Neuropeptide Y-expressing dorsal horn inhibitory interneurons gate spinal pain and itch signalling
Figures
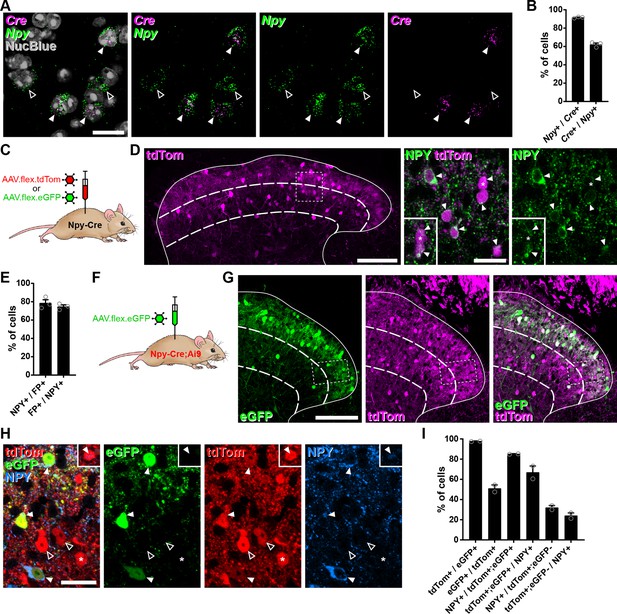
Cre-dependent adeno-associated virus (AAV) injections in young adult Npy-Cre mice target dorsal horn inhibitory neuropeptide Y (NPY) interneurons and avoid transient NPY-expressing cells.
(A) In situ hybridisation for Cre (magenta) and Npy (green) mRNA in the mid-lumbar dorsal horn. Sections were counterstained with NucBlue (grey) to reveal nuclei. Three Cre-positive cells are also positive for Npy (filled arrowheads), and there are two cells that are positive for Npy only (open arrowheads). Scale bar = 20 µm. (B) Quantification of co-expression of Cre and Npy mRNA in laminae I–III (n = 3 mice). (C) The experimental approach used to generate the data presented in (D, E). (D) Co-expression of tdTomato (tdTom; magenta) and NPY (green) immunoreactivity in mid-lumbar dorsal horn of an Npy-Cre mouse injected with AAV.flex.tdTom in adulthood. Low power image shows tdTomato-positive cells throughout laminae I–III (scale bar = 100 µm). High power images (corresponding to the box in the low power image) demonstrate the high degree of co-localisation of tdTomato expression and NPY immunoreactivity (filled arrowheads; scale bar = 20 µm). Insets show clearer NPY labelling in a different z-plane for the cell marked with an asterisk and the cell immediately below it. (E) Quantification of co-expression of fluorescent protein (FP) and NPY in laminae I–III of Npy-Cre mice injected with AAV.flex.tdTom or AAV.flex.eGFP at adulthood (n = 3 mice; 2 injected with AAV.flex.tdTomato and 1 with AAV.flex.eGFP). (F) The experimental approach taken for the data presented in (G–I). (G) Expression of tdTomato and eGFP in mid-lumbar dorsal horn of an Npy-Cre;Ai9 mouse injected with AAV.flex.eGFP in adulthood. eGFP-positive cells (green) are a subset of a broader population of tdTomato-positive cells (magenta; scale bar = 100 µm). (H) High power images corresponding to boxed area in (G) showing three tdTom+/eGFP+ (red and green, respectively) double-labelled cells (filled arrowheads) that are also NPY immunoreactive (blue). Open arrowheads mark two cells positive for tdTomato only. The asterisk marks a tdTomato-positive/eGFP-negative cell that is also NPY immunoreactive, though this is only apparent in a different z-plane (insets). Scale bar = 20 µm. (I) Quantification of co-expression of tdTomato, eGFP, and NPY in laminae I–III of Npy-Cre;Ai9 mice injected with AAV.flex.eGFP at adulthood (n = 2 mice). Solid lines in low power images in (D, G) denote the grey/white matter border, curved dashed lines denote the boundaries of lamina III and dashed boxes denote the regions shown in corresponding high power images. Data are shown as individual values with mean ± standard error of the mean (SEM).
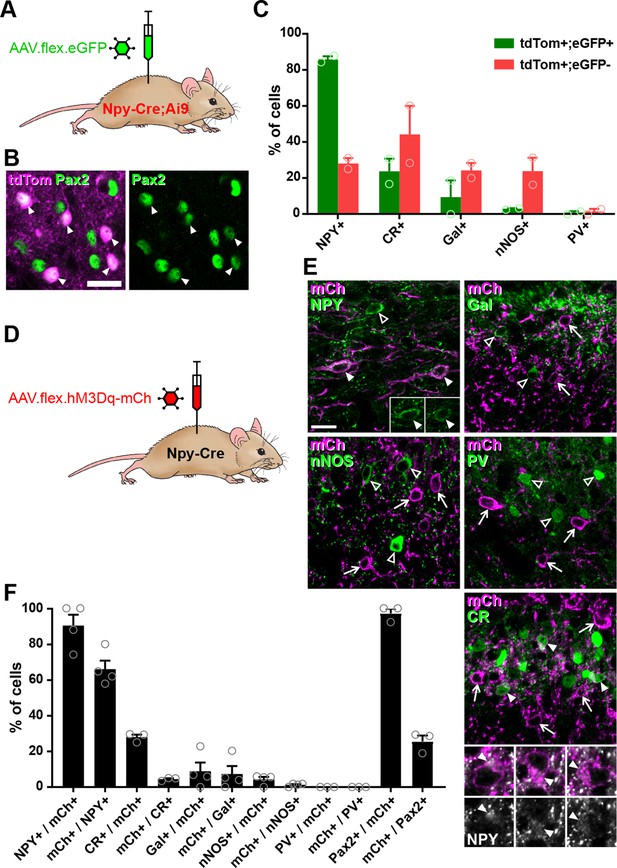
Cre-dependent adeno-associated virus (AAV) injections in young adult Npy-Cre mice target dorsal horn inhibitory neuropeptide Y (NPY) interneurons and avoid transient NPY-expressing cells.
(A) The experimental approach used to generate the data shown in (B, C). (B) Co-expression of tdTomato (tdTom; magenta) and Pax2 (green) in the mid-lumbar dorsal horn of an adult Npy-Cre;Ai9 mouse. All tdTomato-positive cells are also Pax2 immunoreactive (filled arrowheads; scale bar = 20 µm). (C) Quantification of the proportion of tdTom+/eGFP+ double-labelled cells and tdTomato-positive, eGFP-negative cells (tdTom+/eGFP−) that express various inhibitory interneuron neurochemical markers in laminae I and II of Npy-Cre;Ai9 mice that were injected with AAV.flex.eGFP in adulthood (n = 2 mice). (D) The experimental approach used to generate the data shown in (E, F). (E) Micrographs demonstrating the distribution of hM3Dq-mCherry expression (mCh, magenta) and immunoreactivity for various inhibitory interneuron neurochemical markers (green) in mid-lumbar dorsal horn of an Npy-Cre mouse injected with AAV.flex.hM3Dq-mCherry in adulthood (Gal, galanin; nNOS, neuronal nitric oxide synthase; PV, parvalbumin; CR, calretinin). Arrows mark cells labelled with mCherry only, open arrowheads mark cells positive for the neurochemical marker only and filled arrowheads mark cells that express both hM3Dq-mCherry and the neurochemical marker. Insets in the NPY image show NPY staining only for the cells marked with filled arrowheads to demonstrate the NPY immunoreactivity in these cells. Higher magnification images below the CR image show NPY immunoreactivity (grey) in the cells marked with filled arrowheads. Scale bar for all main images = 20 µm. (F) Quantification of co-expression of hM3Dq-mCherry and the various inhibitory interneuron neurochemical markers in laminae I and II of Npy-Cre mice injected with AAV.flex.hM3Dq-mCherry in adulthood (n = 3 mice). Data are shown as individual values with mean ± standard error of the mean (SEM).
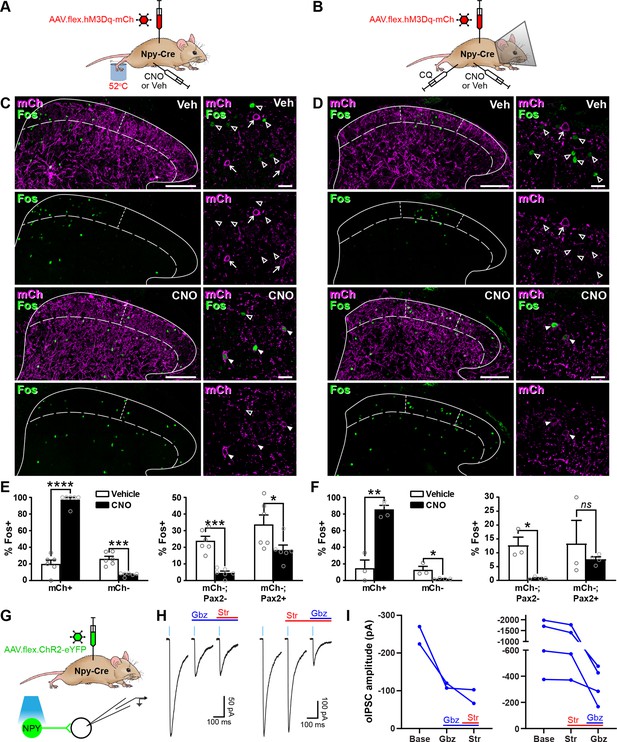
Activation of inhibitory neuropeptide Y (NPY) interneurons reduces activity in dorsal horn circuits recruited by noxious and pruritic stimuli.
(A, B) The experimental approaches used to generate the data presented in (C, E) and (D, F), respectively. (C, D) Low power images show mCherry expression (mCh; magenta) and Fos immunoreactivity (green) in L4 (C) or L3 (D) dorsal horn of Npy-Cre mice that had been injected with AAV.flex.hM3Dq-mCherry and treated with vehicle control (Veh) or clozapine-N-oxide (CNO) 30 min prior to a noxious heat stimulus (immersion of the hindpaw in 52°C water; C) or a pruritic stimulus (intradermal injection of 100 μg chloroquine, CQ, dissolved in 10 μl phosphate-buffered saline (PBS) into the calf; D) and perfusion-fixed 2-hr post-stimulation. In vehicle-treated animals, Fos expression is observed in the somatotopically relevant area of the superficial laminae (left of short dashed line in C; between short dashed lines in D). In CNO-treated animals, Fos expression is observed in hM3Dq-mCherry-expressing cells, but is reduced in surrounding hM3Dq-mCherry-negative cells within the somatotopically relevant area. This is demonstrated in the high magnification images (to the right of the main image in each case), where filled arrowheads mark examples of hM3Dq-mCherry-expressing cells immunoreactive for Fos, open arrowheads mark Fos-positive cells that lack hM3Dq-mCherry and arrows mark hM3Dq-mCherry-expressing cells that are negative for Fos. Scale bars = 100 µm (low power images), 20 µm (high power images). Solid lines in (C and D) denote the grey/white matter border, curved dashed lines denote the lamina II/III border. (E, F) Left-hand graphs show quantification of the proportion of hM3Dq-mCherry-positive (mCh+) and -negative (mCh−) cells that display Fos immunoreactivity in vehicle- or CNO-treated mice that received a noxious heat (E; n = 3 mice for vehicle, 4 for CNO) or pruritic (F; n = 6 for both groups) stimulus. Right-hand graphs in (E, F) show quantification of the proportion of hM3Dq-mCherry-negative cells that display Fos immunoreactivity, separated into excitatory (Pax2−) and inhibitory (Pax2+) populations. Analyses were performed within the somatotopically relevant areas of laminae I and II. (G) The experimental approach used to generate the data presented in (H, I). (H) Representative optogenetically evoked IPSCs (oIPSCs) recorded in unlabelled (ChR2-eYFP-negative) cells in spinal cord slices from Npy-Cre mice that had received intraspinal injections of AAV.flex.ChR2-YFP. Recordings were made in the absence and presence of gabazine (Gbz) and strychnine (Str). Traces show an average of six stimuli, light blue bars denote period of optogenetic stimulation. Note that in the presence of high intracellular Cl− concentration, IPSCs appear as inward currents. (I) Quantification of the mean peak amplitude of oIPSCs recorded in the absence (Base) and presence of gabazine and strychnine. Two cells were initially tested with gabazine and then strychnine, while four cells were initially tested with strychnine and then gabazine. Data are shown as mean ± standard error of the mean (SEM). *p < 0.05, **p < 0.01, ***p < 0.001, ****p < 0.0001; unpaired t-test with Holm–Šidák correction for multiple comparisons. Data are shown as individual values with mean ± SEM in (E, F) and individual values in (I).

Clozapine-N-oxide (CNO)-mediated activation of dorsal horn inhibitory neuropeptide Y (NPY) interneurons in Npy-Cre mice injected with AAV.flex.hM3Dq-mCherry.
(A) The experimental approach used to generate the data shown in this figure. (B) Sagittal sections through the ipsilateral side of the L3–5 spinal cord segments of Npy-Cre mice injected with AAV.flex.hM3Dq-mCherry at L3, L4, and L5 levels and treated with vehicle control (Veh) or CNO 2 hr prior to perfusion fixation. hM3Dq-mCherry expression (mCh, magenta) can be seen extending through the L3–5 dorsal horn in both animals. Fos-positive cells (green) are infrequent in the vehicle-treated animal, but are numerous in the CNO-treated mouse and correspond to the area of hM3Dq-mCh expression. Dashed boxes indicate the areas shown at higher magnification in the insets. Scale bar for all main images = 500 µm. (C) Top graph shows quantification of the proportion of hM3Dq-mCherry-positive (mCh+) and -negative (mCh−) cells in laminae I–III that display Fos immunoreactivity in vehicle- or CNO-treated mice. Bottom graph shows quantification of the proportion of hM3Dq-mCherry-negative cells in laminae I–III that display Fos immunoreactivity in vehicle- or CNO-treated mice, separated into excitatory (Pax2−) and inhibitory (Pax2+) populations. *p < 0.05, ***p < 0.001, ****p < 0.0001; unpaired t-test with Holm–Šidák correction for multiple comparisons; n = 4. (D) Example confocal image demonstrating co-expression of hM3Dq-mCh (red), Fos (green), and NPY (blue) in L4 dorsal horn of an Npy-Cre mouse that had been injected with AAV.flex.hM3Dq-mCherry and treated with CNO 2 hr prior to perfusion fixation. Three hM3Dq-mCherry-positive cells that are immunoreactive for both Fos and NPY are highlighted (filled arrowheads). A weakly NPY-positive cell that lacks both hM3Dq-mCherry and Fos expression can also be seen (open arrowhead). Scale bar = 20 µm. (E) Quantification of co-expression of hM3Dq-mCherry, Fos, and NPY in laminae I–III in the L4 segment of mice treated with CNO 2 hr prior to perfusion fixation (n = 4). Data are shown as individual values with mean ± standard error of the mean (SEM).

Characterisation of optogenetic activation of NPY-INs.
(A) The experimental approach used to generate data presented in (B, C). Representative traces showing optogenetically evoked action potential firing (B) and currents (C) in an NPY-IN labelled with ChR2-eYFP. Each trace displays six stimuli. (D) The experimental approach used to generate data presented in (E, F). (E) Representative traces ofoptogenetically evoked IPSCs (oIPSCs) recorded in unlabelled (ChR2-eYFP-negative) cells in the absence (Baseline) and presence of NBQX and D-APV, six individual oIPSCs are shown in grey and an averaged trace in black. (F) Quantification of the mean peak amplitude of oIPSCs recorded in the absence and presence of NBQX and D-APV (n = 7 cells). Blue boxes in (B, C, E) denote the period of optogenetic stimulation. Data are shown as individual matched values with mean ± standard error of the mean (SEM).
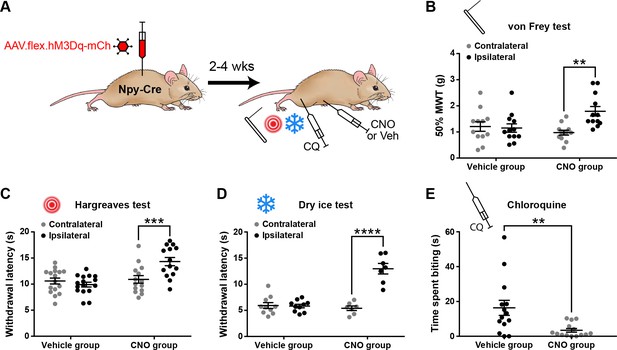
Activation of inhibitory neuropeptide Y (NPY) interneurons increases acute nociceptive thresholds and reduces pruritogen-evoked itch behaviour.
(A) The experimental approach used to generate the data presented in (B–E). (B–D) AAV.flex.hM3Dq-mCherry spinal-injected Npy-Cre mice display an increased mechanical withdrawal threshold (MWT) (B; n = 12 vehicle group, 12 clozapine-N-oxide [CNO] group) and increased withdrawal latencies to radiant heat (C; n = 16 vehicle group, 14 CNO group) and to cold (D; n = 10 vehicle group, 7 CNO group) of the ipsilateral hindpaw following CNO injection, but not vehicle control injection. (E) AAV.flex.hM3Dq-mCherry spinal-injected mice spend significantly less time biting the calf region in the 30 min following intradermal injection of chloroquine when injected with CNO, compared to vehicle-treated controls (n = 14 vehicle group, 14 CNO group). Data are shown as individual values with mean ± standard error of the mean (SEM). **p < 0.01, ***p <0.001, ****p < 0.0001; repeated-measures two-way ANOVA with Šidák’s post-test in (B–D); unpaired t-test in (E).

Injection of 5 mg/kg clozapine-N-oxide (CNO) does not result in off-target behavioural effects.
(A) The experimental approach used to generate data presented in this figure. Eight naive wild-type C57BL/6 mice were injected with 5 mg/kg CNO or vehicle control using a cross-over experimental design and tested for mechanical withdrawal thresholds (MWT; B), as well as withdrawal latencies to heat (C) and cold (D) on both hindpaws. General motor co-ordination was assessed using the accelerating rotarod test (E). No significant differences were seen between treatments for either paw in any of the nociceptive tests (repeated-measures two-way ANOVA with Šidák’s post-test), or in the maximum RPM in the accelerating rotarod test (paired t-test). Data are shown as individual values with mean ± standard error of the mean (SEM).
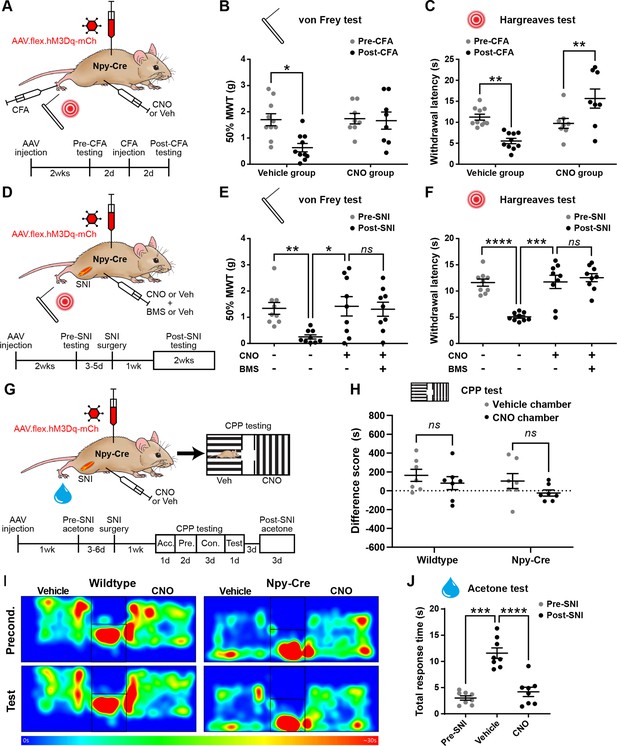
Activation of inhibitory neuropeptide Y (NPY) interneurons blocks mechanical and thermal hypersensitivity in models of inflammatory and neuropathic pain.
(A) The experimental approach taken to generate the data presented in (B, C). (B, C) Vehicle control-treated AAV.flex.hM3Dq-mCherry spinal-injected Npy-Cre mice display marked reductions in mechanical withdrawal threshold (MWT) (B) and withdrawal latency to radiant heat (C) of the ipsilateral paw 2 days after intraplantar injection of complete Freund’s adjuvant (CFA). Both mechanical and thermal hypersensitivity are blocked in clozapine-N-oxide (CNO)-treated mice (n = 10 vehicle group, 8 CNO group). (D) The experimental approach taken for the data presented in (E, F). Drug treatments were administered using a crossover design (n = 9). (E, F) Marked reductions in MWT (E) and withdrawal latency to radiant heat (F) are observed following spared nerve injury (SNI). These are blocked by CNO treatment, and this blockade persists in the presence of the Y1 antagonist BMS 193885 (BMS). (G) The experimental approach taken for the data presented in (H–J). Acc. = acclimation; Pre. = pre-conditioning; Con. = conditioning. (H) Neither wild-type control nor AAV.flex.hM3Dq-mCherry spinal-injected Npy-Cre mice displayed a conditioned place preference (CPP) to CNO following SNI (n = 7). (I) Heat maps of a representative mouse from each group demonstrating position and time spent in each chamber during preconditioning and post-conditioning test days. (J) A marked increase in the time spent responding to application of acetone to the ipsilateral paw (shaking, lifting, and/or licking) is seen in vehicle-treated AAV.flex.hM3Dq-mCherry spinal-injected Npy-Cre mice following SNI. This cold hypersensitivity is blocked when the same mice are treated with CNO (n = 8). Data are shown as individual values with mean ± standard error of the mean (SEM). *p < 0.05, **p < 0.01, ***p < 0.001, ****p < 0.0001; repeated-measures two-way ANOVA with Šidák’s post-test in (B, C, H), repeated-measures one-way ANOVA with Šidák’s post-test in (E, F, J).

Spared nerve injury (SNI) results in minimal ipsilateral hM3Dq expression in L4/5 DRG of AAV.flex.hM3Dq-mCherry spinal-injected Npy-Cre mice.
Maximum-intensity projections of confocal image stacks from contralateral and ipsilateral (with regard to adeno-associated virus (AAV) injection and SNI) L4 and L5 whole-mount dorsal root ganglia (DRG) from an AAV.flex.hM3Dq-mCherry spinal-injected Npy-Cre mouse perfused 4 weeks following SNI surgery. As expected, no hM3Dq-mCherry-positive cells were detected in the contralateral DRGs. On the ipsilateral side, a small number of hM3Dq-mCherry-positive cells were observed in both L4 and L5 DRGs (filled arrowheads mark examples). Scale bar for all images = 200 µm. The table shows quantification of mCherry-labelled cells on the contralateral and ipsilateral sides (n = 4; values are mean ± standard error of the mean [SEM; range]).

Gabapentin administration, but not chemogenetic activation of NPY-INs, induces conditioned place preference (CPP) following spared nerve injury (SNI).
(A) The experimental approach taken to generate the data presented in (B). (B) Neither wild-type control nor AAV.flex.hM3Dq-mCherry spinal-injected Npy-Cre mice displayed a CPP to i.p. clozapine-N-oxide (CNO) following SNI (n = 7; RM two-way ANOVA). Data per individual mouse, also presented in Figure 4H. (C) The experimental approach taken to generate the data presented in (D). (D) Wild-type mice display CPP to i.p. gabapentin administration following SNI (n = 6; *p = 0.016; paired t-test). Acc. = acclimation; Pre. = pre-conditioning; Con. = conditioning. Data are shown as individual matched values in (B) and individual values with mean ± standard error of the mean (SEM) in (D).
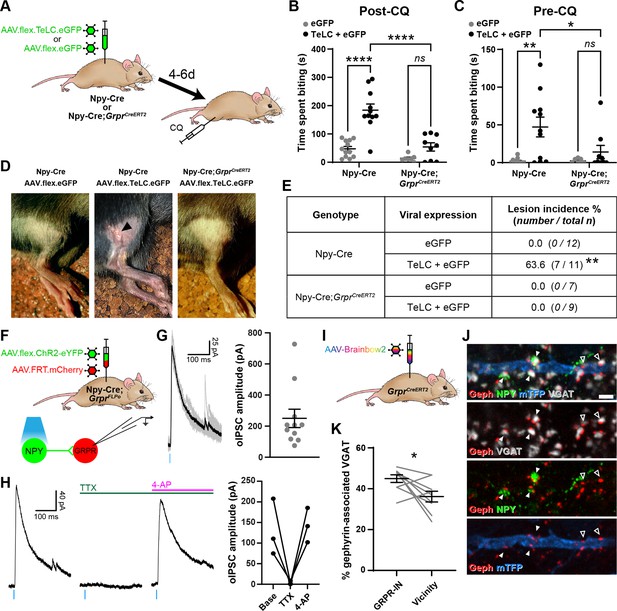
Increased itch caused by silencing neuropeptide Y (NPY) interneurons operates through a circuit involving GRPR-expressing excitatory interneurons.
(A) The experimental approach used to generate the data presented in (B–E). (B) Silencing of NPY-INs by viral expression of tetanus toxin light chain (TeLC) in AAV.flex.TeLC.eGFP spinal-injected Npy-Cre mice results in a significant enhancement of chloroquine-evoked itch (Post-CQ), compared to that seen in AAV.flex.eGFP-injected controls. This enhancement of CQ-evoked itch is significantly reduced when NPY- and GRPR-INs are simultaneously silenced by injecting AAV.flex.TeLC.eGFP into Npy-Cre;GrprCreERT2 mice. The numbers of mice per group are as outlined in the table in (E). (C) Silencing of NPY-INs by TeLC also results in the development of spontaneous itch behaviour as assessed over 30 min prior to CQ administration (Pre-CQ). This spontaneous itch is also significantly reduced when GRPR-INs are simultaneously silenced. (D) Representative images of a skin lesion on the calf of an AAV.flex.TeLC.eGFP-injected Npy-Cre mouse (arrowhead, middle image), and the lack of lesions in AAV.flex.eGFP-injected Npy-Cre or AAV.flex.TeLC.eGFP-injected Npy-Cre;GrprCreERT2 mice. (E) Table outlining the incidence of lesions in AAV.flex.eGFP- or AAV.flex.TeLC.eGFP-injected Npy-Cre or Npy-Cre;GrprCreERT2 mice. Lesions were observed in approximately two-thirds of AAV.flex.TeLC.eGFP-injected Npy-Cre mice, but never in AAV.flex.TeLC.eGFP-injected Npy-Cre;GrprCreERT2 mice, nor in AAV.flex.eGFP-injected control groups. (F) The experimental approach used to generate the data presented in (G, H). (G) Optogenetic activation of NPY-INs induces monosynaptic optogenetically evoked IPSCs (oIPSCs) in GRPR-INs. Representative traces of oIPSCs recorded in a GRPR neuron are shown on the left, with six individual oIPSCs in grey and an averaged trace in black. Quantification of the mean peak amplitude of oIPSCs recorded in 11 GRPR-INs is shown on the right. For all 11 cases, all 6 light stimuli resulted in oIPSCs with no failures. (H) Example traces from a GRPR-IN (left) show that oIPSCs are blocked by tetrodotoxin (TTX) and reinstated by 4-aminopyridine (4-AP); quantification of mean peak oISPC amplitude from three GRPR-INs is shown on the right. (I) The experimental approach used to generate the data presented in (J, K). (J) Filled arrowheads mark three examples of NPY-immunoreactive (green) inhibitory boutons synapsing onto dendrite of a Brainbow-labelled GRPR-IN (mTFP, blue). Inhibitory synapses were defined as VGAT-positive profiles (grey) in contact with gephyrin puncta (red). Open arrowheads mark two examples of NPY-negative inhibitory synapses on the Brainbow-labelled dendrite. Scale bar = 2 µm. (K) Quantification of the percentage of inhibitory synapses on to nine GRPR-INs (n = 3 mice), or in the vicinity of those cells, at which the presynaptic VGAT bouton is NPY-immunoreactive. Data are shown as individual values with mean ± standard error of the mean (SEM) in (B, C, G), individual values in (H) and individual matched values with mean ± SEM in (K). *p < 0.05, **p < 0.01, ****p < 0.0001; two-way ANOVA with Tukey’s post-test in (B, C), Fisher’s exact test with Bonferroni correction in (E) and Wilcoxon matched pairs test in (K).
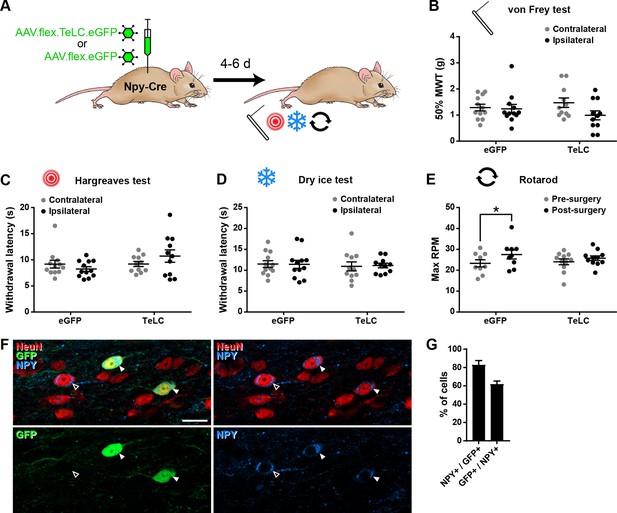
Silencing of NPY-INs does not affect nociceptive thresholds.
(A) The experimental approach used to generate data presented in this figure. Results of mechanical (B), radiant heat (C), and cold (D) nociceptive testing of hindpaws of Npy-Cre mice ipsi- and contralateral to spinal injections of AAV.flex.TeLC.eGFP or AAV.flex.eGFP carried out 4–6 days earlier. These analyses were performed on the same Npy-Cre mice as in Figure 5B–E (n = 12 for AAV.flex.eGFP, 11 for AAV.flex.TeLC.eGFP). No statistically significant differences were seen in any of the sensory modalities (repeated-measures two-way ANOVA with Šidák’s post-test). (E) Expression of TeLC and/or eGFP in NPY-INs did not impair motor co-ordination, as assessed by the accelerating rotarod test in the same Npy-Cre mice as above. A mild improvement in performance was observed in the AAV.flex.eGFP group compared to pre-surgery values, most likely reflecting a training effect (*p = 0.0137, repeated-measures two-way ANOVA with Šidák’s post-test). (F) Example confocal image (single optical section) from laminae II/II in the L4 segment of an Npy-Cre mouse that received spinal injections of AAV.flex.TeLC.eGFP. Two NeuN-immunoreactive cells (red) that co-express GFP (green) and neuropeptide Y (NPY; blue) are marked with filled arrowheads. Another NPY-positive cell that does not express GFP is marked with an open arrowhead. Scale bar = 20 µm. (G) Quantification of the overlap between GFP and NPY immunoreactivity in laminae I–III of the L4 segment of four Npy-Cre mice that received spinal injections of AAV.flex.TeLC.eGFP. Data are shown as individual values with mean ± standard error of the mean (SEM) in (B–E) and mean ± SEM in (G).
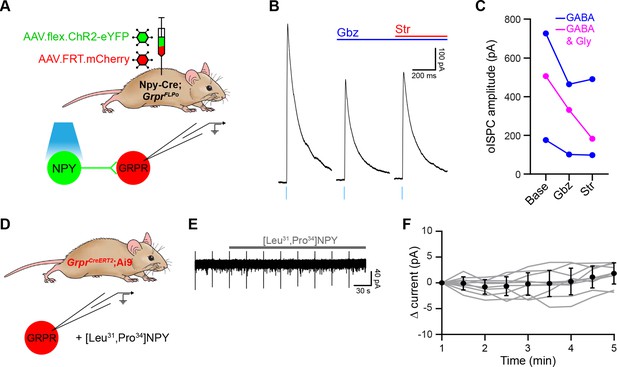
NPY-INs generate GABAergic and glycinergic inhibition of GRPR-INs.
(A) The experimental approach used to generate data presented in (B, C). (B) Representative optogenetically evoked IPSCs (oIPSCs) recorded in a GRPR-IN in the absence and presence of gabazine (Gbz) and strychnine (Str). Traces show an average of six stimuli, light blue bars denote period of optogenetic stimulation. (C) Quantification of the mean peak amplitude of oIPSCs recorded in the absence (Base) and presence of gabazine and strychnine (n = 3 cells). (D) The experimental approach used to generate data shown in (E, F). (E) Representative trace recorded in a GRPR-IN prior to and during application of the NPY Y1R receptor agonist, [Leu31,Pro34]-neuropeptide Y. (F) Quantification of the change in current recorded during the application of [Leu31,Pro34]-neuropeptide Y, compared to the mean current measured during the 1-min baseline period. All cells tested (n = 10) were classified as non-responders as they did not display an outward current of at least 5 pA. Grey lines denote trajectories for individual cells, black dots and bars denote mean ± standard deviation (SD).
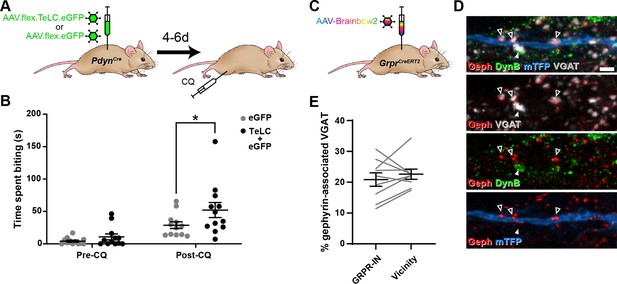
Toxin-mediated silencing of dynorphin-expressing inhibitory interneurons enhances pruritogen-evoked itch.
(A) The experimental approach used to generate the data presented in (B). (B) Silencing of Dyn-INs by viral expression of tetanus toxin light chain (TeLC) in PdynCre mice results in a significant enhancement of chloroquine-evoked itch (Post-CQ), compared to eGFP-expressing controls (p = 0.0379; repeated-measures two-way ANOVA with Šidák’s post-test). No significant difference was observed in biting time prior to injection with chloroquine (Pre-CQ; p = 0.7431; n = 12 for both GFP and TeLC groups). (C) The experimental approach used to generate the data presented in (D, E). (D) Filled arrowhead marks an example of a DynB-expressing (green) inhibitory bouton synapsing onto dendrite of a Brainbow-labelled GRPR-IN (mTFP, blue). Inhibitory synapses were defined as VGAT-positive profiles (grey) in contact with gephyrin puncta (red). Open arrowheads mark three examples of DynB-negative inhibitory synapses on the Brainbow-labelled dendrite. Scale bar = 2 µm. (E) Quantification of the percentage of total inhibitory synapses on to nine GRPR-INs (n = 3 mice), or in the vicinity of those cells, that contain DynB. No significant difference was observed between these proportions (p = 0.3438; Wilcoxon matched pairs test). Data are shown as individual values with mean ± standard error of the mean (SEM) in (B) and individual matched values with mean ± SEM in (E).

Injection of AAV.flex.TeLC.eGFP does not cause exaggerated itch in wild-type mice.
(A) The experimental approach used to generate data shown in (B). AAV.flex.eGFP (eGFP) or AAV.flex.TeLC.eGFP (TeLC) were injected into the L3 segments of wild-type mice (n = 8 for both groups), and itch-related behaviour was assessed 4–6 days later. (B) No differences were observed in the time spent biting the calf between the eGFP and TeLC groups in the 30 min prior to (Pre-CQ) or following (Post-CQ) injection of chloroquine (p = 0.9891 and 0.8919, respectively, repeated-measures two-way ANOVA with Šidák’s post-test). Data are shown as individual values with mean ± standard error of the mean (SEM).
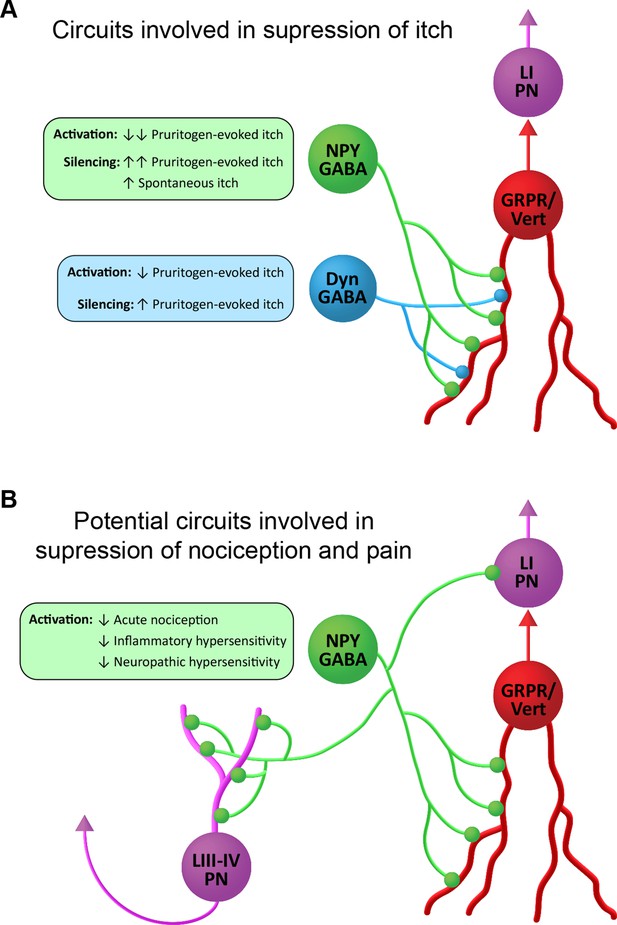
Suggested roles of neuropeptide Y (NPY)-expressing inhibitory interneurons in spinal itch and pain circuits.
(A) Circuits involved in suppression of itch. NPY-INs provide a high proportion (45%) of the inhibitory synapses on GRPR-expressing excitatory interneurons. The GRPR cells have vertical (Vert) morphology and are thought to transmit itch- and pain-related information to spinal projection neurons in lamina I (LI PNs). Pruritogen-evoked itch is markedly reduced by NPY-IN activation, while silencing of NPY-INs enhances pruritogen-evoked itch and results in spontaneous itch. Dyn-INs also provide inhibitory input to GRPR cells, but only account for ~20% of their inhibitory synapses. Silencing of Dyn-INs increases pruritogen-evoked itch, but to a lesser degree than NPY-IN silencing, and without the appearance of spontaneous itch. Activation of Dyn-INs has previously been shown to reduce itch in response to a range of pruritogens (see Huang et al., 2018). (B) Potential circuits involved in suppression of nociception and pain. Vertical cells are thought to be involved in the transmission of both normal and pathological pain signals through their input to LI PNs. Chemogenetic activation of GRPR-expressing vertical cells elicits both itch- and pain-related behaviours (Polgár et al., 2023). NPY-INs may therefore act to supress acute nociception, as well as inflammatory and neuropathic hypersensitivity, via inhibition of vertical cells. Additionally, NPY-INs have previously been shown to directly innervate nociceptive projection neurons of the anterolateral system in lamina I and laminae III–IV (LIII–IV PN).
Tables
Reagent type (species) or resource | Designation | Source or reference | Identifiers | Additional information |
---|---|---|---|---|
Strain, strain background (Mus musculus) | STOCK Tg(Npy-cre)RH26Gsat/Mmucd (Npy-Cre) | GENSAT/MMRRC | Cat#: 34810-UCD RRID: MMRRC_34810-UCD | |
Strain, strain background (Mus musculus) | B6.Cg-Gt(ROSA)26Sortm9(CAG-tdTomato)Hze/J (Ai9) | Prof. Hongkui Zheng | Cat#: 007909 RRID: IMSR_JAX:007909 | Available from The Jackson Laboratory, ME, USA |
Strain, strain background (Mus musculus) | GRPR-iCreERT2 (GrprCreERT2) | Dr. Yan-Gang Sun | Liu et al., 2019 | |
Strain, strain background (Mus musculus) | GrprFLPo | Dr. Yan-Gang Sun | Mu et al., 2017 | |
Strain, strain background (Mus musculus) | B6;129S-Pdyntm1.1(cre)Mjkr/LowlJ (PdynCre) | The Jackson Laboratory, ME, USA | Cat#: 027958 RRID: IMSR_JAX:027958 | |
Strain, strain background (Adeno-associated virus) | AAV1 pCAG-FLEX-EGFP-WPRE (AAV.flex.eGFP; 1.72 × 109 GC) | Penn Vector Core, PA, USA; available from Addgene, MA, USA (deposited by Hongkui Zeng) | Cat#: 51502 RRID: Addgene_51502 | Oh et al., 2014 |
Strain, strain background (Adeno-associated virus) | AAV1 pCAG-FLEX-tdTomato-WPRE (AAV.flex.tdTomato; 1.76 × 109 GC) | Penn Vector Core, PA, USA; available from Addgene, MA, USA (deposited by Hongkui Zeng) | Cat#: 51503 RRID: Addgene_51503 | Oh et al., 2014 |
Strain, strain background (Adeno-associated virus) | pAAV1-EF1a-double floxed-hChR2(H134R)-EYFP-WPRE-HGHpa (AAV.ChR2-eYFP; 5.09 × 108 GC) | Penn Vector Core, PA, USA; available from Addgene, MA, USA (deposited by Karl Deisseroth) | Cat#: 20298 RRID: Addgene_20298 | |
Strain, strain background (Adeno-associated virus) | rAAV2/hSyn-DIO-hm3D-mcherry (AAV.flex.hM3Dq-mCherry; 3.8 × 108 GC) | University of North Carolina Vector Core, NC, USA; available from Addgene, MA, USA (deposited by Bryan Roth) | Cat#: 44361 RRID: Addgene_44361 | Krashes et al., 2011 |
Strain, strain background (Adeno-associated virus) | ssAAV-2/2-hSyn1-dlox-hM3D(Gq)_mCherry(rev)-dlox-WPRE-hGHp(A) (AAV.flex.hM3Dq-mCherry; 7.65 × 108 GC) | Viral Vector Facility, University of Zurich, Switzerland | Cat#: v89-2 | |
Strain, strain background (Adeno-associated virus) | ssAAV-2/2-hSyn1-dlox-EGFP(rev)-dlox-WPRE-hGHp(A) (AAV.flex.eGFP; 2 × 108 GC) | Viral Vector Facility, University of Zurich, Switzerland | Cat#: v115-2 | |
Strain, strain background (Adeno-associated virus) | pssAAV-2-hSyn1-chI-dlox-EGFP_2A_FLAG_TeTxLC(rev)-dlox-WPRE-SV40p(A) (AAV.flex.TeLC.eGFP; 2 × 108 GC) | Viral Vector Facility, University of Zurich, Switzerland | Cat#: v322-2 | |
Strain, strain background (Adeno-associated virus) | ssAAV-8/2-hSyn1-dFRT-mCherry(rev)-dFRT-WPRE-hGHp(A) (AAV.FRT.mCherry; 8.7 × 108 GC) | Viral Vector Facility, University of Zurich, Switzerland | Cat#: v188-8 | |
Strain, strain background (Adeno-associated virus) | AAV9-EF1a-BbChT (AAV-Brainbow2; 1.5–5.96 × 107 GC) | Addgene, MA, USA; Deposited by Dawen Cai & Joshua Sanes | Cat#: 45186-AAV9 RRID: Addgene_45186 | Cai et al., 2013 |
Antibody | anti-Calretinin (Goat polyclonal) | SWANT, Bellinoza, Switzerland | Cat#: CG1 RRID: AB_10000342 | IF (1:1000) |
Antibody | anti-cFOS (Goat polyclonal) | Santa Cruz Biotechnology Inc, CA, USA | Cat#: sc-52-G RRID: AB_2629503 | IF (1:2000) |
Antibody | anti-DynorphinB (Rabbit polyclonal) | Dr. Philippe Ciofi, INSERM, France | Cat#: IS-35 RRID: AB_2819033 | IF (1:500) |
Antibody | anti-Galanin (Rabbit polyclonal) | Peninsula Laboratories, CA, USA | Cat#: T-4334 RRID: AB_518348 | IF (1:1000) |
Antibody | anti-Gephyrin (Mouse monoclonal) | SynapticSystems, Göttingen, Germany | Cat#: 147021 RRID: AB_2232546 | IF (1:500) |
Antibody | anti-GFP (Chicken polyclonal) | Abcam plc., Cambridge, UK | Cat#: ab13970 RRID: AB_300798 | IF (1:1000) |
Antibody | anti-mCherry (Chicken polyclonal) | Abcam plc., Cambridge, UK | Cat#: ab205402 RRID: AB_2722769 | IF (1:10,000) |
Antibody | anti-mCherry (Rat monoclonal) | Invitrogen; Thermo Fisher Scientific, UK | Cat#: M11217 RRID: AB_2536611 | IF (1:1000) |
Antibody | anti-mTFP (Rat polyclonal) | Kerafast Inc, Boston, MA, USA | Cat#: EMU108 | IF (1:500) |
Antibody | anti-NeuN (Chicken polyclonal) | SynapticSystems, Göttingen, Germany | Cat#: 266006 RRID: AB_2571734 | IF (1:1000) |
Antibody | anti-NeuN (Guinea pig polyclonal) | SynapticSystems, Göttingen, Germany | Cat#: 266004 RRID: AB_2619988 | IF (1:500) |
Antibody | anti-nNOS (Rabbit polyclonal) | MilliporeSigma, MA, USA | Cat#: 07-571 RRID: AB_310722 | IF (1:2000) |
Antibody | anti-NPY (Rabbit polyclonal) | Peninsula Laboratories, CA, USA | Cat#: T-4070 RRID: AB_518504 | IF (1:1000) |
Antibody | anti-Parvalbumin (Guinea pig polyclonal) | Frontier Institute Co. Ltd, Hokkaido, Japan | Cat#: PV-GP-Af1000 RRID: AB_2336938 | IF (1:2500) |
Antibody | anti-PAX2 (Rabbit polyclonal) | Invitrogen; Thermo Fisher Scientific, UK | Cat#: 71-6000 RRID: AB_2533990 | IF (1:1000) |
Antibody | anti-PAX2 (Rabbit polyclonal) | MilliporeSigma, MA, USA | Cat#: HPA047704 RRID: AB_2636861 | IF (1:200) |
Antibody | anti-VGAT (Goat polyclonal) | Frontier Institute Co. Ltd, Hokkaido, Japan | Cat#: VGAT-Go-Af620 RRID: AB_2571623 | IF (1:1000) |
Antibody | Alexa Fluor 488 Donkey Anti-Chicken IgY (Donkey polyclonal) | Jackson ImmunoResearch, PA, USA | Cat#: 703-545-155 RRID: AB_2340375 | IF (1:500) |
Antibody | Alexa Fluor 488 Donkey Anti-Goat IgG (Donkey polyclonal) | Jackson ImmunoResearch, PA, USA | Cat#: 705-545-003 RRID: AB_2340428 | IF (1:500) |
Antibody | Alexa Fluor 488 Donkey Anti-Rabbit IgG (Donkey polyclonal) | Jackson ImmunoResearch, PA, USA | Cat#: 711-545-152 RRID: AB_2313584 | IF (1:500) |
Antibody | Alexa Fluor 647 Donkey Anti-Chicken IgY (Donkey polyclonal) | Jackson ImmunoResearch, PA, USA | Cat#: 703-605-155 RRID: AB_2340379 | IF (1:500) |
Antibody | Alexa Fluor 647 Donkey Anti-Goat IgG (Donkey polyclonal) | Jackson ImmunoResearch, PA, USA | Cat#: 705-605-147 RRID: AB_2340437 | IF (1:500) |
Antibody | Alexa Fluor 647 Donkey Anti-Guinea pig IgG (Donkey polyclonal) | Jackson ImmunoResearch, PA, USA | Cat#: 706-605-148 RRID: AB_2340476 | IF (1:500) |
Antibody | Alexa Fluor 647 Donkey Anti-Rabbit IgG (Donkey polyclonal) | Jackson ImmunoResearch, PA, USA | Cat#: 711-605-152 RRID: AB_2492288 | IF (1:500) |
Antibody | Biotin-SP Donkey Anti-Goat IgG (Donkey polyclonal) | Jackson ImmunoResearch, PA, USA | Cat#: 705-065-147 RRID: AB_2340397 | IF (1:500) |
Antibody | Biotin-SP Donkey Anti-Guinea pig IgG (Donkey polyclonal) | Jackson ImmunoResearch, PA, USA | Cat#: 706-065-148 RRID: AB_2340451 | IF (1:500) |
Antibody | Biotin-SP Donkey Anti-Rabbit IgG (Donkey polyclonal) | Jackson ImmunoResearch, PA, USA | Cat#: 711-065-152 RRID: AB_2340593 | IF (1:500) |
Antibody | Biotin-SP Donkey Anti-Rat IgG (Donkey polyclonal) | Jackson ImmunoResearch, PA, USA | Cat#: 712-065-153 RRID: AB_2315779 | IF (1:500) |
Antibody | Rhodamine Red-X Donkey Anti-Chicken IgY (Donkey polyclonal) | Jackson ImmunoResearch, PA, USA | Cat#: 703-295-155 RRID: AB_2340371 | IF (1:100) |
Antibody | Rhodamine Red-X Donkey Anti-Guinea pig IgG (Donkey polyclonal) | Jackson ImmunoResearch, PA, USA | Cat#: 706-295-148 RRID: AB_2340468 | IF (1:100) |
Antibody | Rhodamine Red-X Donkey Anti-Mouse IgG (Donkey polyclonal) | Jackson ImmunoResearch, PA, USA | Cat#: 715-295-151 RRID: AB_2340832 | IF (1:100) |
Antibody | Rhodamine Red-X Donkey Anti-Rabbit IgG (Donkey polyclonal) | Jackson ImmunoResearch, PA, USA | Cat#: 711-295-152 RRID: AB_2340613 | IF (1:100) |
Antibody | Rhodamine Red-X Donkey Anti-Rat IgG (Donkey polyclonal) | Jackson ImmunoResearch, PA, USA | Cat#: 712-295-153 RRID: AB_2340676 | IF (1:100) |
Sequence-based reagent (RNAscope probe) | Mm-Npy-C3 | ACD BioTechne, CA, USA | Cat#: 313321-C3 | |
Sequence-based reagent (RNAscope probe) | Cre-C2 | ACD BioTechne, CA, USA | Cat#: 312281-C2 | |
Peptide, recombinant protein | [Leu31,Pro34]-neuropeptide Y | Tocris, Abingdon, UK | Cat#: 1176 | |
Chemical compound, drug | Clozapine-N-oxide (CNO) | Tocris, Abingdon, UK | Cat#: 4936 | |
Chemical compound, drug | CNO-dihydrochloride | Tocris, Abingdon, UK | Cat#: 6329 | |
Chemical compound, drug | Gabapentin | Sigma-Aldrich, Glasgow, UK | Cat#: PHR1049-1G | |
Chemical compound, drug | SR95531 (Gabazine) | Abcam, Cambridge, UK | Cat#: ab120042 | |
Chemical compound, drug | Strychnine hydrochloride | Sigma-Aldrich, Glasgow, UK | Cat#: S8753 | |
Chemical compound, drug | Tetrodotoxin citrate (TTX) | Alamone Labs, Jerusalem, Israel | Cat#: T-550 | |
Chemical compound, drug | NBQX disodium salt | Abcam, Cambridge, UK | Cat#: ab120046 | |
Chemical compound, drug | D-APV | Tocris, Abingdon, UK | Cat#: 0106 | |
Chemical compound, drug | 4-Aminopyridine (4-AP) | Sigma-Aldrich, Glasgow, UK | Cat#: 275875 | |
Chemical compound, drug | BMS 139885 | Tocris, Abingdon, UK | Cat#: 3242 | |
Chemical compound, drug | (−)-Bicuculline methobromide | Tocris, Abingdon, UK | Cat#: 0109 | |
Chemical compound, drug | Chloroquine diphosphate salt | Sigma-Aldrich, Glasgow, UK | Cat#: C6628 | |
Chemical compound, drug | Complete Freund’s adjuvant (CFA) | Sigma-Aldrich, Glasgow, UK | Cat#: F5881 | |
Software, algorithm | Neurolucida | MBF Bioscience, VT, USA | RRID: SCR_001775 | https://www.mbfbioscience.com/neurolucida |
Software, algorithm | Neurolucida Explorer | MBF Bioscience, VT, USA | RRID: SCR_017348 | https://www.mbfbioscience.com/neurolucida-explorer |
Software, algorithm | pClamp | Molecular Devices, CA, USA | RRID: SCR_011323 | https://www.moleculardevices.com/products/axon-patch-clamp-system/acquisition-and-analysis-software/pclamp-software-suite#gref |
Software, algorithm | Zen Black | Carl Zeiss, Germany | RRID: SCR_018163 | https://www.zeiss.com/microscopy/int/products/microscope-software/zen.html |
Software, algorithm | Prism | GraphPad Software, CA, USA | RRID: SCR_002798 | https://www.graphpad.com/scientific-software/prism/ |
Software, algorithm | Behavioral Observation Research Interactive Software (BORIS) | Oliver Friard & Marco Gamba, University of Torino, Italy | RRID: SCR_021434 | https://www.boris.unito.it/ |