Predicting ventricular tachycardia circuits in patients with arrhythmogenic right ventricular cardiomyopathy using genotype-specific heart digital twins
Figures
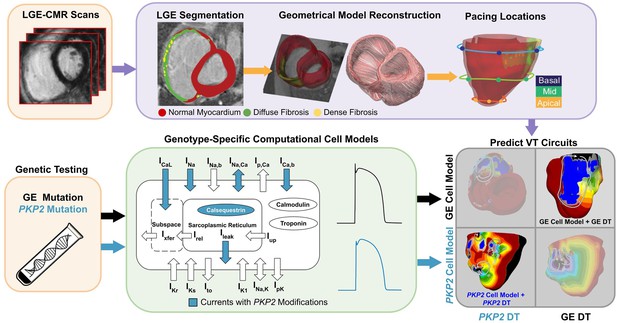
Overview of the study.
The flowchart summarizes the workflow of using Geno-DT to understand ARVC arrhythmogenesis and to predict VTs in ARVC patients of the two genotype groups, PKP2 and GE. Geno-DT integrates genotype-specific cell models (green) and patient-specific clinical-image-based heart digital twin modeling. Orange blocks refer to clinical data, which include genetic testing results and LGE-MRI images for each patient in the cohort. Patient-specific geometrical heart models were reconstructed from the LGE-CMR (top, purple, left and middle images). Genotype-specific cell models (green) developed here were incorporated into each heart model based on the patient’s genetic testing result. The integrated multi-scale (from the subcellular to the organ), patient-specific Geno-DT models were subjected to rapid pacing from multiple sites on the RV (top purple, rightmost image) to understand the role of remodeling in ARVC arrhythmogenesis and to predict VT circuits (bottom gray).
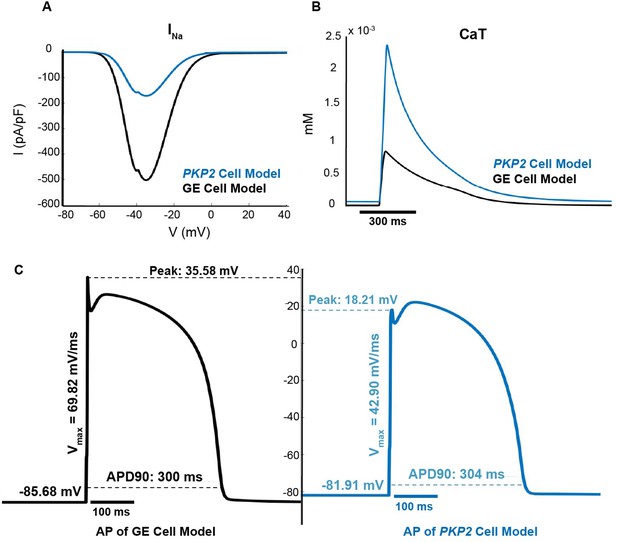
The PKP2 cell model has lower excitability and altered calcium-cycling as compared to the GE model.
(A) Current-voltage curves for INa; (B) Calcium transient curves; (C) Action potentials at steady state at 1 Hz pacing.
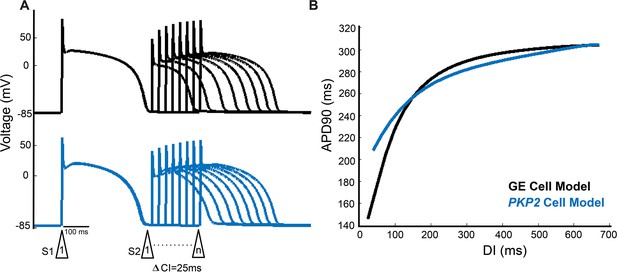
The PKP2 cell model exhibits poorer rate adaptation and a flatter electrical restitution curve as compared to the GE model.
(A) Representative premature APs at DIs decrementing to just before loss of one-to-one capture of GE cell model (top) and PKP2 cell model (bottom). (B) APD restitution curves of GE and PKP2 cell models.
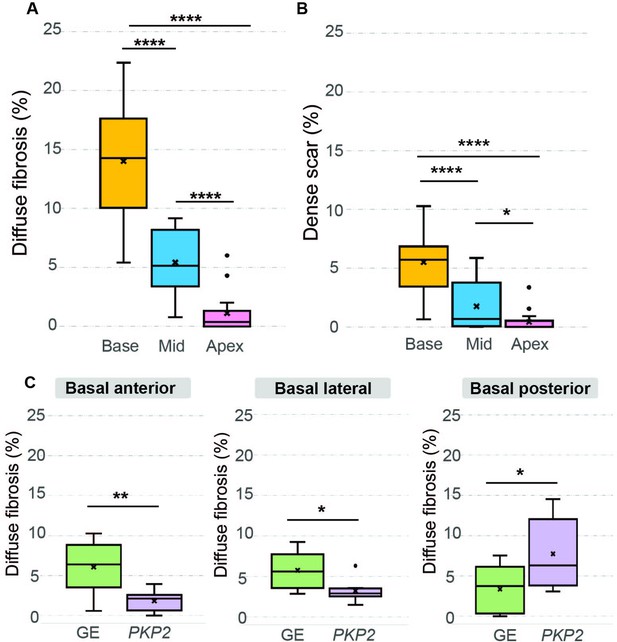
Amounts of diffuse fibrosis and dense scar in different regions of the RV and in the two patient groups.
(A, B) Boxplots showing the amount of diffuse fibrosis (A, Base: n = 16, interquartile range [IQR] = 7.43; Mid: n = 16, IQR = 4.65; Apex: n = 16, IQR = 1.18; ****p < 0.0001) and dense scar (B, Base: n = 16, IQR = 2.73; Mid: n = 16, IQR = 3.14; Apex: n = 16, IQR = 0.36; *p < 0.05, ****p < 0.0001) in the entire ARVC cohort in different regions of the RV. (C) Boxplots comparing diffuse fibrosis amounts in different RV AHA segments between the two genotype groups. Significance was found in the following basal segments: basal anterior (GE: n = 8, IQR = 2.91; PKP2: n = 8, IQR = 1.78; **p < 0.01), basal lateral (GE: n = 8, IQR = 3.21; PKP2: n = 8, IQR = 0.75; *p < 0.05), and basal posterior (GE: n = 8, IQR = 4.74; PKP2: n = 8, IQR = 7.25; *p < 0.05). Amounts of diffuse fibrosis/dense scar are normalized with respect to the patient’s total RV tissue volume. Median and interquartile range were represented in each boxplot, where dots indicate outliers. Paired Student’s t-tests were applied to assess statistical significance.
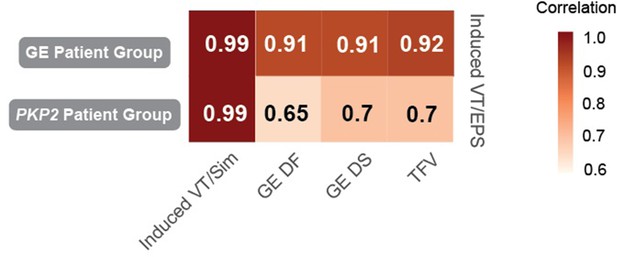
Correlation between the number of VTs induced during the EPS and structural remodeling features across different RV anatomical locations in ARVC patients.
The number of VT episodes induced during EPS is highly correlated with the volume of structural remodeling (DF, DS, TFV) in GE patient group (top row), while correlated less in PKP2 patient group (bottom row). The VT episodes induced during EPS and by Geno-DT are highly correlated in both patient groups (first column). Numbers in the block stand for the correlation coefficient (r) between the two corresponding variables. r > 0.7 stands for high correlation; 0.5 < r ≤ 0.7 stands for moderate correlation. Induced VT/EPS: VTs induced during the clinical EPS; Induced VT/Sim: VTs induced in Geno-DTs; DF: diffuse fibrosis; DS: dense scar; VT: ventricular tachycardia, TFV: total fibrotic volume = DF + DS.
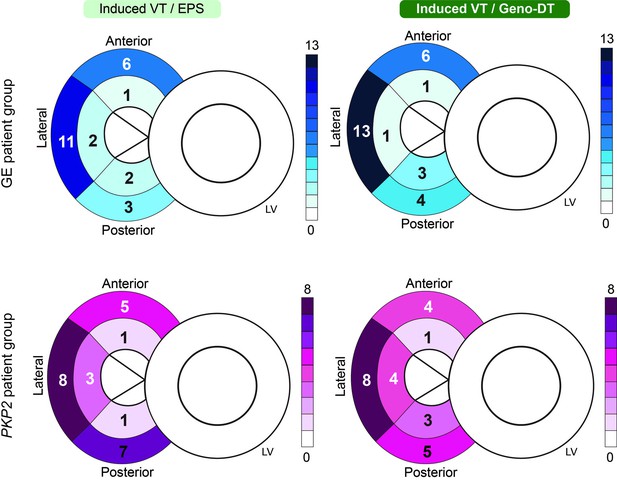
Comparison of the number of unique VT morphologies induced in Geno-DTs to VTs induced during EPS in each RV region.
Schematics of the heart were labeled with the numbers of unique VT morphologies at different AHA segments on the RV induced during clinical EPS (left) and induced in Geno-DTs (right) of the two ARVC genotypes (GE: blue, PKP2: pink).
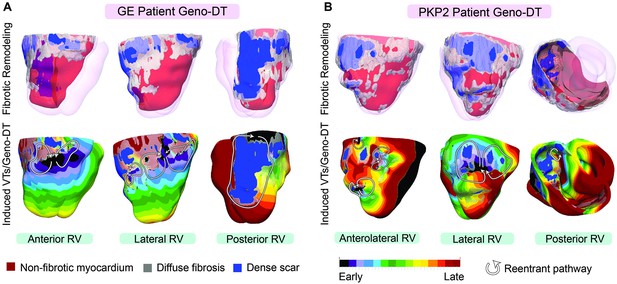
VTs induced by Geno-DT in two ARVC patients each from the GE and PKP2 genotype groups.
(A, B) The top row shows three different views of reconstructed geometrical models of GE and PKP2 patient hearts with personalized diffuse fibrosis (gray) and dense scar (blue). The bottom row shows the activation patterns of the VT reentrant circuits induced by Geno-DT rapid pacing.
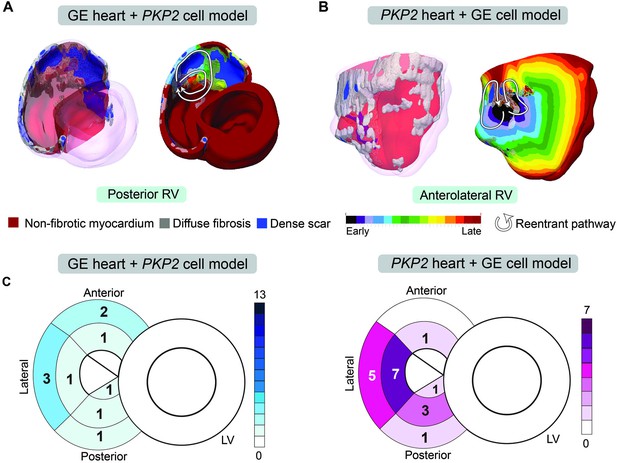
Genotype-mismatched DTs significantly underpredicted VTs in ARVC patients.
(A, B) Geometrical ventricular models with structural substrates visualized (left); VT circuits induced in genotype-mismatched DTs (right). (C) Bullseye plots labeled with the number of unique VT morphologies in different RV AHA segments induced in Geno-DTs under genotype-mismatched conditions.
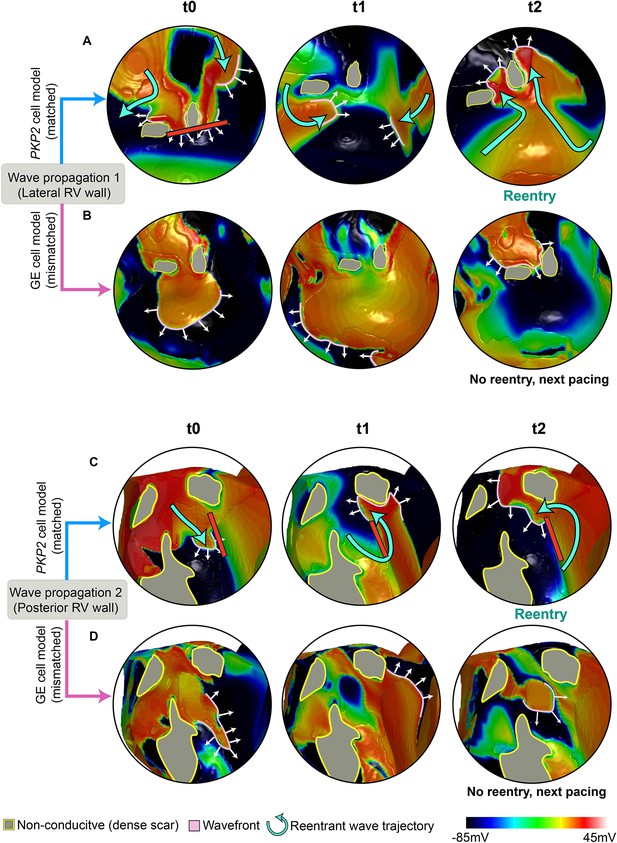
Comparison of propagation following pacing in a PKP2 patient’s ventricular model using different cell models.
Each row of the figures shows a series of frames that depict the continuous wave propagation in a portion of the PKP2 patient’s DT from Figure 7B (genotype-matched conditions, [A] and [C]) and Figure 8B (genotype-mismatched conditions, [B] and [D]). The images in each column (A and B, C and D) show the same time instant. Pink lines mark the wavefronts, white arrows indicate direction of propagation and cyan curved arrows in (A) and (C) represent reentrant wave trajectories. Gray areas contoured by yellow represent the dense scar (DS) regions that are non-conductive. (A, B) (A) and (B) compare the wave propagation on the lateral RV wall between genotype-matched and -mismatched conditions. At t0, the wavefront in (A) propagates slower than that in (B), resulting in conduction block in (A) but not in (B). In (A), following wavefront fusion at t1, reentry is established at t2. In (B), no reentry forms and the next pacing stimulus captures. (C, D) (C) and (D) compare the wave propagation on posterior RV wall between genotype-matched and -mismatched conditions. At t0, the wavefront in (C) propagates slower than that in (D). Due to the extended refractoriness in PKP2, tissue adjacent to the wavefront is still recovering, and conduction block takes place (red line). In (D), tissue ahead of the wavefront is fully recovered, allowing it to propagate through. At t1, in (C), the wavefront, with a high curvature, travels around the conduction block. In (D), the wavefront is more planar and continues to propagate. At t2, a reentrant circuit forms in (C); while in (D), no reentry forms and the next pacing stimulus captures.
Animation of two VT circuits propagation corresponding to Figure 9.
The video presents side-by-side comparison of the wavefront propagation induced by Geno-DT under genotype-matched and genotype-mismatched conditions, with labels of pacing locations, VT morphologies and conduction blocks.
Tables
Patient characteristics (n = 16).
Clinical characteristics | ARVC GE patients (n = 8) | ARVC PKP2 patients (n = 8) | p value |
---|---|---|---|
Male | 2 {25} | 2 {25} | - |
Age at CMR, years | 31.0 [22-45] | 35.3 [18-55] | 0.55 |
Age at first clinical VT, years | 34.0 [22-45] | 31.0 [17-55] | 0.94 |
ICD implantation | 7 {88} | 7 {88} | - |
Beta blocker | 8 {100} | 5 {63} | 0.056 |
Sodium channel blocker | 2 {25} | 3 {38} | 0.59 |
Syncope | 2 {25} | 2 {25} | - |
Cardiac function | |||
RV hypokinesis | 4 {50} | 6 {75} | 0.15 |
RVEF (%) | 37.6±5.5 | 36.1±9.7 | 0.76 |
RVCO (L/min) | 4.7±1.2 | 4.9±0.9 | 0.72 |
RVEDVI (ml/m2) | 131.8±31.2 | 144.5±63.3 | 0.66 |
LVEF (%) | 57.2±6.5 | 55.7±10.0 | 0.74 |
LVEDVI (ml/m2) | 89.3±9.2 | 89.7±23.0 | 0.97 |
LVCO (L/min) | 5.1±0.8 | 5.0±0.9 | 0.86 |
-
Values are given as n {%}, mean [range], or mean ± standard deviation. p Values were calculated using Student’s t-test and z-test with p ≤ 0.05 as statistically significant. CMR = cardiac magnetic resonance; VT = ventricular tachycardia; ICD = implantable cardiac defibrillator; RV = right ventricle; LV = left ventricle; RVEF = right ventricular ejection fraction; RVCO = right ventricular cardiac output; RVEDVI = right ventricular end-diastolic volume index; LVEF = left ventricular ejection fraction; LVCO = left ventricular cardiac output; LVEDVI = left ventricular end-diastolic volume index. The cardiac function parameters were obtained from CMR reports.
Capability of Geno-DTs to predict VT locations.
GE patient group (n = 8) | PKP2 patient group (n = 8) | |
---|---|---|
Sensitivity | 1.00 | 0.86 |
Specificity | 0.94 | 0.90 |
Accuracy | 0.96 | 0.89 |
Error rate | 0.042 | 0.11 |
F1 score | 0.89 | 0.83 |
MCC | 0.90 | 0.76 |