Targeting ribosome biogenesis as a novel therapeutic approach to overcome EMT-related chemoresistance in breast cancer
Figures
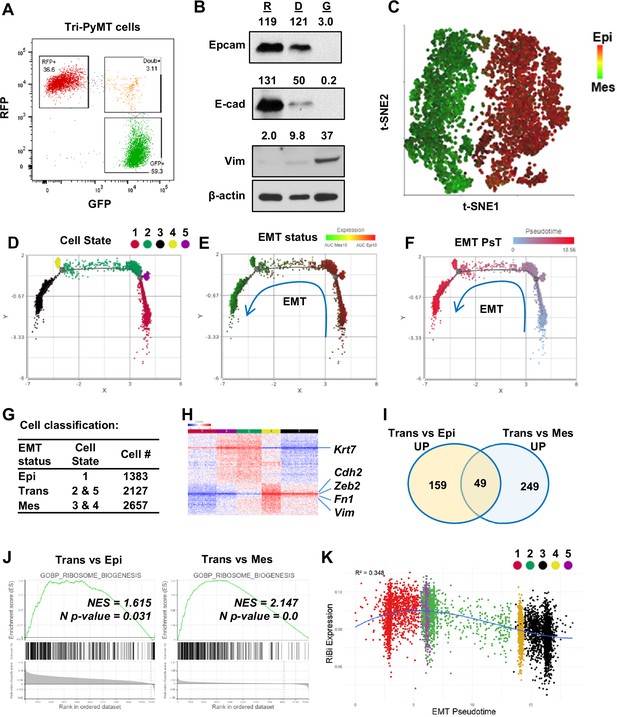
Activation of ribosome biogenesis pathway during the epithelial-to-mesenchymal transition (EMT) transitioning phase in Tri-PyMT cells.
(A) Representative flow cytometry plot displays the percentage of RFP+, Double+ (Doub+, EMT transitioning cells), and GFP + Tri PyMT cells in culture. (B) Western blot of EMT markers with flow-sorted RFP+ (R), Doub+ (D), and GFP+ (G) Tri-PyMT cells. The Doub+ cells exhibit an intermediate EMT status with expression of epithelial marker (E-cadherin and Epcam) expression and higher mesenchymal marker (Vimentin) expression as compared with RFP + cells. The numbers indicate the normalized intensity of the band according to the β-actin band of the sample. (C) The t-SNE plot of scRNA-seq analysis of Tri-PyMT cells. Two major cell clusters with differential EMT status are shown with the AUC value of 10 epithelial marker genes (in red) and 10 mesenchymal marker genes (in green). The marker genes are shown in Figure 1—source data 1. (D, E, F) Trajectory analysis using Monocle DDR tree. Cell trajectory analysis was performed with filtered EMT-related genes using the Monocle 2 model. Five cell states (D) were identified with differential EMT statuses. From epithelial to mesenchymal phenotype, the Cell States were identified in order of 1, 5, 2, 4, and 3. EMT status (E) of cells was also highlighted by the AUC calculation with epithelial and mesenchymal marker genes. EMT Pseudotime (F) was calculated with Cell State 1 (the most epithelial state) as the root. (G) Classification of cells with EMT states. Cells were classified according to their EMT state as Epi, Trans, and Mes; the cell number in each cluster is indicated in the table. (H) The heatmap of differentially expressed genes in Trans cells compared to Epi and Mes cells. Totally, 313 genes were identified with criteria p<0.01, Fold change >1.2, and Average expression ≥ 5. (I) The common enriched GO_BP pathways with gene set enrichment analysis (GSEA) when comparing Trans vs. Epi and Trans vs. Mes. There are 49 common pathways, including five pathways related to Ribosome Biogenesis (RiBi) or rRNA processing. Pathway names are shown in Figure 1—source data 2. (J) GSEA plots showing the specific enrichment of RiBi pathway in EMT transitioning (Trans) cells compared to the Epi or Mes cells. (K) The scatter plot displays the correlation of RiBi activity to EMT pseudotime. The polynomial regression line (order = 3) highlights the elevated RiBi pathway in Trans phase cells, R2=0.348, p<0.001.
-
Figure 1—source data 1
EMT marker genes that were used in calculation of AUCell value of EMT status.
- https://cdn.elifesciences.org/articles/89486/elife-89486-fig1-data1-v1.docx
-
Figure 1—source data 2
Enriched pathways in GSEAs of EMT transitioning cells.
- https://cdn.elifesciences.org/articles/89486/elife-89486-fig1-data2-v1.docx
-
Figure 1—source data 3
Raw western blot images of Figure 1B.
- https://cdn.elifesciences.org/articles/89486/elife-89486-fig1-data3-v1.zip
-
Figure 1—source data 4
Whole western blot images of Figure 1B with labels.
- https://cdn.elifesciences.org/articles/89486/elife-89486-fig1-data4-v1.pdf
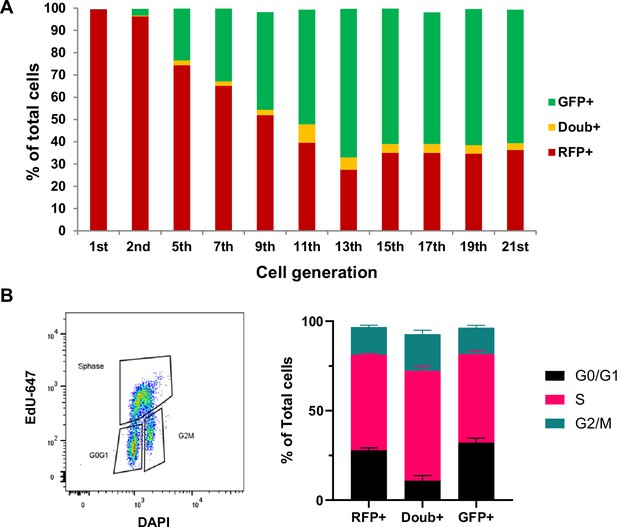
Characterization of fluorescent marker switch in Tri-PyMT cells.
(A) RFP + Tri PyMT cells were sorted via flow cytometry and cultured in DMEM with 10% FBS. Plot shows the percentage of RFP+, GFP + and Doub+ cells at different generations of cell culture. The experiment was repeated twice with similar results. (B) Cell cycle analysis of Tri-PyMT cells. Tri-PyMT cells (p7) composed by both RFP + and GFP + cells were cultured in a growth medium. Cell cycle analysis was performed by using EdU incorporation and Click-chemistry. Cells in the G0/G1, S, and G2/M phases were quantified by flow cytometry. n=4, two-way Anova, p=0.0242, for S phase RFP + vs GFP+; p<0.0001, for S phases Doub+ vs RFP + and Doub+ vs GFP + cells.
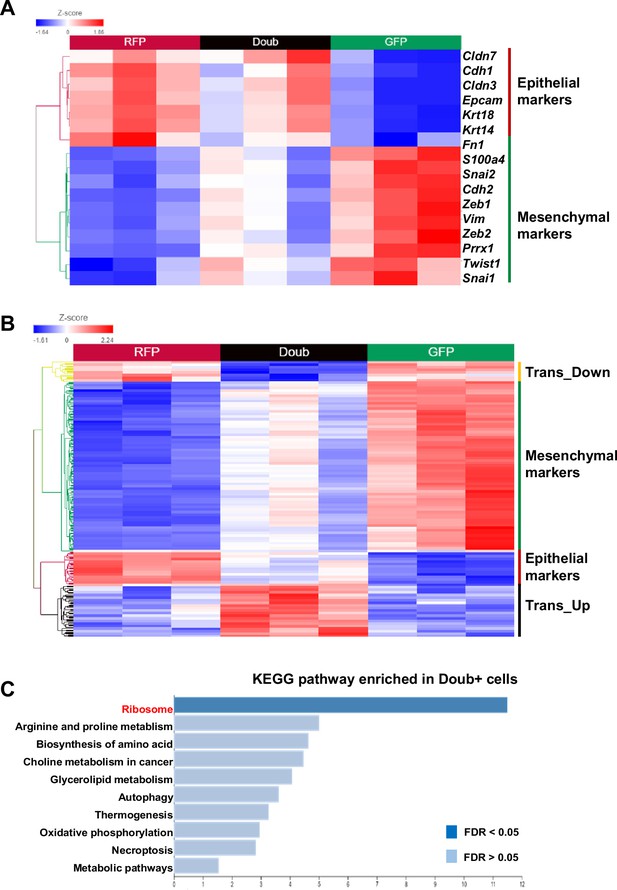
RNA-sequencing analysis of Tri-PyMT cells.
RFP + Doub+, and GFP + Tri PyMT cells were purified using flow cytometry sorting, total RNA was extracted and submitted for RNA-sequencing analysis. (A) Heatmap of selected epithelial-to-mesenchymal transition (EMT) marker genes in RFP+, Doub+, and GFP + cells. Of note, the intermediate expression of both epithelial and mesenchymal markers in Doub+ cells. (B) Heatmap of differentially expressed genes in Doub+ cells compared to RFP + and GFP + cells. In total, 213 genes are presented in 4 clusters, p<0.01, Doub+ vs RFP + and GFP+. (C) Gene set over-representative assay with upregulated genes in Doub+ cells showing the significant enrichment of Ribosome pathway (326 KEGG pathways analyzed in total).
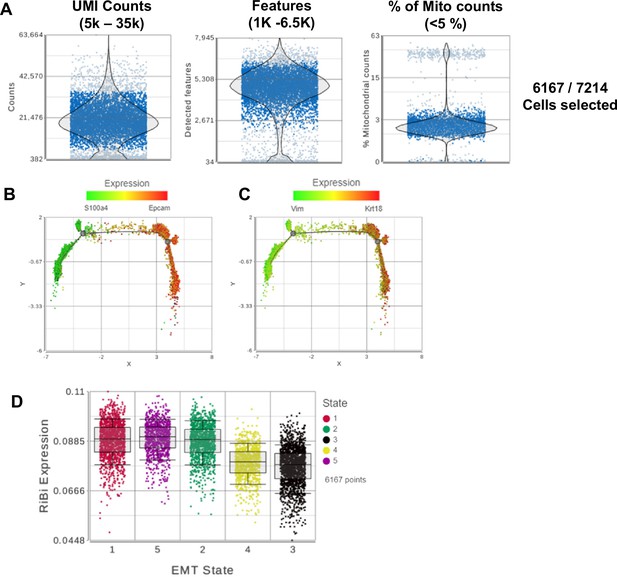
The single-cell RNA (scRNA)-sequencing analysis of Tri-PyMT cells.
RFP+, Doub+, and GFP + Tri PyMT cells were sorted via flow cytometry and submitted for scRNA-sequencing analysis. (A) Quality controls of the scRNA-seq. Plots show the single-cell QA/QC analyses including total counts of UMI, detected gene features, and the percentage of mitochondria genes. Totally, 6167 cells out of 7214 cells were filtered according to the criteria for downstream analyses. (B, C) Epithelial-to-mesenchymal transition (EMT) trajectory analysis plots. Cell trajectory analysis was performed with filtered EMT-related genes using the Monocle 2 model. Pairs of epithelial and mesenchymal marker genes, S100a4 vs. Epcam (B) and Vim vs. Krt18 (C), are used to highlight the EMT status of individual cells. (D) Scatter plot shows the expression of Ribosome biogenesis genes (AUC values of RiBi pathway genes) according to EMT cell states. A trend of highest ribosome biogenesis (RiBi) activation was detected in State 5 cells (p=0.072 for 5 vs 1, and p<0.01 for 5 vs 2, 3, 4, One-way ANOVA).
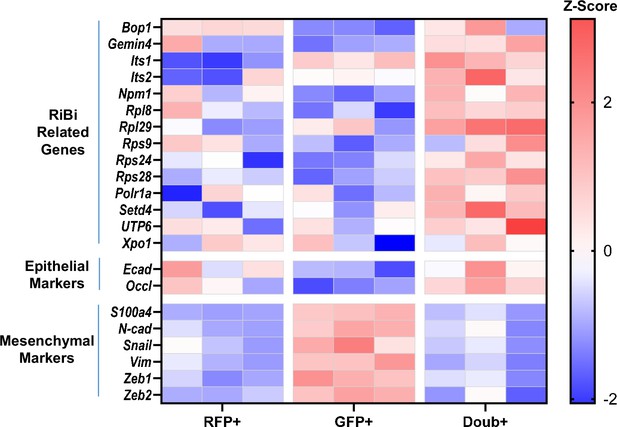
RT-PCR analysis of ribosome biogenesis (RiBi) genes in Tri-PyMT cells.
RFP+, Doub+, and GFP + cells were purified from Tri-PyMT (p7) culture using flow cytometry sorting. Total RNA was extracted and analyzed by RT-PCR. The heatmap shows the z-score of RiBi-related genes, epithelial and mesenchymal marker genes expression with Gapdh as internal control, n=3.
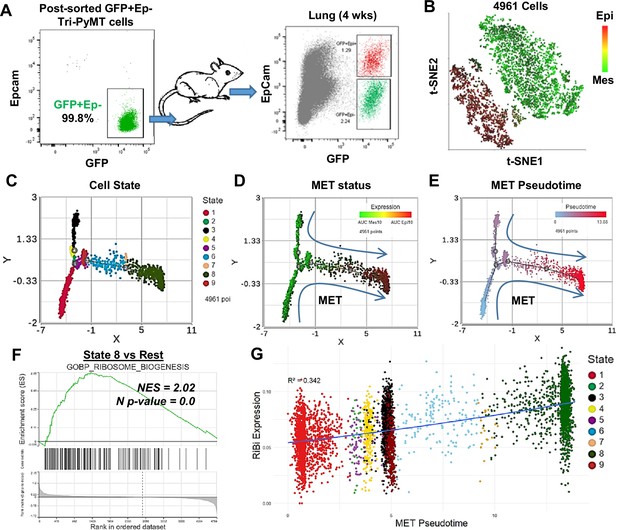
Activation of ribosome biogenesis (RiBi) pathway in mesenchymal-to-epithelial transition (MET) during lung metastasis outgrowth.
(A) Schematic of MET induction. GFP + Tri PyMT cells were sorted by flow cytometry (left) and injected into mice through the tail vein. Metastasis-bearing lungs were harvested after 4 weeks and analyzed for the gain of epithelial marker (EpCam) in tumor cells (right). Both Epcam + and Epcam- cells were sorted and submitted for single-cell RNA sequencing (scRNA-seq) analysis. (B) The t-SNE plot of scRNA-seq analysis of GFP + Tri PyMT cells sorted from metastatic lungs of three individual animals. Two major cell clusters with distinct epithelial-to-mesenchymal transition (EMT) statuses were identified, as indicated by the AUC values of 10 epithelial genes (in red) and 10 mesenchymal genes (in green). (C, D, E) Monocle 2 model-based cell trajectory analysis identifies nine cell states (C). The MET process is emphasized by AUC values of mesenchymal and epithelial markers (D). MET Pseudotime is calculated with State 1 (the most mesenchymal state) as the root (E). (F) Gene set enrichment analysis (GSEA) plot exhibits the enrichment of the GOBP_Ribosome Biogenesis (RiBi) pathway in State 8 cells (the most epithelial phenotype) compared to the other states. (G) Scatter plot illustrates the correlation between RiBi activity and MET pseudotime. A polynomial regression line (order = 3) emphasizes the elevated RiBi pathway during the MET process, R2=0.342, p<0.01.
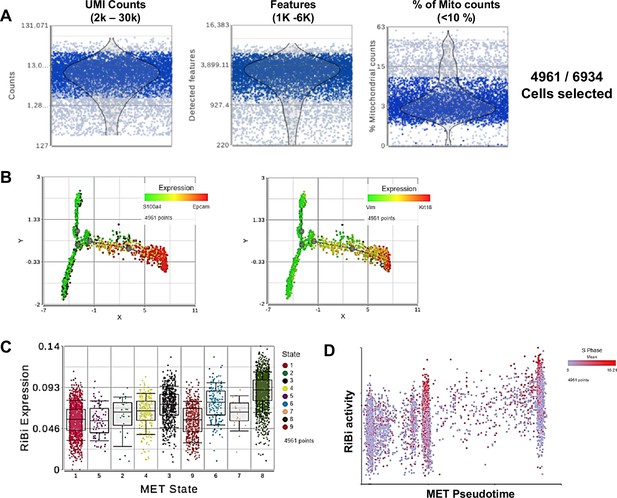
The single-cell RNA (scRNA)-sequencing analysis of GFP + Tri PyMT cells.
GFP + Tri PyMT cells, including Epcam + and Epcam- cells were sorted from metastasis-bearing lungs and submitted for single-cells RNA sequencing analysis. (A) Quality controls of the scRNA-seq. Plots show the single-cell QA/QC analyses of the total counts of UMI, detected gene features, and the percentage of mitochondria genes. Totally, 4961 cells out of 6934 cells were included in the downstream analyses. (B) Plots of mesenchymal-to-epithelial transition (MET) trajectory analysis. Cell trajectory analysis was performed with filtered EMTome genes using the Monocle 2 model. Pairs of epithelial and mesenchymal marker genes, S100a4 vs. Epcam (left) and Vim vs. Krt18 (right) are used to highlight the MET status of individual cells.(C) Elevated ribosome biogenesis pathway during MET. Cells were classified by their MET state. The activation of the ribosome biogenesis (RiBi) pathway was indicated by the AUC value of genes in the ribosome biogenesis pathway. Of note, the highest RiBi expression in MET State 8 cells, which exhibit the most epithelial phenotypes. (D) Scatter plot illustrates the correlation between RiBi activity and MET pseudotime. Proliferating cells (in S phase) are highlighted in red color.
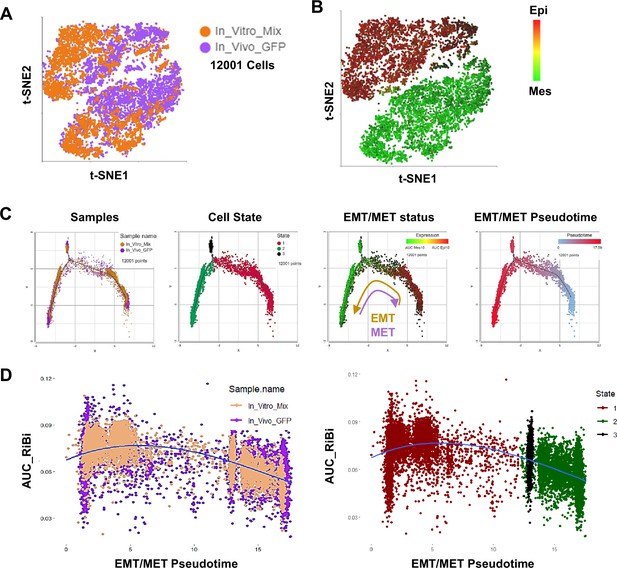
The single-cell RNA sequencing (scRNA-seq) analysis with merged epithelial-to-mesenchymal transition (EMT) and mesenchymal-to-epithelial transition (MET) cells.
To obtain a whole picture of both EMT and MET processes, we merged the scRNA-seq data exhibited in Figure 1 and Figure 2 and performed cell clustering and EMT/MET trajectory analysis together. (A) The t-SNE plot of merged data highlights the distribution of cells from EMT (In_Vitro_Mix) and MET (In_Vivo_GFP) cells. (B) The t-SNE plot exhibits the EMT status of cells. Two major cell clusters with differential EMT status are shown with the AUC value of 10 epithelial marker genes (in red) and 10 mesenchymal marker genes (in green). (C) EMT trajectory analysis with filtered EMT-related genes using Monocle 2 model. Plot highlight the origin samples, cell state detected, EMT/MET status with AUC values, and the EMT/MET pseudotime with Cell State 1 (the most epithelial state) as the root. (D) The scatter plot displays the correlation of ribosome biogenesis (RiBi) activity to EMT/MET pseudotime. Cells with lower EMT/MET pseudotime indicate a more epithelial-like phenotype. The polynomial regression line (order = 3) highlights the elevated RiBi pathway in the early EMT or late MET phases, R2=0.384361, p<0.001.
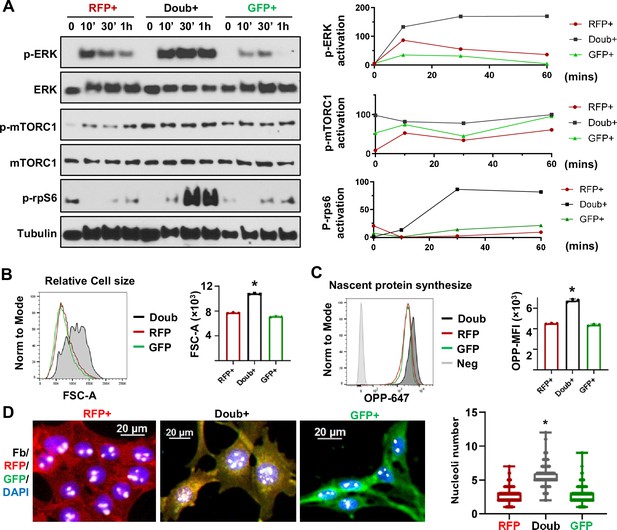
MAPK and mTOR pathways mediated ribosome biogenesis activation in epithelial-to-mesenchymal transition (EMT) transitioning phase cells.
(A) Western blots show phospho-ERK, total ERK, phospho-mTORC1, total mTORC1, and p-rps6 in sorted RFP+, Doub+, and GFP + Tri PyMT cells following stimulation with 10% FBS at 0, 10, 30, and 60 min. Activation of p-ERK, p-mTORC1, and p-Rps6 are quantified by the band intensity after normalizing to loading controls (right). (B) Cell size analysis. Tri-PyMT cells (p5) were analyzed by flow cytometry. The relative cell size was indicated by FSC-A, for individual RFP+, GFP+, and Doub + cells. Data from three biological replicates. (C) Nascent protein synthesis assay. Histogram of OPP incorporation, as measured by flow cytometry, demonstrates enhanced nascent protein synthesis in Doub + cells. The rate of new protein synthesis is assessed by adding fluorescently labeled O-propargyl-puromycin (OPP). Three biological replicates, One-way ANOVA, *p<0.0001, Doub + vs. RFP+, and Doub + vs. GFP+. (D) Fluorescent images reveal increased ribosome biogenesis (RiBi) activity in Doub + Tri PyMT cells, as evidenced by Fibrillarin staining. RFP+, Doub+, and GFP + Tri PyMT cells were sorted and stained for nucleoli using an anti-Fibrillarin antibody. The quantification of nucleoli per cell is shown on the right. n=522 (RFP+), 141 (Doub+), 289 (GFP+). One-way ANOVA, *p<0.0001.
-
Figure 3—source data 1
Raw western blot images of Figure 3A.
- https://cdn.elifesciences.org/articles/89486/elife-89486-fig3-data1-v1.zip
-
Figure 3—source data 2
Whole western blot images of Figure 3A with labels.
- https://cdn.elifesciences.org/articles/89486/elife-89486-fig3-data2-v1.pdf
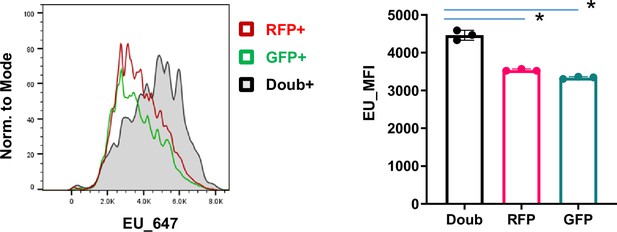
Elevated RNA transcription activity in the Doub+ Tri PyMT cells.
Tri-PyMT cells (p7) including both RFP + and GFP + cells were cultured in a growth medium. EU incorporation assay was performed and detected with click chemistry using Alexa A647-azide. Labeled cells were analyzed by flow cytometry (Left). The median fluorescence intensity (MFI) of EU staining of RFP+, GFP +, and Doub+ cells were quantified, n=3, One-way ANOVA, *p<0.0001, Doub+ vs. RFP+; *p<0.0001, Doub+ vs. GFP+.
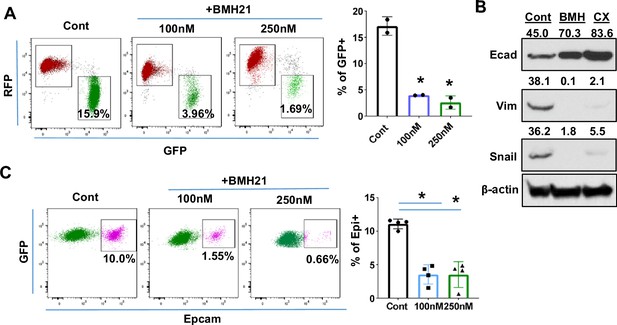
Ribosome biogenesis (RiBi) inhibition by Pol I inhibitor reduces the epithelial-to-mesenchymal transition (EMT)/mesenchymal-to-epithelial transition(MET) capability of tumor cells.
(A) Flow cytometry plots display the percentage of GFP + cells following BMH21 treatment. RFP+/EpCam + Tri PyMT cells were sorted and cultured in a growth medium with or without BMH21 for 5 days. Percentage of GFP + cells was analyzed by flow cytometry. Biological repeat, n=2, One-way ANOVA, *p=0.0039, 100 nM vs. Cont; *p=0.0029, 200 nM vs. Cont. (B) Western blots reveal the expression of the epithelial marker (E-cad) and mesenchymal markers (Vim and Snail) in Tri-PyMT cells following a 5 day treatment with BMH21 (100 nM) and CX5461 (20 nM). The numbers indicate the normalized intensity of the band according to the β-actin band of the sample. (C) Flow cytometry plots show the percentage of Epcam+/GFP + Tri PyMT cells treated with BMH21. GFP+/EpCam- Tri-PyMT cells were sorted and cultured in 3D to induce MET for 14 days. The gain of Epcam expression was analyzed by flow cytometry. n=4, One-way ANOVA, *p<0.0001, 100 nM vs. Cont; *p<0.0001, 200 nM vs. Cont.
-
Figure 4—source data 1
Raw western blot images of Figure 4B.
- https://cdn.elifesciences.org/articles/89486/elife-89486-fig4-data1-v1.zip
-
Figure 4—source data 2
Whole western blot images of Figure 4B with labels.
- https://cdn.elifesciences.org/articles/89486/elife-89486-fig4-data2-v1.pdf
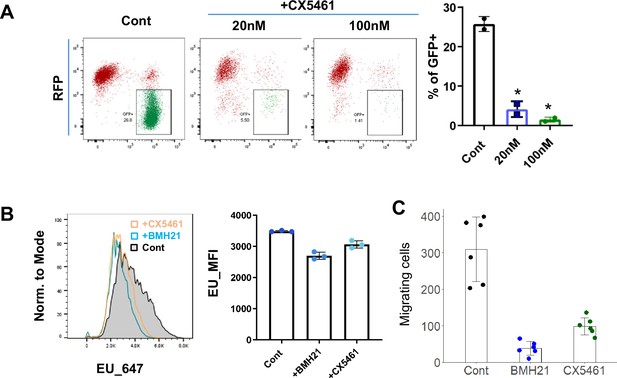
Ribosome biogenesis (RiBi)inhibition impairs RNA transcription activity and reduces the epithelial-to-mesenchymal transition (EMT) capability of tumor cells.
(A) Fluorescence switch in Tri-PyMT cells with CX5461 treatment. Flow cytometry plots exhibit the percentage of GFP + Tri PyMT cells after treatment with the Pol I inhibitor CX5461. Biological repeat, n=2, One-way ANOVA, *p=0.0015, 20 nM vs. Cont; *p=0.0011, 100 nM vs. Cont. (B) EU incorporation assay. Tri-PyMT cells (p10) were treated with RiBi inhibitors, BMH21 at 100 nM, or CX5461 at 20 nM, for 2 days. EU incorporation assay was performed and detected with click chemistry using Alexa A647-azide. Labeled cells were analyzed by flow cytometry (Left). The median fluorescence intensity (MFI) of EU staining of cells were quantified, n=3, One-way ANOVA, p=0.0001,+BMH21 vs. Cont; p=0.0032, +CX5461 vs. Cont. (C) Cell migration assay. Tri-PyMT cells (p7) were seeded in the inserts of the migration plate and treated with BMH21 (100 nM) or CX5461 (20 nM) for 5 days. Cell migration was induced by the FBS gradient for 24 hr. Migrating cells were counted from the bottom of the insert. n=6, One-way ANOVA, *p<0.001 BMH21 vs. Cont and CX5461 vs. Cont.
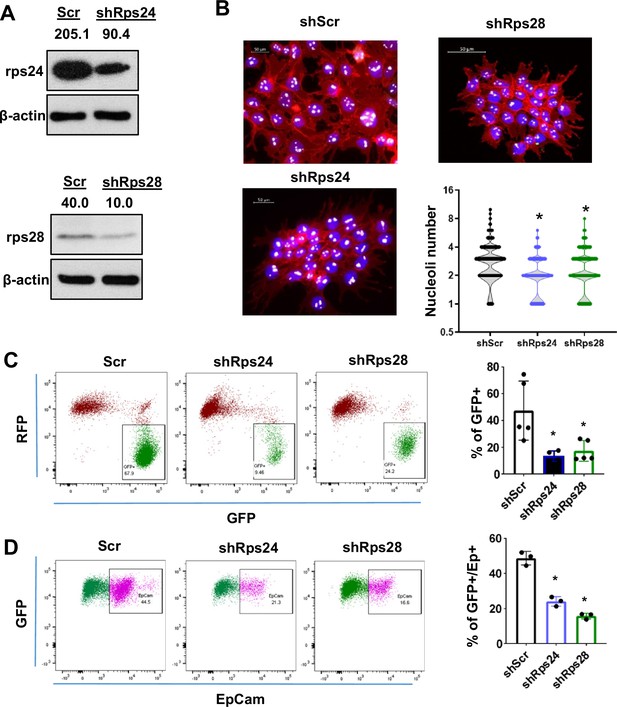
Ribosome biogenesis (RiBi) modulating by knocking down ribosome proteins reduces the epithelial-to-mesenchymal transition (EMT)/mesenchymal-to-epithelial transition (MET) capability of tumor cells.
(A) Western blots show the knockdown expression of Rps24 and Rps28 in targeted Tri-PyMT cells. The numbers indicate the relative intensity of the band normalizing to the β-actin band of the sample. (B) Impact on nucleoli numbers by Rps knockdown in RFP + Tri PyMT cells. Fluorescent images show the reduced number of nucleoli in Rps24 and Rps28 knockdown cells. n=623 cells (shScr), 490 cells (shRps24), 553 cells (shRps28). One-way ANOVA with Dunnett multiple comparisons, *p<0.0001 for shScr vs. shRps24 and shScr vs. shRps28. (C) Flow cytometry plots show the percentage of GFP + cells in Rps24 and Rps28 knockdown Tri-PyMT cells. 4 biological repeats, One-way ANOVA, *p=0.0085, shScr vs. shRps24, *p=0.0117, shScr vs. shRps28. (D) Flow cytometry plots show the Epcam+/GFP + cells in Rps24 or Rps28 knockdown Tri-PyMT cells. Three biological repeats, one-way ANOVA, *p=0.0055, shScr vs. shRps24, *p=0.0124, shScr vs. shRps28.
-
Figure 4—figure supplement 2—source data 1
Raw western blot of Figure 4—figure supplement 2A.
- https://cdn.elifesciences.org/articles/89486/elife-89486-fig4-figsupp2-data1-v1.zip
-
Figure 4—figure supplement 2—source data 2
Whole western blot of Figure 4—figure supplement 2A with labels.
- https://cdn.elifesciences.org/articles/89486/elife-89486-fig4-figsupp2-data2-v1.pdf
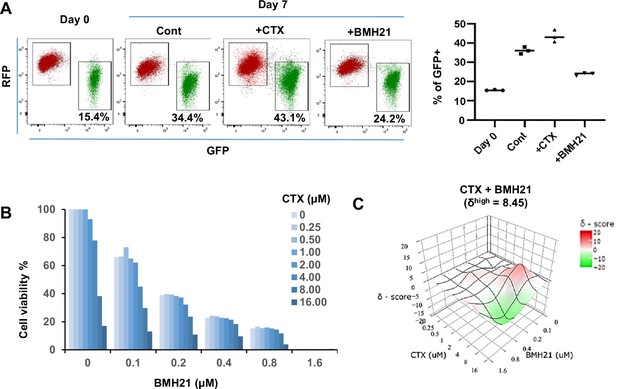
RNA Pol I inhibitor synergizes with chemo drug in vitro.
(A) Flow cytometry plots display the fluorescence switch of Tri-PyMT cells. Tri-PyMT cells (p5) were treated with cyclophosphamide (CTX, 2 μM), Pol I inhibitor (BMH21, 0.1 μM), or vehicle control for 7 days. Flow cytometry was performed to analyze the percentage of GFP + cells. n=3 wells/treatment, One way ANOVA, *p=0.0050, Cont vs. CTX; *p=0.0002, Cont vs. BMH21. (B) Cytotoxic assay of BMH21 and CTX treatments. Tri-PyMT cells were treated with serial concentrations of BMH21 (0, 0.1, 0.2, 0.4, 0.8, and 1.6 μM) in combination with serial concentrations of CTX (0, 0.25, 0.5, 1.0, 2.0, 4.0, 8.0, 16.0 μM). Bars represent the mean value of cell viabilities from duplicated treatments, n=2 wells/treatment. (C) Synergy plots of BMH21 and Cyclophosphamide (CTX). Synergic scores (δ) for each combination were calculated using the ZIP reference model in SynergyFinder 3.0. Deviations between observed and expected responses indicate synergy (red) for positive values and antagonism (green) for negative values.
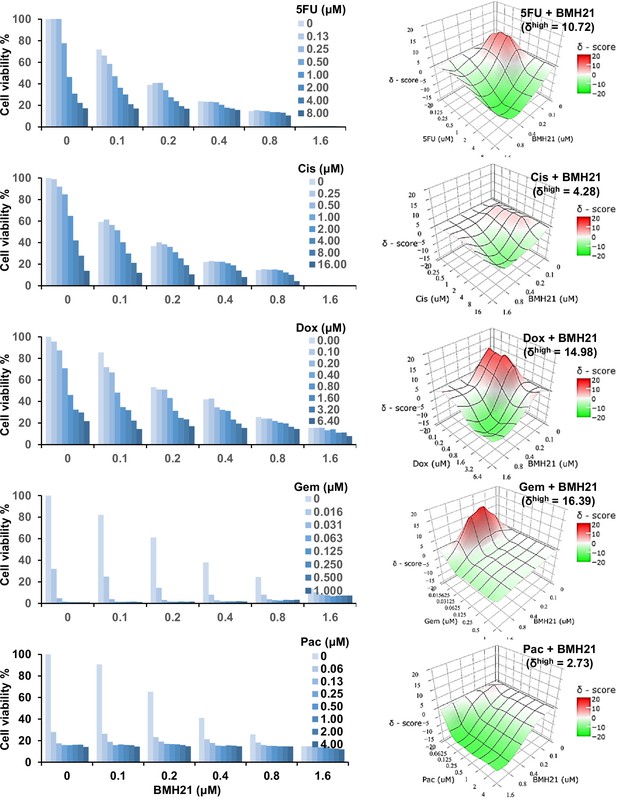
Combination therapy of RNA Pol I inhibitor and chemo drugs.
Left, Bar graphs display Tri-PyMT cell viability following combination treatment with BMH21 and chemotherapeutic drugs at various concentrations. Bars represent the mean value of duplicated treatments, n=2 wells/treatment. Right, Synergy plots of BMH21 and chemotherapeutic drugs. Synergistic scores (δ) for each combination were calculated using the ZIP model in SynergyFinder 3.0. The highest δ numbers among combinations are shown.
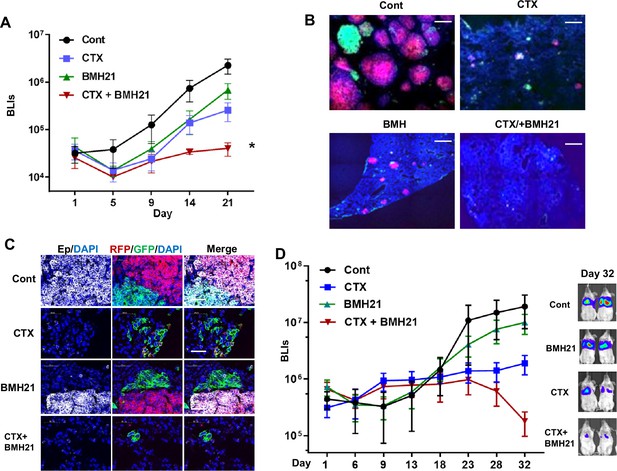
RNA Pol I inhibition synergizes with chemo drug in treating lung metastatic tumors.
(A–C) The same number of RFP + and GFP + Tri PyMT cells were injected in animals via tail vein. Animals were treated with cyclophosphamide (CTX) (100 mg/kg, once a week, i.p.), BMH21 (20 mg/kg, five times a week, i.p.), or both in combination. (A) Lung metastasis growth curves as measured by bioluminescent imaging (BLI). n=5, two-way ANOVA with Tukey’s test, *p=0.0154, Cont vs. CTX + BMH; *p=0.0151, BMH21 vs. CTX + BMH21; *p=0.0372, CTX vs. CTX + BMH21 at Day 21 post-inoculation. (B) Fluorescent images display RFP + and GFP + metastatic nodules in the lungs treated with CTX and BMH21. Cell nuclei were stained with DAPI. Scale bar: 200 μm. (C) Fluorescent images exhibit epithelial-to-mesenchymal transition (EMT) statuses of RFP + and GFP + metastases. Sections were stained with anti-EpCam antibody; cell nuclei were stained with DAPI. Scale bar: 50 μm. (D) Lung metastasis growth curves for LM2 model. Lung metastatic breast tumor cells (LM2 cells) were injected into animals via the tail vein. Animals were treated with CTX (100 mg/kg, once a week, i.p.), BMH21 (20 mg/kg, five times a week, i.p.), or both in combination. n=5, two-way ANOVA with Tukey’s test, *p=0.0404, Cont vs. CTX + BMH21; *p=0.0161, BMH21 vs. CTX + BMH21; *p=0.0187, CTX vs. CTX + BMH at Day 32 post-inoculation. Representative bioluminescent imaging (BLI) images of Day 32 were presented on the right.
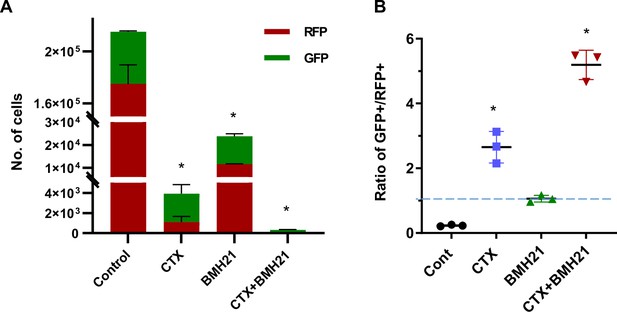
Differential impact of Pol I inhibitor and chemo drug on epithelial and mesenchymal Tri-PyMT cells in vivo.
The same number of RFP + and GFP + Tri PyMT cells were injected in animals via the tail vein. Animals were treated with cyclophosphamide (CTX) (100 mg/kg, once a week, i.p.), BMH21 (20 mg/kg, five times a week, i.p.), or both in combination. Number of RFP + and GFP + cells were quantified by flow cytometry on day 21 after inoculation. (A) Plots show the number of RFP + and GFP + Tri PyMT cells in the lung treated with CTX and/or BMH21. n=3 mice/treatment, One-way ANOVA, *p<0.0001 for Cont vs. CTX, Cont vs. BMH21 and Cont vs. CTX + BMH21. (B) The ratio of GFP + vs. RFP + cells in the lung treated with CTX and/or BMH21. n=3 mice/treatment, One-way ANOVA, *p=0.0001 for Cont vs. CTX; *p=0.0661 for Cont vs. BMH21 and *p<0.0001 for Cont vs. CTX + BMH21.

E-cadherin expression by LM2 cells in lung metastases.
qRT-PCR analysis of E-cadherin expression in LM2 cells isolated from lung metastases. LM2 cells in culture served as control. GAPDH served as the internal control for PCR. n=3 experiments, unpaired t-test, *p=0.0025.
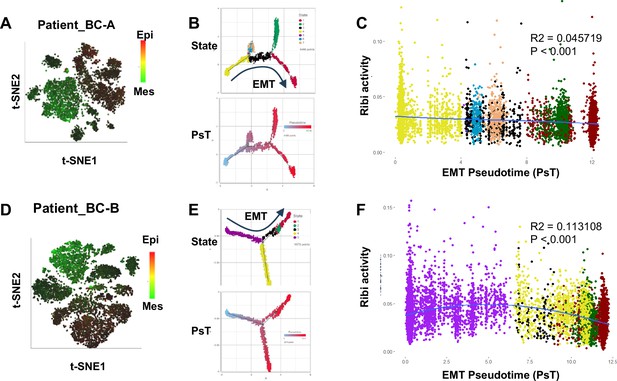
Correlation of ribosome biogenesis pathway and epithelial-to-mesenchymal transition (EMT) status in human breast cancer cells.
Single-nucleus cell RNA sequencing analysis of primary tumor cells from breast cancer patients (BC-A and BC-B, GEO database, GSE 198745). (A,D) The t-SNE plots show the heterogeneity in EMT statuses of tumor cells with AUC values of epithelial genes (in red) and mesenchymal genes (in green). (B,E) Cell trajectory analysis was performed with filtered EMT-related genes (EMTome genes) using the Monocle 2 model. Cell states were identified with the most epithelial cells as the root for the calculation of EMT pseudotime (PsT). (C,F) The scatter plot displays the correlation of ribosome biogenesis (RiBi) activity to EMT pseudotime. The polynomial regression line (order = 3) highlights the higher RiBi activity in cells toward epithelial phenotypes.
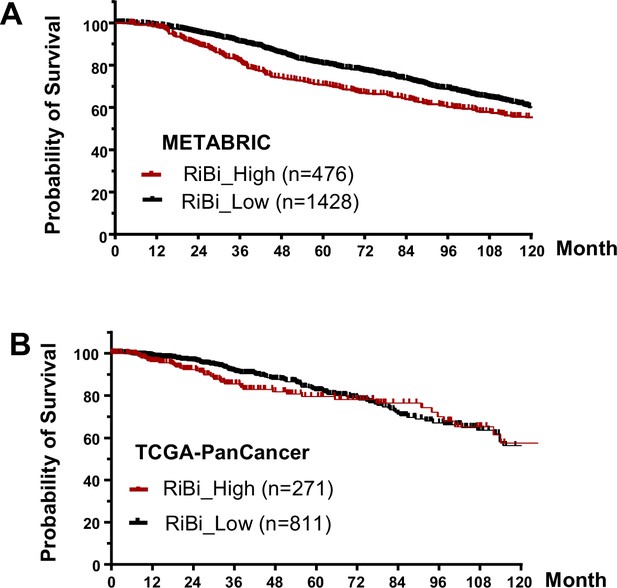
Survival curves of breast cancer patients with different ribosome biogenesis (RiBi) activities.
Gene expression data of METABRIC (A) and TCGA-PanCancer (B) was obtained from CBioPortal. Patients were grouped according to the average z-score of RiBi gene expression, RiBi High (>1) and RiBi Low (RiBi score <0.5). Upper, METABRIC dataset, Gehan-Breslow-Wilcoxon test <i>p-value = 0.0019. Lower, TCGA data, Gehan-Breslow-Wilcoxon test <i>p-value = 0.0225.
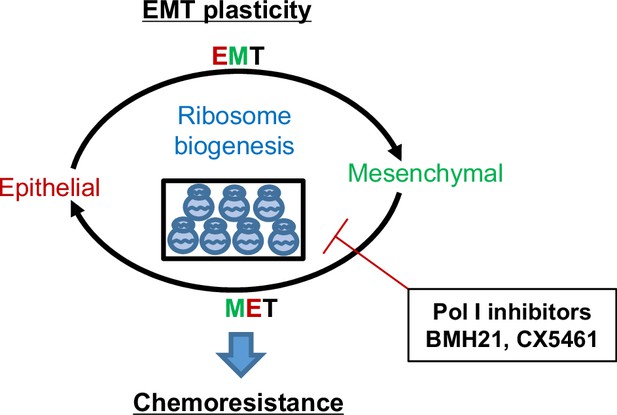
Working model of ribosome biogenesis in EMP plasticity.
Tumor cells exhibit plasticity by undergoing EMT (epithelial-mesenchymal transition) and MET (mesenchymal-epithelial transition), contributing to the development of resistance to chemotherapies. Elevated ribosome biogenesis is essential for maintaining this EMT plasticity. Inhibition of ribosome biogenesis by RNA Pol I inhibitors synergizes with chemotherapeutic drugs to overcome resistance development.