corner. We also tested different variations of all these parameters. Canton-S flies turned less overall, and showed less anti-directional turning, but still turned in the opposite direction after 5 s of high-contrast stimuli. We tested flies walking on highly visible silver balls with black dots and saw behavior similar to wildtype. Two-week-old flies showed reduced turning and much reduced anti-directional behavior. Flies raised at 25°C had behavior similar to 2-week-old flies. When we performed experiments at 25°C, we saw much less optomotor turning, but anti-directional turning persisted. Rearing on molasses-based media or in the dark did not have strong effects on behavior. N=22, 8, 12, 12, 24, 19, 19, 13 flies top to bottom, left to right. (c) Other changes to the experimental setup did not cause large differences in behavior. We compared responses to high-contrast stimuli presented with green light (peak wavelength: 525 nm) and blue light (peak wavelength: 450), and did not see large differences in behavior. Head-fixed flies (middle) showed similar behavior to head-free flies (a, top). N=5 and 11 flies, top to bottom.
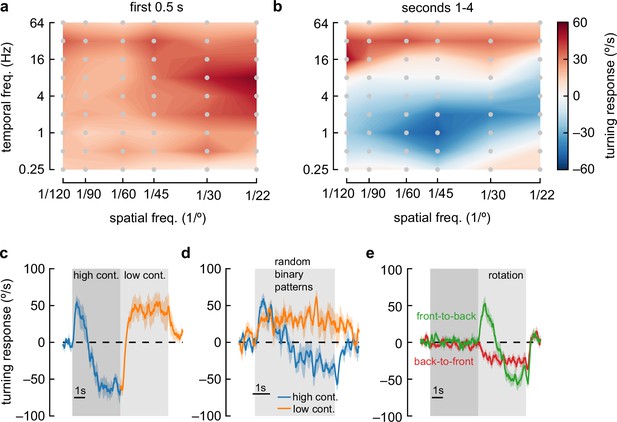
Anti-directional turning behavior has distinct tuning and is driven by adaptation.
(a) Heatmap of fly turning velocity during the first 0.5 s of sinusoidal grating stimulation under high-contrast conditions and variable temporal and spatial frequencies. The flies turned in the direction of the stimulus across all conditions and responded most to 8 Hz, 22° stimuli. N=16, 21, 17, 21, 7, and 22 flies for spatial frequencies 1/120°, 1/90°, 1/60°, 1/45°, 1/30°, and 1/22° respectively. (b) Heatmap as in (a), measured during the last 4 s of stimulation. Flies turned in the same direction as the stimulus at high and low temporal frequencies, but in the opposite direction of the stimulus at intermediate temporal frequencies, with a maximal anti-directional response at wavelengths between 30° and 60°. (c) Switching stimulus contrast from high to low after 5 s caused flies to revert to syn-directional behavior after the anti-directional response. N=7 flies. (d) Presenting rotating random binary patterns (5° vertical strips rotating at 150 °/s) induced anti-directional turning similar to that elicited by rotating sine wave gratings. N=7 flies. (e) We presented flies with 5 s of ‘translational’ stimuli (dark shaded region), with high-contrast sinusoidal gratings moving either front-to-back or back-to-front, bilaterally, for 5 s. After that, we presented high-contrast rotational sinusoidal grating stimuli (60° wavelength, 1 Hz). Front-to-back stimulation did not affect the subsequent response to rotational stimuli, but back-to-front stimuli caused flies to turn immediately in the opposite direction of the stimulus. N=18 flies.
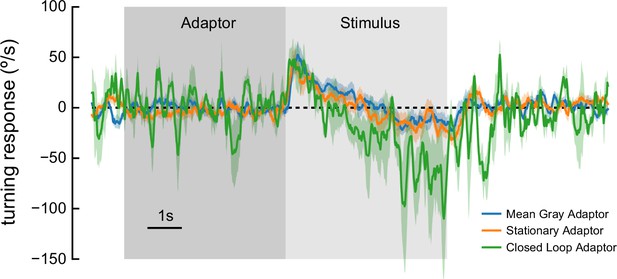
As in Figure 2e, with different adapters.
We presented flies with 5 s of adapting stimuli (dark shaded region) for 5 s, with adapting stimuli consisting of (1) mean gray luminance; (2) a stationary, 60° wavelength contrast 1 sinusoid; or (3) a closed-loop, 60° wavelength contrast 1 sinusoid, which rotated in response to the behavior of the fly, for instance rotating 90° clockwise when the ball rotated 90° clockwise. The closed-loop lag time was approximately 66 ms. After the adapter, we presented high-contrast rotational sinusoidal grating stimuli (60° wavelength, 1 Hz). The closed-loop stimulus yields noisier responses because each fly controlled the stimulus for only about 1/5 of the trials. The qualitative behavior in all cases was comparable to the anti-directional turning observed elsewhere. N=10 flies.
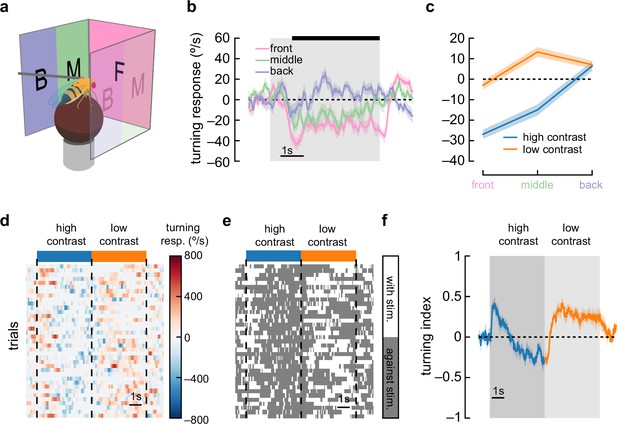
Anti-directional turning is driven by stimuli in the forward-facing visual field and is not driven by saccades.
(a) We divided our panoramic display into three sections: the front 90°, the 45° behind the fly on either side, and a middle 45°. (b) High-contrast sinusoidal gratings were presented on each of these three display sections, with the remaining sections blank. Flies turned syn-directionally when stimuli were presented behind the fly, and turned anti-directionally when stimuli were presented in front of the fly. Shaded patches represent ±1 SEM. N=55 flies. (c) Average turning in the last 4 s of the stimulus (black bar in b), in low-contrast and high-contrast conditions. Shaded patches in the time trace plots represent ±1 SEM. N=55 flies. (d) A single fly responds to many trials of sinusoidal grating stimuli at high contrast (blue bar) and low contrast (orange bar). We show a heatmap of the fly’s responses over time (horizontal axis) and across trials (vertical axis). (e) We can ignore the magnitude of the turning and instead only quantify whether the fly was turning in the same direction as the stimulus (white area) or in the opposite direction (dark gray area). This shows sustained anti-directional turning, not brief saccades. (f) Averaging the direction (but not magnitude) of turning across trials and across flies yields a turning index for each point in time. Shaded patches in the time trace plots represent ±1 SEM. N=7 flies.
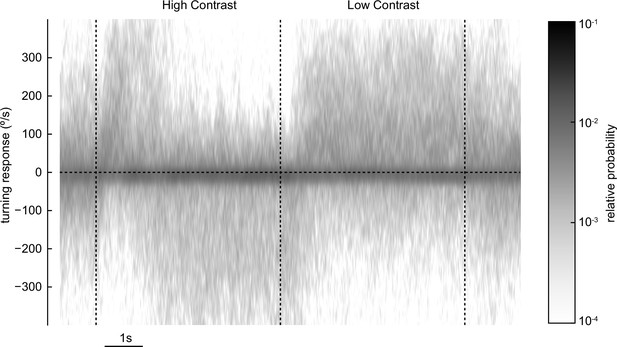
Distribution of turning responses over trials and over flies.
These are the traces from Figure 3d–f, in which responses were measured to high-contrast followed by low-contrast sinusoids. Here, we aggregated all trials and over all flies, and plotted the distribution of rotational velocities at each timepoint (60 Hz). The most common velocities were near 0, but the full distribution clearly shifts negatively after ~2 s of the high-contrast stimulus, before shifting back positive for the low-contrast stimulus. This full distribution suggests that mean rotational velocities are a reasonable summary statistic for the distribution of turning velocities.
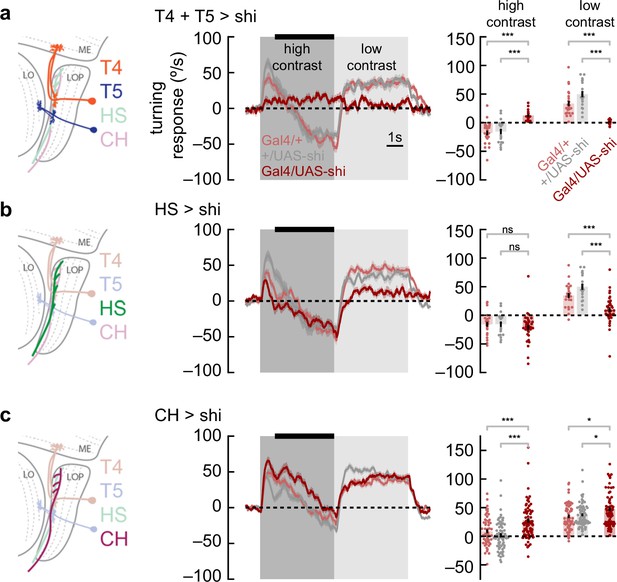
Syn-directional and anti-directional turning share common circuitry.
(a) We silenced T4 and T5 neurons by expressing shibirets selectively in those neurons. We measured turning behavior during a contrast-switching stimulus (as in Figure 2c). Results from flies with T4 and T5 silenced shown in dark red, while controls are in light red and gray. Average fly behavior during the last 4 s of the first contrast (black bar on left) shown as bars on the right, with individual fly behavior shown as dots. Note that the data labeled ‘low contrast’ are from experiments in which the low-contrast stimulus was shown before the high-contrast stimulus. Shaded patches in the time trace plots represent ±1 SEM, as do vertical lines on bar plots. *** indicates experimental results are significantly different from results, p<0.001 via a two-sample Student’s t-test. * indicates p<0.05. N=17, 24, 19 flies with genotypes T4T5/Shibirets, T4T5/+, +/Shibirets. (b) Results from horizontal system (HS) neuron silencing as in (a). Silencing HS neurons reduced syn-directional turning behavior (p<0.001) but did not have a strong effect on anti-directional turning. N=34, 21, 19 flies with genotypes HS/Shibirets, HS/+, +/Shibirets. (c) Results from CH neuron silencing as in (a). CH neuron silencing reduced the degree of anti-directional turning (p<0.001). N=63, 57, 70 flies with genotypes CH/Shibirets, CH/+, +/Shibirets.
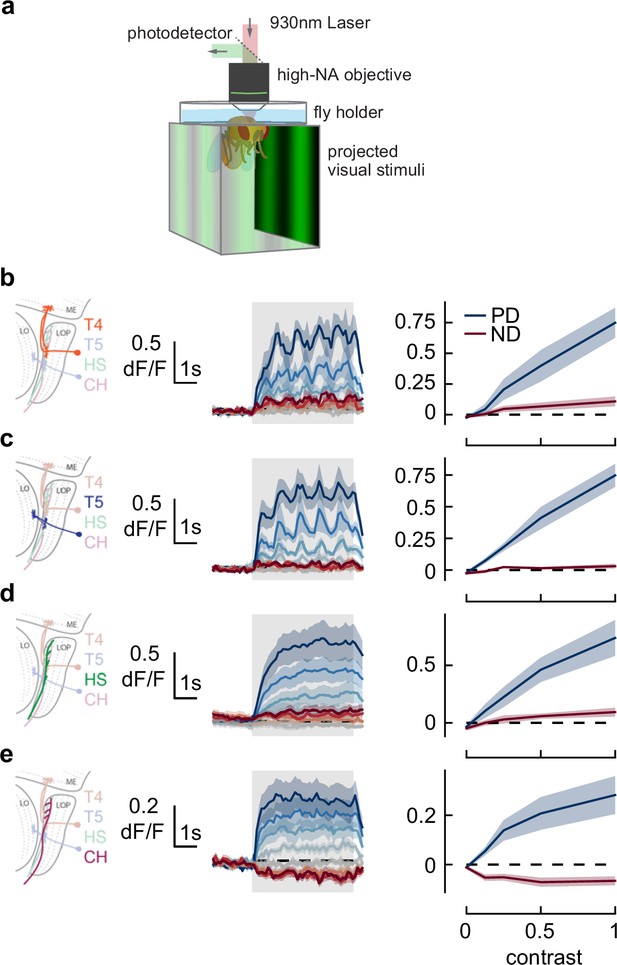
Responses in early direction-selective cells do not show a reduction or reversal of response on the timescale of the behavior.
(a) We used two-photon microscopy to measure calcium activity in lobula plate neurons while presenting sinusoidal gratings at a range of contrasts. (b) T4 cells, marked in orange (left), responded to drifting sinusoidal gratings with increased calcium activity (middle). Darker colors indicate higher contrast, preferred direction in blue, null direction in red. When integrated across the stimulus presentation (right), calcium activity increased with stimulus contrast. N=8 flies. (c–e) As in (b) measuring calcium activity in T5, horizontal system (HS), and CH neurons. N=8, 10, 15 flies.

Anti-directional turning behavior occurs when using the optical filters also employed in the two-photon imaging experiments.
High- and low-contrast sinusoidal stimuli were presented as in Figure 2c, but using the bandpass filters also used in our two-photon microscope stimulus presentation. N=30 flies.
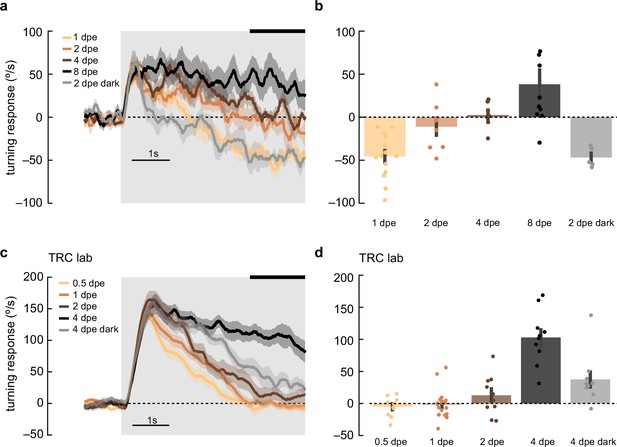
Maturation of optomotor response in early adulthood.
(a) Adult flies at various ages post eclosion were presented with 5 s, high-contrast, rotating sinusoidal gratings as in Figure 2b. As the flies aged from 1 day post eclosion (dpe) to 2, 4, and 8 dpe, the initial anti-directional turning response transitioned into syn-directional turning. Dark-rearing flies at 2 dpe reduced this maturation effect. Shaded patches represent ±1 SEM. N=5–14 flies. (b) The last 1.5 s of the mean turning velocity of each fly was averaged, and the population response was plotted. (c) As in (a) but in the TRC lab, using 0.5, 1, 2, and 4 dpe, with dark rearing for 4 dpe. With maturation, the syn-directional turning became less transient. N=9–15 flies. (d) As in (b) but for data in (c).
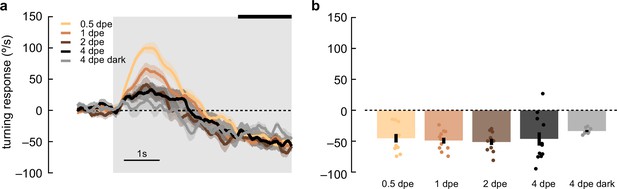
D. yakuba lacks plasticity of anti-directional responses in adulthood that is observed D. melanogaster.
(a) Adult yakuba flies at various ages post eclosion were presented with 5 s, high-contrast, rotating sinusoidal gratings as in Figure 6. Data was acquired in the TRC lab. Anti-directional responses stayed consistent from 0.5 days post eclosion (dpe) to 1, 2, and 4 dpe, although the initial optomotor response became smaller as the flies aged. Shaded patches represent ±1 SEM. N=7–11 flies. (b) The last 1.5 s of the mean turning velocity of each fly was averaged, and the population response was plotted.
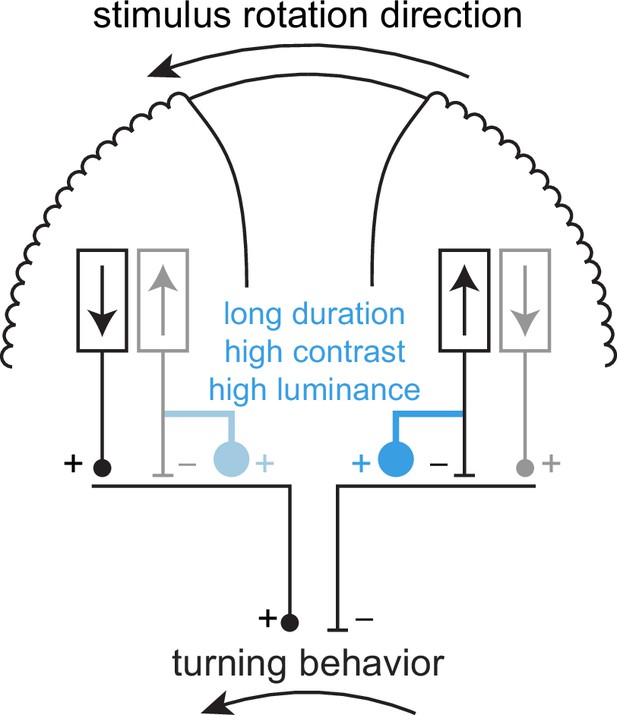
Schematic of potential subtractive circuit properties consistent with data presented in this study.
Schematic top view of the head of a fly, showing one eye on each side. A counterclockwise stimulus would tend to drive counterclockwise turning behavior on short timescales. In this schematic, this is driven by front-to-back motion detectors on the left eye and back-to-front motion detectors on the right eye (in black, as opposed to gray detectors, which are not active). The influences from the two eyes sum to contribute to counterclockwise turning. One putative anti-directional turning mechanism is shown in blue: under high-contrast and high-luminance conditions, on long timescales, the regressive motion detectors on the right eye influence turning with the opposite sign, with an influence stronger than the progressive motion detectors on the left eye. Our imaging data show that this reversal is not occurring in T4, T5, horizontal system (HS), or CH neurons (Figure 5), so must be somewhere parallel to or downstream of those neurons.
Tables
Parental stock genotypes.
Name | Genotype | Source | Stock # |
---|---|---|---|
Wildtype | +; +; + (IsoD1) | Gohl et al., 2011 | N/A |
T4T5-Gal4 | +; +; R42F06-Gal4 (IsoD1 background) | BDSC | BDSC 41253 |
HS-Gal4 | +; +; R27B03-Gal4 (IsoD1 bg) | Seelig et al., 2010 | BDSC 49211 |
CH-Gal4 | w; +; R35A10-Gal4 (Janelia bg) | BDSC | BDSC 49897 |
UAS-Shibirets | +; +; UAS-Shibirets (IsoD1 bg) | Silies et al., 2013 | N/A |
Empty Gal4 | w; +; pBDPGAL4.1Uw (Janelia bg) | BDSC | BDSC 68384 |
GCaMP6f | w; UAS-GCaMP6f; + | BDSC | BDSC 42747 |
jGCaMP7b | w; +; UAS-jGCaMP7b | BDSC | BDSC 79029 |
mtdTomato | w; +; UAS-mtdTomato | BDSC | BDSC 30124 |
Genotypes of flies used in behavior experiments.
Experimental | Gal4 control | UAS control | Background control |
---|---|---|---|
T4T5-Gal4 x UAS- Shibirets: +; +; R42F06-Gal4/UAS-Shibirets | T4T5-Gal4 x IsoD1: +;+;R42F06-Gal4/+ | IsoD1 x UAS-Shibirets: +; +; +/UAS-Shibirets | IsoD1: +; +; + |
HS-Gal4 x UAS- Shibirets: +; +; R27B03-Gal4/UAS-Shibirets | HS-Gal4 x IsoD1: +; +; R27B03-Gal4/+ | IsoD1 x UAS-Shibirets: +; +; +/UAS-Shibirets | IsoD1: +; +; + |
CH-Gal4 x UAS-Shibirets: w/+; +; R35A10-Gal4/UAS-Shibirets | CH-Gal4 x IsoD1: w/+; +; R35A10-Gal4/+ | Empty Gal4 x UAS-Shibirets: +/w; +; pBDPGAL4.1Uw /UAS-Shibirets | Empty Gal4 X IsoD1: +/w; +; +/ pBDPGAL4.1Uw |