Mitochondrial genomes of Pleistocene megafauna retrieved from recent sediment layers of two Siberian lakes
eLife assessment
This work presents convincing evidence for the presence of wooly mammoth/rhinoceros ancient environmental DNA (aeDNA) far from the time likely to host living individuals: what is effectively a genetic version of a geological inclusion. These are important findings that will have ramifications for the interpretation and conclusions extracted from aeDNA more generally.
https://doi.org/10.7554/eLife.89992.3.sa0Important: Findings that have theoretical or practical implications beyond a single subfield
- Landmark
- Fundamental
- Important
- Valuable
- Useful
Convincing: Appropriate and validated methodology in line with current state-of-the-art
- Exceptional
- Compelling
- Convincing
- Solid
- Incomplete
- Inadequate
During the peer-review process the editor and reviewers write an eLife Assessment that summarises the significance of the findings reported in the article (on a scale ranging from landmark to useful) and the strength of the evidence (on a scale ranging from exceptional to inadequate). Learn more about eLife Assessments
Abstract
Ancient environmental DNA (aeDNA) from lake sediments has yielded remarkable insights for the reconstruction of past ecosystems, including suggestions of late survival of extinct species. However, translocation and lateral inflow of DNA in sediments can potentially distort the stratigraphic signal of the DNA. Using three different approaches on two short lake sediment cores of the Yamal peninsula, West Siberia, with ages spanning only the past hundreds of years, we detect DNA and identified mitochondrial genomes of multiple mammoth and woolly rhinoceros individuals—both species that have been extinct for thousands of years on the mainland. The occurrence of clearly identifiable aeDNA of extinct Pleistocene megafauna (e.g. >400 K reads in one core) throughout these two short subsurface cores, along with specificities of sedimentology and dating, confirm that processes acting on regional scales, such as extensive permafrost thawing, can influence the aeDNA record and should be accounted for in aeDNA paleoecology.
Introduction
Sedimentary deposits constitute highly valuable archives of past ecosystem changes as they contain dateable layers with organismic remains including ancient DNA (aDNA). Such remains are typically assumed to represent the ecosystem of the time around which the respective stratum was deposited. aDNA from sediments has yielded remarkable insights regarding paleoecology, phylogeography, and extirpation and extinction events of keystone taxa such as mammoths (Haile et al., 2009; Boessenkool et al., 2012; Graham et al., 2016; Murchie et al., 2021b). Based on such ancient environmental DNA (aeDNA), a recent study proposed that the woolly mammoth (Mammuthus primigenius) may have survived in Eurasia for much longer than previously assumed, as the authors retrieved mammoth DNA sequences in sediment layers that were approximately 4.6–7 thousand years (kyr) younger than the most recent mammoth fossils Wang et al., 2021; however, in response to this interpretation, Miller and Simpson, 2022 opined that these results may be more likely due to taphonomic processes leading to release of aeDNA from the remains of long-dead organisms from permafrost, where it is well preserved.
Conclusions derived from aeDNA isolated from sediment cores rely on the stratified nature of the remains in question and dating of the respective layer by radiometric methods. However, in theory, various physical and geochemical processes such as translocation of DNA through sediment strata (Haile et al., 2007), re-deposition of older sediment carrying DNA of extinct organisms (Arnold et al., 2011), and preservation bias (Boere et al., 2011) can distort the biological signal of aeDNA and thus bias the accuracy of allocation of taxa to specific time periods (Armbrecht et al., 2019). For lake sediments, deposited under aquatic conditions, studies have suggested that leaching is not a concern (Parducci et al., 2017), but it has been observed in soils and cave sediments (Haile et al., 2007). The question of obtaining last appearance dates of extinct taxa using aeDNA in dated sediment layers (Haile et al., 2009) is under discussion, but the surprisingly young records published so far still date to multiple thousands of years before present and thus lie within a timeframe of possible late survival.
Results and discussion
In 2019, we retrieved short subsurface sediment cores from two Arctic thermokarst lakes (LK-001 and LK-007, located approximately 5 km apart, over massive permafrost; Table 1; Dvornikov et al., 2016) on the Yamal peninsula, Siberia, to extract DNA and assess changes in mammal abundances in the Arctic over the past decades and centuries. From lake LK-001, we collected a secondary core which was sliced in the field at 1 cm steps for Pb210 radiometric dating, which indicated that the sediments at the top of this core were deposited recently, and that the core spanned the past few centuries (Appendix 1—table 7). The cores for DNA extraction were closed in the field immediately after retrieval and were then transported to the dedicated aDNA laboratories of the University of Konstanz, Germany. In this lab facility, established in 2020, no other samples from the Arctic or from any large mammals had been processed previously. From core LK-001, we isolated DNA and produced genomic double-stranded libraries from 23 samples, from 1.5 to 80 cm core depth (Supplement section 1), according to standard procedures. The core was opened and all subsequent steps until index PCR setup were carried out under customary aDNA laboratory conditions. In particular, the core opening facilities and the lab are located in buildings separated from the downstream molecular genetic analyses, the ventilation of the aDNA lab is based on a HEPA filter system and positive air pressure, and the lab is subjected to nightly UV radiation. Work in the lab is conducted under strict aDNA precautions, adhering to established aDNA protocols (Fulton and Shapiro, 2019). We enriched the libraries for mammalian DNA using a custom RNA bait panel produced from complete mitogenome sequences of 17 mammal species that currently or previously occurred in the Arctic (adapted from Murchie et al., 2021a). The enriched libraries were sequenced, and we mapped the sequences against a database of 73 mammal mitogenomes, followed by BLASTn alignment against the complete NCBI nt database. We thus retrieved mitogenomic sequences of mammals that were expected during the age range covered by the core (Appendix 1—table 9), for example, reindeer (Rangifer tarandus), Arctic lemming (Dicrostonyx torquatus), and hare (Lepus); however, throughout the entire core, there were abundant sequences of two species that have been extinct for several thousand years, that is mammoth (Mammuthus primigenius) and woolly rhinoceros (Coelodonta antiquitatis). Twenty-two of the 23 LK-001 libraries produced >1000 reads, each, assigned to Mammuthus, with read counts ranging from 2852 to 72,919 (mean 21,140±17,296). Negative controls (extraction and library blanks) did not produce any reads assigned to mammals. In the sample with the highest M. primigenius read counts (31.5 cm depth, dated to 81 years), the coverage of the reference mitogenome (NCBI accession NC_007596.2) was 95.3%, (434 (±213)-fold). Across all samples, 465,080 reads assigned to mammoth were produced, with 98.3% coverage (2762 to ±1176 fold). Read lengths ranged from 28 to 289 bp (mean 100±44 bp; Figure 1). The number of woolly mammoth reads decreased from lower samples towards the top of the core (Figure 1). Signatures of post-mortem DNA decay were comparably minor (Figure 1), with reference to an M. primigenius genome downloaded from NCBI (accession NC_007596.2), and mapping suggested that the sequences throughout the core originated from multiple individuals. Further analyses of the three libraries with the most mammoth reads using mixemt (Vohr et al., 2017) identified a number of mitochondrial haplogroups in the sequences from the core, suggesting that they originated from a multiple individuals (Figure 2). The haplogroups identified were known to occupy the region, and it seems likely the sequences reflect a history of mammoth occupation at the core site. Twelve of the 23 libraries produced >100 reads, each, assigned to woolly rhinoceros, with a total of 2737 reads and a cumulative coverage of 44% (assembled to NC_012681.1 2). Further analyses are mostly focused on the mammoth sequences as these occurred in substantially higher numbers.
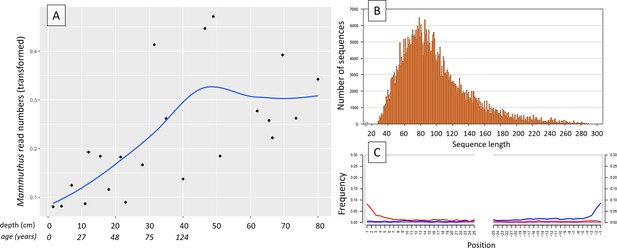
aDNA of Mammuthus in recent lake sediments.
(A) Read counts assigned to Mammuthus (square-root-transformed proportion of the respective number of raw reads per library) after hybridization capture enrichment of aeDNA of core LK-001 (shown are results of 22 libraries; one library was excluded as it did not produce any reads assigned to mammals); square-root transformation of percentage. Indicated are sample depths (in cm; 1.5–80 cm) and approximate ages as per 210Pb chronology (Appendix 1—table 7; to a maximum depth of 39.5 cm). The solid line indicates the general trend. Across the 22 libraries: (B) Fragment length distribution and (C) damage patterns (red indicates C-to-T transitions, blue G-to-A transitions. the Y-axis indicates the percentage of positions with a nucleotide change, the X-axis indicates the position along the fragment).
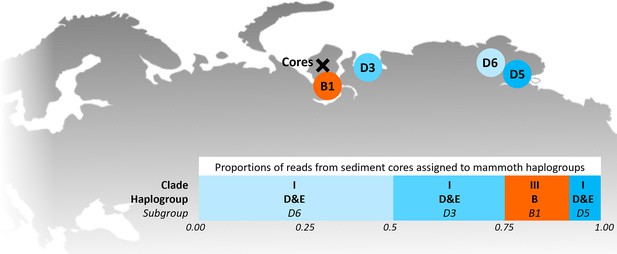
Locations of the sediment cores of the present study (Yamal peninsula, Siberia) and previously retrieved mammoth remains and their haplo(sub)groups (Appendix 1—table 6).
The bar chart indicates a maximum-likelihood estimate of the haplogroup proportions derived from the reads from the three sediment core libraries with the most mammoth reads.
Sediment cores retrieved from two lakes on the Yamal peninsula, Siberia.
Lake | Coordinates | m above sea level | Area | Water depth | Core length |
---|---|---|---|---|---|
LK-001 | 70°16'45.6" N, 68°53'02.8" E | 28 | 38 ha | 17 m | 80 cm |
LK-007 | 70°16'02.8" N, 68°59'35.7" E | 36 | 39 ha | 14 m | 75 cm |
As these results were entirely incongruent with the temporal occurrence of these two species, we employed several methods to confirm their validity, that is conventional PCR and Sanger sequencing of a mammoth cytochrome c oxidase subunit I (COI) fragment, mammal metabarcoding, and droplet digital PCR (ddPCR) of a mammoth cytochrome b fragment. We performed these analyses on core LK-001 and, to evaluate whether this is a locally isolated phenomenon, on a second short core, LK-007, from a nearby lake, which had been opened and processed under the same conditions indicated above to prevent contamination. Additionally, we screened the core LK-001 for plant macrofossils, of which three were sent for 14C AMS dating.
Conventional PCR and Sanger sequencing confirmed amplification of a COI fragment of M. primigenius. Mammal metabarcoding produced mammoth sequences (74 bp) in 13 samples of core LK-001 and 9 samples of core LK-007. In the LK-001 core, the highest M. primigenius read count occurred at 31.5 cm (2,992 reads), and in the LK-007 core at 26 cm M. primigenius (3,580 reads). ddPCR produced M. primigenius sequences in 14 samples of each core. Metabarcoding and ddPCR patterns across the cores were similar, although not completely congruent, as ddPCR appeared to be more sensitive (Appendix 1—figure 1). The dates retrieved by radiocarbon dating were not congruent with the initial age inference suggested by Pb210. While the lowest and topmost sample (with ages of 1547±228 and 339±79 uncal. yrs BP respectively) suggest relatively young ages and agree in their temporal succession, the middle sample, at 51 cm, yielded a radiocarbon age of 8677±132 years.
The mammoth was abundant throughout most of Eurasia during the Pleistocene, but populations declined at the end of the Pleistocene, with the species going extinct in the mid-Holocene (Nogués-Bravo et al., 2008). The youngest fossils from mainland Siberia have been dated to 9650 years (Stuart et al., 2002). The woolly rhinoceros was a cold-adapted megaherbivore, which was abundant from western Europe to north-east Siberia during the Middle to Late Pleistocene (Kahlke and Lacombat, 2008). This species was predominantly grazing, probably resorting to browsing only due to seasonal vegetation restrictions (Kahlke and Lacombat, 2008; Rey-Iglesia et al., 2021; Stefaniak et al., 2021). The reasons underlying the extinction of this species at ca 14 ka BP are not entirely clear, but it is largely attributed to sudden climate warming and subsequent habitat unsuitability due to vegetation changes (Stuart and Lister, 2012; Lord et al., 2020; Wang et al., 2021), likely coupled with human influence (Fordham et al., 2022), in the Bølling-Allerød interstadial warming.
The present data, which implies frequent and abundant Pleistocene megafaunal DNA throughout a sediment core deposited in the lake over the past centuries suggests that physical processes, rather than presence of live organisms, are responsible for the recovery of this DNA. While not in itself fully conclusive, our data suggests the source of the DNA of Pleistocene mammals from older permafrost deposits, either from a carcass or from sedimentary materials carrying the DNA. The numbers of mammoth sequences increased with depth downcore, with comparably low abundances over the most recent 11 cm of the core (aged <30 years), pointing to a decrease of input of this DNA through time. The apparently rather limited extent of aDNA damage in the mammoth sequences suggests that the source of this DNA has been preserved exceptionally well, which also suggests an origin from permafrost, and the specific dynamics of thawing and re-deposition of material in the study area offer an explanation.
Here, the active thermokarst likely began during the climatic optimum of the Holocene (9000–3,000 BC; Savvichev et al., 2021). The LK-001 and LK-007 lake basins are interbedded mainly into the IVth marine plain, formed in the Kazantsevskaya, Marine Isotopic Stage 5. The permafrost of these lake catchments was formed no earlier than 70—60 kyr BP after the Kazantsevskaya transgression in more sub-aerial conditions and with mean annual temperatures 6 to 7 °C lower than modern temperatures (Baulin et al., 1981). As this area was under coastal-marine conditions for a long period, these lake basins may be paleo-marine remnants, or they were formed later as a result of thermokarst over the segregated or tabular (massive) ground ice during the Holocene climatic optimum (Kachurin, 1961). The area is also subject to abrupt permafrost thaw (thermo-denudation), resulting, for example, in the formation of retrogressive-thaw slumps (thermocirques) and the transport of a large amounts of thawed terrestrial material into the lake water (Dvornikov et al., 2018). Such abrupt permafrost thaw processes normally appear adjacent to lakes and can form specific geomorphological elements, that is, thermo-terraces (Kizyakov et al., 2006) within lake catchments and lakes; they are normally polycyclic processes appearing due to the extension of a seasonally thawed layer (active layer) up to the top of massive ice and more favorable thermal conditions within the existing forms (Leibman and Kizyakov, 2007; Kokelj et al., 2009). Traces of past thermo-denudation can be observed within both lake catchments. In catchments of five neighboring lakes, large retrogressive-thaw slumps appeared in recent years (2012–2013) accompanied by the thaw and lateral transport of modern and Late Pleistocene deposits into lakes. Additionally or alternatively, thermo-erosion of upper geomorphological levels and transport via stream networks could transport ancient material into the modern lacustrine sediments. However, the two studied lakes are headwater lakes (with outlet, no apparent inlet) and this option can only be considered in terms of small thermo-erosional valleys within the catchments.
An alternative mechanism for the redistribution of Late Pleistocene material in the sediments is related to subcap methane emission (bubbling) from degrading permafrost beneath the lake bottom. In-lake bubbling can be observed in a circum-Arctic scale: in North-East Siberia, Alaska and Canada. This is common especially in lakes with a depth exceeding two meters, which do not freeze entirely up to the bottom in winter, leading to the formation of a talik (a layer of year-round unfrozen ground that lies in permafrost area). The expansion of the talik may further trigger subcap methane emission, which can reach 40–70 kg yr–1 of pure (94–100%) methane in neighboring lakes (Kazantsev et al., 2020). The constant methane seepage does not allow ice to be formed in winter (whereas the normal winter ice thickness is approximately 1.5 m) and can potentially disturb the stratigraphy of lake sediments. Additionally, dramatic emissions of methane can form craters in terrestrial and lacustrine environments (Dvornikov et al., 2019). In this case, Late Pleistocene sediments will well be re-distributed within the water-body and the entire stratigraphy will be mixed.
In the case reported here, the simultaneous finding of a Pb210 chronology indicating recent sediment deposition and of plant macrofossils that dated to >8000 years BP in a sample from 36.5 cm, suggest lateral input of ancient material, including the mammalian DNA, putatively related to permafrost thawing processes. Numerous studies on aDNA discussed possible leaching through sedimentary strata of the DNA itself, yet it was typically considered not an issue as most of these studies were conducted under stable permafrost or similar conditions (e.g. Hansen et al., 2006; Haile et al., 2007, but see Andersen et al., 2012). Permafrost thawing and re-deposition of material adds a new dimension to this problem of temporal interpretation. The fact that we retrieved mammoth sequences from both cores of two lakes located approximately 5 km apart suggests that this is not an isolated phenomenon but occurs on a regional or even larger scale. Given the wide spread of abrupt permafrost thaw processes in the Arctic plains (West Siberia, Taimyr, Chukotka, Alaska, Canadian Arctic; Kizyakov et al., 2006 and references therein), the phenomena of disturbed stratigraphy of lacustrine sediments can potentially be observed at a pan-Arctic scale. While this indicates that temporal interpretation of sedimentary aeDNA records should be exercised with caution, our study also demonstrates that a careful evaluation of available information on the site and ecosystem in conjunction with the use of independent dating techniques can uncover incongruencies. This is more difficult in older time periods, where artefactual stratigraphies caused by equivalent processes acting for a limited time will not be detected as easily as in our case of long extinct species. The same applies to extant taxa or those which have undergone extinction or extirpation more recently, the presence of which cannot as easily be excluded as in the current example. However, we suggest that the inclusion of robust dating techniques and knowledge of local geophysical processes can provide good arguments to evaluate the reliability of aeDNA records.
Materials and methods
Field sites, DNA isolation and hybridization capture enrichment
Request a detailed protocolIn 2019, sediment cores (6 cm diameter; Table 1) were retrieved from two lakes (LK-001 and LK-007, respectively, Table 1) on the Yamal peninsula, Siberia, using a UWITEC piston corer (UWITEC, Mondsee, Austria). The lakes were located approximately 5 km apart. The cores were transported to the aDNA laboratories of the University of Konstanz, Germany; from lake LK-001, a secondary core was taken which was sliced in the field at 1 cm steps for radiometric dating from 0 to 39.5 cm depth, performed at the Environmental Radioactivity Research Centre, University of Liverpool (Supplement section 9). Additional 14 C dating of three specimens of plant remains, extracted at 36.5 cm, 51 cm, and 74 cm, was performed (Supplement section 9).
Sedimentary DNA was isolated from 23 samples of core LK-001 and from 16 samples of core LK-007 using commercially available kits with modified protocols (Supplement section 1). The extracts of core LK-001 were subjected to library preparation for capture enrichment. Enrichment probes were designed from mitogenomes of 17 herbivorous mammal species that currently or previously occurred in the Arctic (Appendix 1—table 2) and few lichen sequences (Appendix 1—table 3). Genomic libraries were produced according to Li et al., 2013 with some modifications (Seeber et al., 2019; Seeber et al., 2023; Li et al., 2023; Supplement section 2). Filtered reads were mapped to mammalian mitogenomes, followed by BLASTn alignment against the complete NCBI nucleotide database and subsequent metagenomic analyses using MEGAN (Huson et al., 2016). Reads assigned to Mammuthus were mapped to a complete M. primigenius reference mitogenome (NCBI accession NC_007596.2); reads assigned to Coelodonta antiquitatis were mapped to the NCBI reference mitogenome NC_012681.1 2. The reads mapped to mammoth from the top three libraries were assigned to haplogroup using mixemt (https://github.com/svohr/mixemt, Copy archieved at Vohr, 2024) with a custom-made representative panel of 15 mammoth mitogenomes (Figure 2; Appendix 1—table 6).
Conventional PCR, mammal metabarcoding, and ddPCR
Request a detailed protocolBased on the enriched fragments with the highest coverage, PCR primers specific to M. primigenius were designed using Geneious Prime 2022.1.1 (Kearse et al., 2012), that is mamm801 (5`- CCCATGCAGGAGCTTCTGTAGA-3`) and mamm800r (5`-GACATAGCTGGAGGTTTTATGT-3`) to produce a 121 bp amplicon of the CO1 gene. The specific PCR conditions are described in Supplement section 6. Mammal metabarcoding PCRs were performed on 21 DNA extracts of core LK-001 and 27 samples of core LK-007, with eight independent replicates, each. Each batch of PCRs included one non-template control. Established metabarcoding primers were used (Giguet-Covex et al., 2014), and human blocking primers (Garcés-Pastor et al., 2021) were included. PCR conditions are described in the supplementary material. Sequencing was performed on an Illumina NovaSeq platform, with 2x150 reads. The raw data were processed as described in the supplement. We used the sample LK-001_66.5 of core LK-001 which had produced one of the highest Mammuthus read counts after enrichment. The specific target amplified by the primer pair were used to design a probe (5`-GGATACTCCTGCAAGGTGAAGTG-3`). With this probe, ddPCR was performed with 24 extracts of core LK-001 and with 30 extracts of core LK-007, with three replicates, each (Supplement section 8).
Appendix 1
Sampling and DNA isolation
The sediment cores were subsampled according to procedures described by Epp et al., 2019, extracting approximately 1–2 g sediment from the center of the core at close depth intervals (Appendix 1—table 1). Sampling was performed in a window-less cold room at 4 °C, adhering to standard aDNA precautions. DNA was extracted using two highly similar kits. Sample depths and respective extraction protocols for both cores are listed below (Appendix 1—table 1).
Sample depths and extraction protocols.
(a) LK-001 | (b) LK-007 | ||
---|---|---|---|
Sample depth (cm) | Extraction protocol* | Sample depth (cm) | Extraction protocol* |
1.5 | PowerLyzer | 2.0 | PowerLyzer |
4.0 | 4.0 | PowerSoil Pro | |
7.0 | 6.0 | PowerLyzer | |
11.0 | 8.0 | PowerSoil Pro | |
12.0 | 10.0 | PowerLyzer | |
15,5 | 12.0 | PowerSoil Pro | |
18.0 | 14.0 | PowerSoil Pro | |
21.5 | 18.0 | PowerLyzer | |
23.0 | 20. | PowerSoil Pro | |
28.0 | 24.0 | PowerLyzer | |
31.5 | 26.0 | PowerSoil Pro | |
35.0 | 30.0 | PowerSoil Pro | |
40.0 | 32.0 | PowerSoil Pro | |
44.0 | 36.0 | PowerLyzer | |
46.5 | 38.0 | PowerSoil Pro | |
49.0 | 42.0 | PowerSoil Pro | |
51.0 | 44.0 | PowerLyzer | |
62.0 | 48.0 | PowerLyzer | |
65.5 | 50.0 | PowerLyzer | |
66.5 | 54.0 | PowerLyzer | |
69.5 | 56.0 | PowerLyzer | |
73.5 | 60.0 | PowerLyzer | |
80.0 | 62.0 | PowerSoil Pro | |
64.0 | PowerLyzer | ||
66.0 | PowerSoil Pro | ||
68.0 | PowerLyzer | ||
70.0 | PowerSoil Pro |
-
*
Kits from Qiagen (Hilden, Germany).
The PowerLyzer DNA extraction was performed according to a previous study (Alsos et al., 2020), with some modifications (Appendix 1—table 2).
PowerLyzer DNA extraction.
Day 1: |
|
Day 2: |
|
PowerSoil Pro DNA extraction was performed as follows: | |
Day 1: |
|
Day 2: |
|
Preparation of genomic libraries for hybridization capture
Double-stranded libraries were prepared from genomic DNA according to the protocol below and were visualized by gel electrophoresis. Each batch of libraries (N=12) was processed with a library blank to control for contamination during library prep. Indexing was performed using individual combinations of P5/P7 index primers.
Library preparation.
End repair | per library | |
---|---|---|
Mix following components in a sterile low-binding PCR tube | ||
NEBNext End Repair Buffer (10 X) | 5 µL | |
NEBNext End Repair Enzyme Mix | 2.5 µL | |
genomic DNA | 42.5 µL | |
Incubate in a thermal cycler for 30 mins at 20 °C. Purify using QIAquick/MinElute PCR purification kit. Elution: add 32 µl buffer EB and incubate at 37 °C for 5 min before spinning down the DNA at 13,000 rpm for 1 min. | ||
Adapter ligation | ||
Mix following components in a sterile low-binding PCR tube | ||
Quick Ligation Reaction Buffer (10 X) | 10 µL | |
nuclease-free water | 4 µL | |
P5/P7 adapter mix (50 µM stock) | 1 µL | |
DNA as purified in step above | 30 µL | |
Quick T4 DNA ligase | 4.8 µL | |
*final adapter concentrations for ancient samples should be between 0.25–0.5 µM. **it is vital to add ligase after mixing DNA with adaptors. | ||
Incubate for 15 min at 25 °C; purify using a QIAquick/MinElute PCR purification kit. Elution: 42 µL buffer EB and incubate at 37 ºC for 5 min before spinning down the DNA at 13,000 rpm for 1 min. | ||
Fill-In Reaction | ||
Add the following reagents into a low-binding PCR tube | ||
ThermoPol Reaction Buffer | 5 µL | |
dNTPs (10 mM) | 2 µL | |
Bst DNA polymerase | 3 µL | |
DNA as eluted above | 40 µL | |
Incubate: 20 mins at 65 °C 20 mins at 80 °C No purification is needed after this step. |
Indexing PCR.
Reagent | µL | |
---|---|---|
H2O | 3.45 | |
Platinum Hifi Taq Buffer 10 X (Thermo Fisher Scientific) | 2.50 | |
dNTPs (25 mM) | 0.25 | |
bovine serum albumin (New England Biolabs) | 1.00 | |
MgSO4 (50 mM) | 1.00 | |
Platinum HiFi (5 U/µL; Thermo Fisher Scientific) | 0.20 | |
total | 8.40 | |
Index P5 (10 µM) | 0.80 | |
Index P7 (10 µM) | 0.80 | |
template DNA | 15.00 | |
Thermocycling | ||
°C | t | |
94 | 1 min | |
94 | 15 s | 8 cycles |
60 | 20 s | |
68 | 60 s | |
68 | 3 min | |
20 | store |
Thereafter, libraries were pooled (four libraries/pool) and were purified using a MinElute PCR purification kit (Qiagen; elution: 2x20 µL). Concentrations were measured using a Qubit device (broad-range assay; Thermo Fisher Scientific).
Hybridization capture enrichment and sequencing
To design RNA oligonucleotide baits for hybridization capture, we compiled the mitochondrial genome sequences of 17 selected mammal species (comprising 282,168 nucleotides [nt]; Appendix 1—table 2) and ITS-1 and ITS-2 sequences of Cladonia rangiferina (8546 and 4750 nt, respectively; Appendix 1—table 3). The compiled mitochondrial sequences were submitted to Daicel Arbor Biosciences for custom design of 70 bp baits at threefold tiling, collapsed at 99% identity and 85% overlap, resulting in 9339 unique baits (747,120 nt).
Each purified library pool containing four libraries was subjected to one hybridization capture reaction, apart from libraries produced from extraction blanks (N=15), library blanks (N=15), and PCR non-template controls (N=30), all of which were pooled in one reaction due to the minute DNA concentrations (mostly below detection range).
Hybridization capture was performed according to the MYbaits protocol v. 5.00 (Daicel Arbor Biosciences) with the following modifications: a total amount of 150 ng baits per capture reaction was used, and library pools were incubated for hybridization with the baits at 58 °C for 24 hr. Post-capture, the enriched libraries were removed from the beads and were amplified similarly to the PCR protocol used for the indexing PCR and with primers IS5/IS6 (Illumina, San Diego, CA, USA) and 14 cycles. PCR products were then purified using the MinElute PCR Purification Kit (Qiagen), and the final library concentration was measured on an Agilent Bioanalyzer. For sequencing, the enriched libraries were pooled at equal concentrations, and two library pools (produced from 57 and 110 libraries, respectively) were sequenced on an Illumina NovaSeq platform using three Novaseq SP 2x150 flow cells.
Mitogenome templates of 17 mammal species for design of RNA baits.
Order | Species | NCBI accession | # of baits |
---|---|---|---|
Artiodactyla | Bison bison | NC_012346.1 | 446 |
Bos primigenius | NC_020746.1 | 456 | |
Saiga tatarica | NC_013996.1 | 538 | |
Ovis canadensis | NC_015889.1 | 522 | |
Ovibos moschatus | NC_020631.1 | 542 | |
Cervus elaphus | NC_007704.2 | 523 | |
Rangifer tarandus | NC_007703.1 | 526 | |
Alces alces | NC_020677.1 | 520 | |
Camelus ferus | NC_009629.2 | 583 | |
Perissodactyla | Equus przewalskii | NC_024030.1 | 575 |
Coelodonta antiquitatis | NC_012681.1 | 571 | |
Lagomorpha | Lepus arcticus | NC_044769.1 | 586 |
Ochotona collaris | NC_003033.1 | 591 | |
Proboscidea | Mammuthus primigenius | NC_007596.2 | 588 |
Eulipotyphla | Sorex tundrensis | NC_025327.1 | 584 |
Rodentia | Castor canadensis | NC_033912.1 | 584 |
Dicrostonyx torquatus | NC_034646.1 | 575 | |
9,310 |
Cladonia rangiferina sequences for RNA bait design (shown are the NCBI accessions).
ITS-1 |
---|
MN756840.1; DQ394367.1; JQ695919.1; MK179592.1; KP031549.1; KP001202.1; AF458306.1; KT792792.1; MK811970.1; KT792788.1; MK508944.1; GU169225.1; KP001197.1; KP001201.1; MK812260.1; MK811708.1; KY119381.1; MK508952.1; KT792789.1; EU266113.1; KY266884.1; KP001192.1; KP001190.1; JQ695918.1; KT792790.1; JQ695920.1; KP001191.1; AF458307.1; KP001200.1; MK508943.1; MK812460.1; MK508937.1; KT792791.1 resulting in 23 baits |
ITS-2 |
KT792789.1; KP001190.1; AF458307.1; JQ695919.1; MK179592.1; DQ394367.1; KP001194.1; KP001193.1; KY266884.1; KP001199.1; KP001192.1; MK300750.1; MN756487.1; MK508937.1; KP001200.1; MK812460.1; MK508943.1; KP001191.1; KP031549.1; KP001202.1; AF458306.1; MK811970.1; KY119381.1; KP001198.1; MK812260.1; MK811708.1; GU169225.1; JQ695918.1; KP001201.1 resulting in 6 baits |
Bioinformatics of capture enrichment data
Adapter sequences were removed and sequences were filtered using leeHom with the default ancient DNA settings (Renaud et al., 2014). Duplicates were removed, and filtered reads were mapped against a database of 73 mammal mitogenomes (Appendix 1—table 4) using BWA v. 0.7.17 (Li and Durbin, 2009) and were blastn-aligned against the complete NCBI Genbank nucleotide database (Benson et al., 2009; retrieved March 14 2022) with a maximum e-value of 0.01. All blast output files were processed using MEGAN Community Edition 6.19.8 using a weighted LCA algorithm (Huson et al., 2016). aDNA degradation was examined using mapDamage 2.2.1 (Ginolhac et al., 2011) against a reference genome of M. primigenius (NC_007596.2). The respective command lines are shown in Appendix 1—table 5. The reads blast-assigned to M. primigenius were mapped to the complete M. primigenius reference mitogenome to produce consensus sequences using Geneious Prime 2023.0.1 (Kearse et al., 2012).
Reference mitogenomes used for mapping.
NC_020679.1 | Antilocapra americana | NC_018783.1 | Equus ovodovi |
NC_012346.1 | Bison bison | HM118851.1 | Equus hemionus |
NC_020746.1 | Saiga tatarica | MK982180.1 | Equus asinus |
NC_013996.1 | Bos primigenius | NC_012681.1 | Coelodonta antiquitatis |
NC_015889.1 | Ovis canadensis | NC_007596.2 | Mammuthus primigenius |
NC_020630.1 | Oreamnos americanus | FR691686.1 | Castor fiber |
NC_020631.1 | Ovibos moschatus | NC_033912.1 | Castor canadensis |
NC_027233.1 | Bison priscus | NC_034313.1 | Dicrostonyx groenlandicus |
NC_009629.2 | Camelus ferus | NC_034646.1 | Dicrostonyx torquatus |
KR822422.1 | Camelops cf. hesternus | JN181159.1 | Peromyscus leucopus |
NC_013836.1 | Cervus elaphus xanthopygus | NC_006853.1 | Bos taurus |
NC_007704.2 | Cervus elaphus | KM093871.1 | Capra hircus |
NC_013840.1 | Cervus elaphus yarkandensis | NC_015241.1 | Microtus fortis fortis |
KP405229.1 | Alces alces cameloides | KP200876.1 | Vulpes lagopus |
NC_020677.1 | Alces alces | HM236180.1 | Ovis aries |
NC_020729.1 | Odocoileus hemionus | KT448275.1 | Canis latrans |
NC_015247.1 | Odocoileus virginianus | JN632610.1 | Capreolus capreolus |
NC_007703.1 | Rangifer tarandus | KJ681493.1 | Capreolus pygargus |
KY987554.1 | Platygonus compressus | JN632629.1 | Dama dama |
NC_002008.4 | Canis lupus familiaris | KM982549.1 | Lynx lynx |
NC_009686.1 | Canis lupus lupus | KP202265.1 | Panthera pardus |
NC_013445.1 | Cuon alpinus | NC_026460.1 | Rhinolophus macrotis |
NC_026529.1 | Vulpes lagopus | Y07726.1 | Ceratotherium simum |
NC_028302.1 | Panthera leo | NC_005089.1 | Mus musculus |
NC_022842.1 | Panthera onca | AM711900.1 | Meles meles |
NC_010642.1 | Panthera tigris | KM091450.1 | Mustela erminea |
NC_014456.1 | Lynx rufus | NC_005358.1 | Ochotona princeps |
NC_020642.1 | Martes americana | NC_012095.1 | Sus scrofa domesticus |
NC_020641.1 | Neovison vison | DQ480489.1 | Canis lupus familiaris |
NC_024942.1 | Mustela nigripes | NC_020670.1 | Crocuta crocuta |
NC_020664.1 | Martes pennanti | NC_011116.1 | Arctodus simus |
NC_020639.1 | Mustela nivalis | NC_027963.1 | Sorex araneus |
NC_009685.1 | Gulo gulo | NC_025327.1 | Sorex tundrensis |
NC_011112.1 | Ursus spelaeus | KJ397607.1 | Lepus arcticus |
NC_003426.1 | Ursus americanus | NC_001640.1 | Equus caballus |
NC_003427.1 | Ursus arctos | NC_024030.1 | Equus przewalskii |
NC_003428.1 | Ursus maritimus |
Command lines and software used for initial mapping, processing, and taxonomic assignment.
adapter trimming, filtering, and merging: leeHom Renaud et al., 2014: | src/leeHom -t 120 –ancientdna –auto -fq1 file_R1.fastq.gz -fq2 file_R2.fastq.gz -fqo leehom_out |
mapping: BWA Li and Durbin, 2009 against 75 mammal mitogenomes Appendix 1—table 3: | bwa index reference_mitogenomes.fasta bwa aln reference_mitogenomes.fasta leehom_out.fq.gz -l 16,000 n 0.01 -O 2 -o 2 t 8>bwa_out.sai bwa samse reference_mitogenomes.fasta bwa_out.sai leehom_out.fq.gz>bwa_out.sam samtools view -q ≥ 30 S -b bwa_out.sam>bwa_out.bam |
remove duplicates: samtools Li and Durbin, 2009 | samtools collate -o bwa_out_col.bam bwa_out.bam samtools fixmate -m bwa_out_col.bam bwa_out_fixmate.bam samtools sort -o bwa_out_pos.bam bwa_out_fixmate.bam samtools markdup bwa_out_pos.bam bwa_out_mark.bam samtools fastq bwa_out_mark.bam>bwa_out.fastq |
fastq to fasta | sed -n ‘1~4 s/^@/>/p;2~4 p’ bwa_out.fastq>bwa_out.fasta |
#alignment: blastn Altschul et al., 1990 blastn -db ncbi_nt -query bwa_out.fasta -evalue 0.01 -out blastn_out.fasta | |
aDNA damage: mapDamage Ginolhac et al., 2011 | bwa index reference.fasta bwa aln reference.fasta sample.fasta >sample.sai bwa samse referecne.fasta sample.sai sample.fasta >sample.sam samtools view -q 25 S -b sample.sam >sample.bam mapDamage -i sample.bam -r reference.fasta mapDamage -d results_ sample/ -y 0.1 --plot-only mapDamage -i sample.bam -r reference.fasta –rescale mapDamage -d results_sample / --forward --stats-only -v -r reference.fasta |
Mammoth mitogenome haplogroup identification
The sequences mapping to mammoth from the libraries with the most mammoth reads were remapped to the mammoth reference (NC_007596) with minimap2 (https://github.com/lh3/minimap2, Copy archieved at Li, 2024) to generate a bam file. A panel of 15 mitogenome reference sequences (see Appendix 1—table 5) exemplifying the diversity of mammoths was used to identify variants representative of each haplogroup. In cases where there are multiple reference genomes for a haplogroup, the consensus variants unique to the haplogroup were used except for haplogroups B and D&E where haplosubgroups were also identified. mixemt (Vohr et al., 2017, https://github.com/svohr/mixemt) was then used to detect haplogroups present in the core and estimate the mixture proportions of these haplogroups, using only mapped reads with a map quality of >30 and with the requirement that 40% of the unique defining variants of each haplogroup must be observed to say it is present.
Reference mammoth mitogenomes used for panel.
Clade | Haplogroup | Subgroup | Accession numbers | Proportion of reads assigned to haplogroup |
---|---|---|---|---|
II | A | EU153451, EU153450 | - | |
III | B | B0 | KX027526, KX027531 | - |
B1 | KX027526 | 0.1615 | ||
B2 | KX027531 | - | ||
I | C | KX027498, KX027565, KX027567, JF912200, KX027499, KX027502 | - | |
I | D&E | D0 | DQ316067, EU153454, EU153447, EU153456, EU153449, EU153455, EU153446 | - |
D1 | DQ316067 | - | ||
D2 | EU153454 | - | ||
D3 | EU153447 | 0.2790 | ||
D4 | EU153456 | - | ||
D5 | EU153449 | 0.0765 | ||
D6 | EU153455 | 0.4829 | ||
D7 | EU153446 | - | ||
I | F | KX027503, KX027512, NC_015529, KX027511, KX027548, KX027547, KX027556, KX027559, KX027550 | - | |
Krestovka mammoth | K | PRJEB42269 (European Nucleotide Archive) | - | |
Conventional PCR | ||||
94 °C | 2 min | |||
94 °C | 30 s | 55 cycles | ||
54 °C | 30 s | |||
68 °C | 20 s | |||
68 °C | 1 min |
Conventional PCR
The PCR reaction mix comprised 17.05 µL H2O, 0.2 µL Platinum Taq DNA-Polymerase High Fidelity (Thermo Fisher Scientific, Waltham, MA, USA), 2.5 µL 10 X reaction buffer, 0.25 µL dNTPs (25 mM), 1 µL bovine serum albumin (20 mg/mL; New England Biolabs, Ipswitch, MA, USA), 1 µL MgSO4 (50 mM), 1 µL of each primer (mamm801 and mamm800r; 10 µM), and 1 µL DNA extract. Thermocycling was performed as shown in Appendix 1—table 9.
Amplification products were visualized by gel electrophoresis and were purified from excised gel bands using a NucleoSpin Gel and PCR Clean-up kit (Macherey-Nagel, Düren, Germany), followed by Sanger sequencing.
Mammal metabarcoding
We used the primers MamP007F and MamP007R (Giguet-Covex et al., 2014) and blockers (Garcés-Pastor et al., 2021), with the following conditions:
Conventional PCR.
Reagent | µL | |
---|---|---|
H2O | 14.85 | |
Platinum Hifi Taq Buffer 10 X (Thermo Fisher Scientific) | 2.5 | |
bovine serum albumin (New England Biolabs) | 0.2 | |
MgSO4 (50 mM) | 1.00 | |
dNTPs (25 mM) | 0.25 | |
Platinum HiFi (5 U/µL; Thermo Fisher Scientific) | 0.20 | |
Blocking primer R (1 µM) | 0.5 | |
Blocking primer F (1 µM) | 0.5 | |
total | 20.00 | |
template DNA | 3.00 | |
Thermocycling | ||
°C | t | |
94 | 5 min | |
94 | 30 s | 40 cycles |
50 | 30 s | |
68 | 30 s | |
68 | 10 min | |
RT | store |
PCR products were purified using a MinElute kit (Qiagen). Sequencing was performed on an Illumina NovaSeq platform, with 2x150 reads. The raw data were processed as described below.
Bioinformatics of mammal metabarcoding data
The obtained datasets by Illumina Sequencing were then processed as follows:
Conventional PCR.
Load data | obi import --quality-sanger file_R1.fastq reads1 obi import --quality-sanger file_R2.fastq reads2 |
Import tags | obi import --ngsfilter-input taglist.txt ngsfile |
Align paired-end reads | obi alignpairedend -R reads2 reads1 aligned_reads |
Grep entries whose mode are alignment | obi grep -a mode:alignment aligned_reads good_sequences |
Assign alignments to individual PCRs | obi ngsfilter -t ngsfile -u unidentified_sequences good_sequences identified_sequences |
Filter out sequences | obi grep -p "sequence[’score']>50" identified_sequences identified_sequences_filtered obi grep -p "sequence[’score_norm']>0.9" identified_sequences_filtered identified_sequences_filtered_adj |
Dereplicate Sequences | obi uniq -m sample identified_sequences_filtered_adj dereplicated_sequences_filtered |
Keep only useful tags | obi annotate -k COUNT -k MERGED_sample dereplicated_sequences_filtered cleaned_metadata_sequences |
Discard sequences that are shorter than 60 bp (based on primer pair) | obi grep -p "len(sequence) ≥ 60 and sequence['COUNT'] ≥ 10" cleaned_metadata_sequences denoised_sequences |
Clean the sequences from PCR/sequencing errors | obi clean -s MERGED_sample -r 0.05 H denoised_sequences cleaned_sequences |
Load database | cp STD_MAM_1.dat.gz ~/edna_LauraB/master/mammalia/database/ |
Import it into DMS | obi import /data/scc/edna/LauraBa/master/mammalia/database/STD_MAM_1.dat.gz database_mam obi import --embl EMBL embl_refs |
Download the taxonomy | wget https://ftp.ncbi.nlm.nih.gov/pub/taxonomy/taxdump.tar.gz |
Import the taxonomy in the DMS | obi import --taxdump /data/scc/edna/LauraBa/master/mammalia/taxdump.tar.gz taxonomy/my_tax |
Cleaning the database with in silico PCR | obi ecopcr -e 3 l 50 L 150 F CGAGAAGACCCTATGGAGCT -R CCGAGGTCRCCCCAACC --taxonomy taxonomy/my_tax embl_refs mam_refs |
Filter sequences | obi grep --require-rank=species --require-rank=genus --require-rank=family --taxonomy taxonomy/my_tax mam_refs mam_refs_clean |
Dereplicate identical sequences | obi uniq --taxonomy taxonomy/my_tax mam_refs_clean mam_refs_uniq |
Add taxid at the family level | obi grep --require-rank=family --taxonomy taxonomy/my_tax mam_refs_uniq |
Build the reference database | obi build_ref_db -t 0.97 --taxonomy taxonomy/my_tax mam_refs_uniq_clean mam_db_97 |
Assign each sequence to a taxon | obi ecotag -m 0.97 --taxonomy taxonomy/my_tax -R mam_db_97 cleaned_sequences assigned_sequences |
Align the sequences | obi align -t 0.95 assigned_sequences aligned_assigned_sequences |
Export tables for downstream data analysis | obi grep -A SCIENTIFIC_NAME assigned_sequences assigned_for_metabR |
Output two tables required by metabaR | obi annotate -k MERGED_sample assigned_for_metabR assigned_for_metabR_reads_tableobi export --tab-output --output-na-string 0 assigned_for_metabR_reads_table >mam_reads_01.txtobi annotate --taxonomy taxonomy/my_tax \ --with-taxon-at-rank superkingdom \ --with-taxon-at-rank kingdom \ --with-taxon-at-rank phylum \ --with-taxon-at-rank subphylum \ --with-taxon-at-rank class \ --with-taxon-at-rank subclass \ --with-taxon-at-rank order \ --with-taxon-at-rank suborder \ --with-taxon-at-rank infraorder \ --with-taxon-at-rank superfamily \ --with-taxon-at-rank family \ --with-taxon-at-rank genus \ --with-taxon-at-rank species \ --with-taxon-at-rank subspecies \ assigned_for_metabR assigned_for_metabR_taxInfo obi annotate \ -k BEST_IDENTITY -k TAXID -k SCIENTIFIC_NAME -k COUNT -k seq_length \ -k superkingdom_name \ -k kingdom_name \ -k phylum_name \ -k subphylum_name \ -k class_name \ -k subclass_name \ -k order_name \ -k suborder_name \ -k infraorder_name \ -k superfamily_name \ -k family_name \ -k genus_name \ -k species_name \ assigned_for_metabR_taxInfo assigned_for_metabR_motus obi export --tab-output assigned_for_metabR_motus >mam_motus_01.txt |
Further processing of the data sets was done using RStudio. | |
Editing files for metabar | reads<- dt_reads %>% dplyr::select(-c("DEFINITION", "NUC_SEQ"))%>% as.data.frame() %>% janitor::row_to_names(row_number = 897, remove_rows_above = FALSE, remove_row = TRUE) %>% mutate_if(is.integer,as.numeric) |
Assign name to first column | reads <- cbind(rownames(reads),reads) rownames(reads) <- NULL colnames(reads) <- c(names(reads)) colnames(reads)(1) <- "pcr_id" |
Edit the names of the column | reads$pcr_id = strsplit(reads$pcr_id,"[.]") reads$pcr_id = sapply(reads$pcr_id, function(x) x[length(x)]) rownames(reads)<- reads$pcr_id |
Organizing the MOTUs table | motus<- dplyr::select(dt_motus, 'ID', 'NUC_SEQ', 'COUNT','BEST_IDENTITY', 'TAXID', 'SCIENTIFIC_NAME', ’superkingdom_name', ’species_name', 'class_name', 'order_name', 'family_name', 'genus_name', 'kingdom_name', 'phylum_name', ’subphylum_name', ’subclass_name', ’suborder_name') names(motus)[names(motus) == 'NUC_SEQ'] <- ’sequence' |
Droplet digital PCR (ddPCR)
ddPCR was performed using a Bio-Rad QX200 system (Bio-Rad Hercules, CA, USA) using the following conditions, and data were produced and analyzed as per the standard instructions of the manufacturer. Droplets were generated by using 21 µL of the PCR mixture and 70 µL ddPCR Droplet Reader Oil (Bio-Rad) according to the manufacturer’s instructions. The final volume for PCR amplification was 40 µL and was carried out in a C1000 Touch Thermo cycler (Bio-Rad). The products were analyzed using a QX200 Droplet Reader (Bio-Rad); the threshold was set manually to 3000.
Conventional PCR.
Reagent | µL | |
---|---|---|
ddPCR Supermix for probes | 11 | |
H2O (DEPC) | 6.8 | |
20 x Target-Primers/Probe (FAM) | 1.1 | |
20 x Target-Primers/Probe (HEX) | 1.1 | |
total | 20 | |
template DNA | 2.0 | |
Thermocycling | ||
°C | t | |
95 | 10 min | |
94 | 30 sec | 40 cycles |
50 | 30 sec | |
60 | 30 sec |
Sediment dating
The dating sections of core LK-001 (Appendix 1—table 13) were dried to constant weight at 65 °C and were then analyzed for 210Pb, 226Ra, and 137Cs by direct gamma assay at the Liverpool University Environmental Radioactivity Laboratory using Ortec HPGe GWL series well-type coaxial low background intrinsic germanium detectors. This allowed dating to a depth of 39.5 cm (Appendix 1—table 13).
210Pb chronology of Yamal lake sediment core LK-001.
Depth | Chronology | Sedimentation Rate | ||||
---|---|---|---|---|---|---|
Date | Age | |||||
cm | g cm–2 | AD | y | ± | g cm–2 y–1 | cm y–1 |
0.00 | 0.0 | 2019 | 0 | 0 | ||
0.25 | 0.2 | 2018 | 1 | 1 | 0.34 | 0.37 |
1.25 | 1.1 | 2016 | 3 | 2 | 0.34 | 0.37 |
2.25 | 1.9 | 2013 | 6 | 2 | 0.34 | 0.37 |
3.25 | 2.8 | 2010 | 9 | 2 | 0.34 | 0.37 |
4.25 | 3.8 | 2008 | 11 | 2 | 0.34 | 0.37 |
5.25 | 4.7 | 2005 | 14 | 2 | 0.34 | 0.37 |
6.25 | 5.7 | 2002 | 17 | 2 | 0.34 | 0.37 |
7.25 | 6.7 | 1999 | 20 | 3 | 0.36 | 0.37 |
8.25 | 7.6 | 1996 | 23 | 3 | 0.39 | 0.41 |
10.25 | 9.7 | 1992 | 27 | 3 | 0.49 | 0.47 |
12.25 | 11.8 | 1988 | 31 | 4 | 0.53 | 0.49 |
14.25 | 14.0 | 1984 | 35 | 4 | 0.55 | 0.51 |
14.75 | 14.5 | 1983 | 36 | 4 | 0.55 | 0.51 |
15.50 | 15.4 | 1981 | 38 | 4 | 0.55 | 0.51 |
16.50 | 16.5 | 1980 | 39 | 4 | 0.55 | 0.51 |
17.50 | 17.6 | 1978 | 41 | 4 | 0.55 | 0.51 |
18.50 | 18.6 | 1976 | 43 | 4 | 0.55 | 0.51 |
20.50 | 20.7 | 1971 | 48 | 5 | 0.55 | 0.51 |
22.50 | 22.7 | 1968 | 51 | 6 | 0.55 | 0.51 |
23.50 | 23.7 | 1966 | 53 | 6 | 0.55 | 0.51 |
24.50 | 24.8 | 1964 | 55 | 6 | 0.55 | 0.51 |
25.50 | 25.9 | 1962 | 57 | 7 | 0.44 | 0.42 |
26.50 | 27.0 | 1961 | 58 | 7 | 0.37 | 0.36 |
27.50 | 28.0 | 1957 | 62 | 8 | 0.30 | 0.29 |
28.50 | 29.0 | 1952 | 67 | 9 | 0.28 | 0.22 |
29.50 | 30.2 | 1949 | 70 | 10 | 0.23 | 0.19 |
31.50 | 32.6 | 1938 | 81 | 10 | 0.23 | 0.19 |
33.50 | 34.7 | 1933 | 86 | 10 | 0.23 | 0.19 |
35.50 | 36.9 | 1927 | 92 | 11 | 0.23 | 0.19 |
37.50 | 39.1 | 1913 | 106 | 13 | 0.23 | 0.19 |
39.50 | 41.4 | 1895 | 124 | 17 | 0.23 | 0.19 |
Core LK-001 was additionally dated using radiocarbon. We collected plant remains throughout the core by first using a scalpel to cut 1 cm thick of bulk sediment at desired depths, followed by washing the sediment chunks with distilled water and filtering using 190 µm mesh sieves. We then collected leaves of deciduous trees and seeds, which were placed in glass vials and dried under a fume hood before sending to the Micadas (Alfred Wegener Institute, Bremerhaven, Germany) for radiocarbon dating. Sample preparation and measurement methodologies at the Micadas were performed as described previously (Mollenhauer et al., 2021). We sent three samples that had sufficient plant materials for dating and retained two dates after removing an inaccurate date caused by high inbuilt age from plant remains (Appendix 1—table 14).
Radiocarbon dating results.
Sample label | F14C | ± (abs) | Age (y) | ± (y) | Weight (µg C) | Comment |
---|---|---|---|---|---|---|
LK-001_36.5 cm | 0.9595 | 0.0095 | 332 | 79 | 35 | |
LK-001_51 cm | 0.3396 | 0.0056 | 8677 | 132 | 148 | Off; lateral input |
LK-001_74 cm | 0.8248 | 0.0234 | 1547 | 228 | 13 |
Mammal read numbers
The numbers of reads assigned to all mammals are indicated in Appendix 1—table 15 (core LK-001 only; hybridization capture experiment).
Numbers of sequences assigned to mammals (core LK-001).
depth | |||||||||||||||||||||||
---|---|---|---|---|---|---|---|---|---|---|---|---|---|---|---|---|---|---|---|---|---|---|---|
sum | 1.5 | 4.0 | 7.0 | 11.0 | 12.0 | 15.5 | 18.0 | 21.5 | 23.0 | 28.0 | 31.5 | 35.0 | 40.0 | 46.5 | 49.0 | 51.0 | 62.0 | 65.5 | 66.5 | 69.5 | 73.5 | 80.0 | |
Mammuthus primigenius | 19,640 | 191 | 162 | 524 | 290 | 1205 | 605 | 194 | 666 | 277 | 800 | 3697 | 305 | 1188 | 1374 | 1923 | 631 | 674 | 519 | 1110 | 1083 | 814 | 1408 |
Rangifer tarandus | 18,055 | 253 | 63 | - | 34 | 328 | 688 | 644 | 1816 | 545 | 902 | 2186 | 1240 | 1571 | 1574 | 86 | 284 | 2714 | 56 | 345 | 991 | 114 | 1,621 |
Dicrostonyx torquatus | 16,870 | 211 | 112 | 34 | 111 | 1211 | 614 | 271 | 1035 | 522 | 1049 | 850 | 1298 | 314 | 761 | 193 | 318 | 656 | 200 | 2763 | 1011 | 1408 | 1928 |
Lepus | 6371 | 148 | 134 | 105 | 23 | 584 | 245 | 40 | 369 | 336 | 630 | 382 | 231 | 404 | 799 | 285 | 141 | 116 | 126 | 352 | 330 | 293 | 298 |
Coelodonta antiquitatis | 2737 | 33 | - | - | 28 | 119 | 153 | 347 | 55 | - | 97 | 128 | 467 | 298 | 141 | 27 | 103 | 152 | - | 250 | 144 | 4 | 191 |
Homo sapiens | 387 | - | - | - | - | - | - | 38 | 217 | - | - | 23 | - | 25 | - | 69 | - | 15 | - | - | - | - | - |
Ovibos moschatus moschatus | 145 | - | 69 | - | - | - | - | - | - | - | 38 | - | - | - | 38 | - | - | - | - | - | - | - | - |
Castor fiber | 135 | - | - | - | - | 18 | - | - | - | - | 15 | - | - | - | 27 | 51 | - | 6 | 1 | - | - | - | 17 |
Bos | 105 | - | - | - | - | - | - | - | - | - | - | - | - | - | - | 105 | - | - | - | - | - | - | - |
Cervinae | 92 | - | - | - | - | - | - | - | 33 | - | - | - | - | 31 | 28 | - | - | - | - | - | - | - | - |
Saiga tatarica | 91 | - | - | - | - | - | - | - | - | - | 57 | - | 34 | - | - | - | - | - | - | - | - | - | - |
Sus scrofa cristatus | 85 | - | - | - | - | - | - | - | 34 | - | - | - | - | - | - | 24 | - | - | - | - | 27 | - | - |
Ochotona | 74 | - | - | - | - | - | - | - | - | - | - | - | - | - | - | - | - | - | 14 | - | - | - | 60 |
Ovis aries musimon | 70 | - | - | - | - | 70 | - | - | - | - | - | - | - | - | - | - | - | - | - | - | - | - | - |
Bison | 54 | - | - | - | - | 24 | - | 30 | - | - | - | - | - | - | - | - | - | - | - | - | - | - | - |
Crocuta crocuta | 53 | - | - | - | - | - | - | - | - | - | - | - | - | - | - | - | - | - | - | 53 | - | - | - |
Chrotopterus auritus | 43 | - | - | - | - | - | 43 | - | - | - | - | - | - | - | - | - | - | - | - | - | - | - | - |
Sorex tundrensis | 32 | - | - | - | - | - | - | - | - | - | - | - | - | - | - | - | 32 | - | - | - | - | - | - |
Equus | 32 | - | - | - | - | - | - | - | - | - | - | - | - | - | - | - | - | - | 7 | - | - | - | 25 |
Peromyscus maniculatus bairdii | 29 | - | - | - | - | - | - | - | - | - | - | - | - | - | 29 | - | - | - | - | - | - | - | - |
Murinae | 28 | - | - | - | - | - | - | - | - | - | - | - | - | - | - | - | - | - | - | - | - | 28 | - |
Capra | 21 | - | - | - | - | - | - | - | - | - | - | - | - | - | - | - | 21 | - | - | - | - | - | - |
Myopus schisticolor | 18 | - | - | - | - | - | - | - | 18 | - | - | - | - | - | - | - | - | - | - | - | - | - | - |
Lemmus trimucronatus | 16 | 16 | - | - | - | - | - | - | - | - | - | - | - | - | - | - | - | - | - | - | - | - | - |
Data availability
Sequence data of the hybridization capture were made available as an NCBI BioProject (PRJNA1082062).
-
NCBI BioProjectID PRJNA1082062. Mitochondrial genomes of Pleistocene megafauna retrieved from recent sediment layers of two Siberian lakes.
References
-
Basic local alignment search toolJournal of Molecular Biology 215:403–410.https://doi.org/10.1016/S0022-2836(05)80360-2
-
Meta-barcoding of “dirt” DNA from soil reflects vertebrate biodiversityMolecular Ecology 21:1966–1979.https://doi.org/10.1111/j.1365-294X.2011.05261.x
-
BookMain stages of Permafrost development in the North-east of Western part of USSR and Western SiberiaIn: Dubikov GI, Baulin VV, editors. History of Development of Permafrost in Eurasia: On the Examples of Separate Regions. Nauka. pp. 41–60.
-
Geodatabase and WebGIS project for long-term permafrost monitoring at the Vaskiny Dachi Research Station, Yamal, RussiaPolarforschung 85:107–115.
-
Gas‐emission craters of the Yamal and Gydan peninsulas: A proposed mechanism for lake genesis and development of permafrost landscapesPermafrost and Periglacial Processes 30:146–162.https://doi.org/10.1002/ppp.2014
-
Sampling and extraction of ancient DNA from sedimentsMethods in Molecular Biology 1963:31–44.https://doi.org/10.1007/978-1-4939-9176-1_5
-
Setting up an ancient DNA laboratoryMethods in Molecular Biology 1963:1–13.https://doi.org/10.1007/978-1-4939-9176-1_1
-
mapDamage: testing for damage patterns in ancient DNA sequencesBioinformatics 27:2153–2155.https://doi.org/10.1093/bioinformatics/btr347
-
Ancient DNA chronology within sediment deposits: are paleobiological reconstructions possible and is DNA leaching a factor?Molecular Biology and Evolution 24:982–989.https://doi.org/10.1093/molbev/msm016
-
MEGAN Community edition - interactive exploration and analysis of large-scale microbiome sequencing dataPLOS Computational Biology 12:e1004957.https://doi.org/10.1371/journal.pcbi.1004957
-
Destructive relief-forming processes at the coasts of the Arctic plains with tabular ground iceKriosf Zemli 10:79–89.
-
Origin and polycyclic behaviour of tundra thaw slumps, Mackenzie Delta region, Northwest Territories, CanadaPermafrost and Periglacial Processes 20:173–184.https://doi.org/10.1002/ppp.642
-
Capturing protein-coding genes across highly divergent speciesBioTechniques 54:321–326.https://doi.org/10.2144/000114039
-
Standard operation procedures and performance of the MICADAS radiocarbon laboratory at Alfred Wegener Institute (AWI), GermanyNuclear Instruments and Methods in Physics Research Section B 496:45–51.https://doi.org/10.1016/j.nimb.2021.03.016
-
Ancient plant DNA in lake sedimentsThe New Phytologist 214:924–942.https://doi.org/10.1111/nph.14470
-
leeHom: adaptor trimming and merging for Illumina sequencing readsNucleic Acids Research 42:e141.https://doi.org/10.1093/nar/gku699
-
Late Pleistocene paleoecology and phylogeography of woolly rhinocerosesQuaternary Science Reviews 263:106993.https://doi.org/10.1016/j.quascirev.2021.106993
-
Terrestrial mammal surveillance using hybridization capture of environmental DNA from African waterholesMolecular Ecology Resources 19:1486–1496.https://doi.org/10.1111/1755-0998.13069
-
A phylogenetic approach for haplotype analysis of sequence data from complex mitochondrial mixturesForensic Science International. Genetics 30:93–105.https://doi.org/10.1016/j.fsigen.2017.05.007
Article and author information
Author details
Funding
Deutsche Forschungsgemeinschaft (EP-98/3-1)
- Laura S Epp
Biodiversa+ (BiodivScen ERA-Net COFUND)
- Laura S Epp
The funders had no role in study design, data collection and interpretation, or the decision to submit the work for publication.
Acknowledgements
This research was funded through the 2017–2018 Belmont Forum and BiodivERsA joint call for research proposals, under the BiodivScen ERA-Net COFUND program, and with the funding organizations Deutsche Forschungsgemeinschaft (DFG grant EP-98/3–1 to LSE), Agence Nationale de la Recherche (ANR), Research Council of Norway (NFR), the Swedish Research Council for Environment, Agricultural Sciences and Spatial Planning (Formas), Academy of Finland, National Science Foundation (NSF) and the Natural Sciences and Engineering Research Council of Canada (NSERC-CRSNG). Field work was enabled through a grant of the Young Scholar Fund of the University of Konstanz to LSE. YD was supported by the RUDN University Strategic Academic Leadership Program. Expedition logistics were supported by the Department of Science and Innovations of Yamalo-Nenets Autonomous Okrug and the Non-profit organization «Russian Center of Arctic Exploration». We thank PD Dr. Elena Marinova for assistance and discussions concerning dating of plant macrofossils and Patrick Bartolin for assistance in the wet lab.
Version history
- Preprint posted:
- Sent for peer review:
- Reviewed Preprint version 1:
- Reviewed Preprint version 2:
- Version of Record published:
Cite all versions
You can cite all versions using the DOI https://doi.org/10.7554/eLife.89992. This DOI represents all versions, and will always resolve to the latest one.
Copyright
© 2023, Seeber et al.
This article is distributed under the terms of the Creative Commons Attribution License, which permits unrestricted use and redistribution provided that the original author and source are credited.
Metrics
-
- 1,515
- views
-
- 156
- downloads
-
- 3
- citations
Views, downloads and citations are aggregated across all versions of this paper published by eLife.
Download links
Downloads (link to download the article as PDF)
Open citations (links to open the citations from this article in various online reference manager services)
Cite this article (links to download the citations from this article in formats compatible with various reference manager tools)
Further reading
-
- Genetics and Genomics
An unprecedented amount of SARS-CoV-2 data has been accumulated compared with previous infectious diseases, enabling insights into its evolutionary process and more thorough analyses. This study investigates SARS-CoV-2 features as it evolved to evaluate its infectivity. We examined viral sequences and identified the polarity of amino acids in the receptor binding motif (RBM) region. We detected an increased frequency of amino acid substitutions to lysine (K) and arginine (R) in variants of concern (VOCs). As the virus evolved to Omicron, commonly occurring mutations became fixed components of the new viral sequence. Furthermore, at specific positions of VOCs, only one type of amino acid substitution and a notable absence of mutations at D467 were detected. We found that the binding affinity of SARS-CoV-2 lineages to the ACE2 receptor was impacted by amino acid substitutions. Based on our discoveries, we developed APESS, an evaluation model evaluating infectivity from biochemical and mutational properties. In silico evaluation using real-world sequences and in vitro viral entry assays validated the accuracy of APESS and our discoveries. Using Machine Learning, we predicted mutations that had the potential to become more prominent. We created AIVE, a web-based system, accessible at https://ai-ve.org to provide infectivity measurements of mutations entered by users. Ultimately, we established a clear link between specific viral properties and increased infectivity, enhancing our understanding of SARS-CoV-2 and enabling more accurate predictions of the virus.
-
- Cell Biology
- Genetics and Genomics
Cells react to stress by triggering response pathways, leading to extensive alterations in the transcriptome to restore cellular homeostasis. The role of RNA metabolism in shaping the cellular response to stress is vital, yet the global changes in RNA stability under these conditions remain unclear. In this work, we employ direct RNA sequencing with nanopores, enhanced by 5ʹ end adapter ligation, to comprehensively interrogate the human transcriptome at single-molecule and -nucleotide resolution. By developing a statistical framework to identify robust RNA length variations in nanopore data, we find that cellular stress induces prevalent 5ʹ end RNA decay that is coupled to translation and ribosome occupancy. Unlike typical RNA decay models in normal conditions, we show that stress-induced RNA decay is dependent on XRN1 but does not depend on deadenylation or decapping. We observed that RNAs undergoing decay are predominantly enriched in the stress granule transcriptome while inhibition of stress granule formation via genetic ablation of G3BP1 and G3BP2 rescues RNA length. Our findings reveal RNA decay as a key component of RNA metabolism upon cellular stress that is dependent on stress granule formation.