Targeting sex determination to suppress mosquito populations
Figures
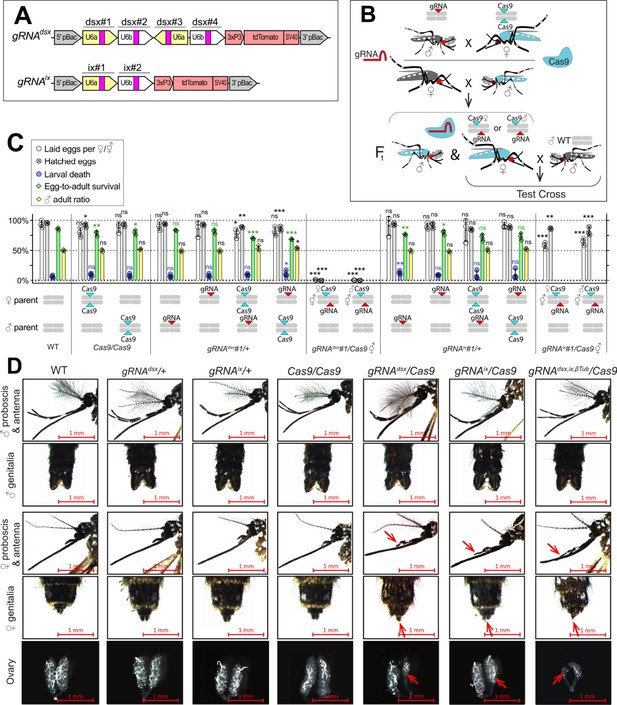
CRISPR/Cas9-mediated disruption of dsx or ix affects female mosquito morphology and fecundity.
(A) Schematic map of gRNA genetic constructs. The gRNAdsx and gRNAix constructs harbor a 3xP3-tdTomato marker and multiple gRNAs guiding Cas9 to the female-specific doublesex (dsx) gene or the female-active intersex (ix) gene, respectively. (B) A schematic of the genetic cross between the homozygous Cas9 and hemizygous gRNAdsx/+ or gRNAix/+ mosquitoes to generate trans-hemizygous F1 females (♀’s). Reciprocal genetic crosses were established and two types of trans-hemizygous F1 ♀’s were generated: gRNA/+; ♀Cas9/+ ♀’s inherited a maternal Cas9; and gRNA/+; ♂Cas9/+ ♀’s inherited a paternal Cas9. Then, both trans-hemizygous ♀’s were crossed to wild-type (WT) males (♂’s), and their fecundity was assessed. A comparison of the fecundity and male ratio of trans-hemizygous, hemizygous Cas9 or gRNA ♀’s to those of WT ♀’s. The bar plot shows means and one standard deviation (± SD) over triple biological replicates (n = 3). The data presentsone transgenic strain of each construct, gRNAdsx#1 and gRNAix#1, as all strains induced similar results (all data can be found in Supplementary file 1c). (D) All gRNAdsx#1/+; Cas9/+ and gRNAix#1/+; Cas9/+ trans-hemizygous ♀ mosquitoes exhibited male-specific features (red arrows in D), had reduced fecundity, and were transformed into intersexes (⚥’s). Statistical significance of mean differences was estimated using a two-sided Student’s t-test with equal variance (ns: p ≥ 0.05, *p < 0.05, **p < 0.01, and ***p < 0.001). Source data are provided in Supplementary file 1.

Changes in morphological structures induced by disruption of targeted genes.
External and internal structures were measured in mosquitoes of each genotype and sex. The trans-hemizygous mosquitoes harboring the maternal Cas9 (Cas9♀) were used for all measurements. The plot shows means ± standard deviation (SD) over 15 mosquitoes (n = 15) for each morphological structure. All data can be found in Supplementary file 1b. Statistical significance of mean differences was estimated using a two-sided Student’s t-test with equal variance (ns: p ≥ 0.05, *p < 0.05, **p < 0.01, and ***p < 0.001). Source data are provided in Supplementary file 1.

Induced mutations at the targeted sequences in dsx and ix.
The gene sequences targeted by gRNAs were Polymerase Chain Reaction (PCR) amplified from precision-guided sterile insect technique (pgSIT) mosquitoes and Sanger sequenced. Good quality of Sanger read chromatogram indicates the presence of a single consistent temple sequence (no cleave) at a targeted region, for example gRNAdsx#3 (A) or gRNAis#2 (B). An active CRISPR/gRNA-mediated cleavage at a particular DNA target results in re-ligation of cut fragments leading to the origin of diverse mutation-bearing alleles. These induced mutations are localized around the gRNA cleavage site and result in the drop of Sanger read quality (cleavage) as indicated for gRNAdsx#1, gRNAdsx#2, gRNAdsx#4, (A) and gRNAis#1 (B).

Ae. aegypti dsx or ix disruption does not result in significant alterations in ear anatomy, wing beat frequency (WBF), and male phonotactic behaviors.
Immunohistochemistry and microanatomic comparison of mosquito Johnston’s Orgran (cup-like structure) with chordotonal neurons responsible for auditory transduction in females (♀’s, A) or males (♂’s, B) among different genotypes. Female JO’s contained far fewer neurons than males across all genotypes, and distributions of synaptic punctate assessed by Anti-Synapsin (3C11, in green) were restricted to the somata, as opposed to the male-type distribution observable in the gap between the cilia and somata tracked by anti-horseradish peroxidase (HRP, in red). WBFs are different between Ae. aegypti ♀’s and ♂’s, and are shown on separate plots, (C) and (D), respectively. Each point represents the estimated frequency of a single fly-by (aka. the frequency of sound produced by one event of a mosquito flying past the microphone). WBF box plots indicate the median (middle bar), and 25% and 75% quartiles (lower/upper boundaries of box). The average ♀ WBF is 468 Hz. No significant differences were observed between ♀ groups, or between ♂ groups, regardless of the genotypes tested (analysis of variance [ANOVA] on ranks, p > 0.05). The male phonotaxis assay was conducted, in which individual flying ♂’s showed attractive or no response (scored as 1 or 0, respectively) toward the female-specific WBF produced for 30 s by a speaker (E). Three batches (aka. 3 replicate groups) of at least 10 ♂’s were examined for each genotype. ♂’s across all genotypes exhibited robust responses to sound. The point plot shows the mean (middle bar) and one standard deviation (± SD) for the proportion of ♂’s responding to the auditory stimulus (n=3). No significant differences were observed across groups (Chi-squared test, p > 0.05).
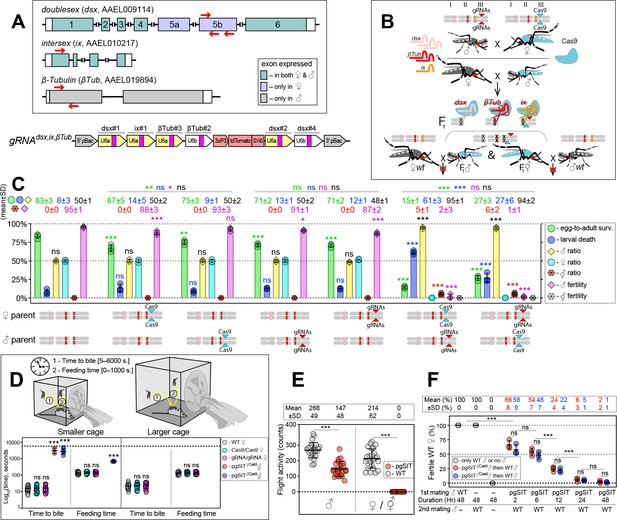
The precision-guided sterile insect technique (pgSIT) cross results in nearly complete female lethality and male sterility.
(A) Schematic maps of targeted genes (box) and gRNAdsx,ix,βTub construct. Red arrows show relative locations of gRNA target sequences (box). gRNAdsx,ix,βTub harbors a 3xP3-tdTomato marker and six gRNAs to guide the simultaneous CRISPR/Cas9-mediated disruption of dsx, ix, and βTub genes. Violet exon Box 5a and 5b represent ♀-specific exons. (B) A schematic of the reciprocal genetic cross between the homozygous Cas9, marked with Opie2-CFP, and homozygous gRNAdsx,ix,βTub to generate the trans-hemizygous F1 (aka. pgSIT) progeny. Relative positions of dsx, ix, and βTub target genes (bar color corresponds to gRNA color), and transgene insertions in the Cas9 (Nup50-Cas9 strain1) and gRNAdsx,ix,βTub#1 strains are indicated in the three pairs of Ae. aegypti chromosomes. To assess the fecundity of generated pgSIT mosquitoes, both trans-hemizygous ♀’s and ♂’s were crossed to the wild-type (WT) ♂’s and ♀’s, respectively. (C) Comparison of the survival, sex ratio, and fertility of trans-hemizygous, hemizygous Cas9 or gRNAdsx,ix,βTub#1, and WT mosquitoes. The bar plot shows means ± standard deviation (SD) (n = 3, all data in Supplementary file 1d). (D) Blood feeding assays using both types of trans-hemizygous intersexes (⚥’s): pgSIT♀Cas9 and pgSIT♂Cas9 ⚥’s. To assess blood feeding efficiency, individual mated ♀’s or ⚥’s were allowed to blood feed on an anesthetized mouse inside a smaller (24.5 × 24.5 × 24.5 cm) or larger cage (60 × 60 × 60 cm), and we recorded the time: (1) to initiate blood feeding (i.e., time to bite); and (2) of blood feeding (i.e., feeding time). The plot shows duration means ± SD over 30 ♀’s or ⚥’s (n = 30) for each genetic background (Supplementary file 1e). (E) Flight activity of individual mosquitoes was assessed for 24 hr using vertical Drosophila activity monitoring (DAM, Supplementary file 1f). The plot shows means ± SD (n=24). (F) Mating assays for fertility of offspring produced via crosses between trans-hemizygous ♂’s that inherited a maternal Cas9 (pgSIT♀Cas9) or paternal Cas9 (pgSIT♂Cas9) and WT ♀’s (Supplementary file 1h). The plot shows fertility means ± SD over three biologically independent groups of 50 WT ♀’s (n = 3) for each experimental condition. Statistical significance of mean differences was estimated using a two-sided Student’s t-test with equal variance (ns: p ≥ 0.05, *p < 0.05, **p < 0.01, and ***p < 0.001). Source data are provided in Supplementary file 1.

Genetic characterization of independent transgenic strains for CRISPR/Cas9-mediated disruption of dsx, ix, and βTub.
(A) Schematic maps of targeted genes (box) and gRNAdsx,ix,βTub construct. Relative locations of gRNA target sequences are depicted with red arrows (box). gRNAdsx,ix,βTub harbors a 3xP3-tdTomato marker and six gRNAs to guide the simultaneous CRISPR/Cas9-mediated disruption of dsx, ix, and βTub genes. (B) A schematic of the reciprocal genetic cross between the homozygous Cas9, marked with Opie2-CFP, and homozygous gRNAdsx,ix,βTub#1 to generate the trans-hemizygous F1 (aka. precision-guided sterile insect technique [pgSIT]) progeny. Relative positions of dsx, ix, and βTub genes (bar’s color corresponds to gRNA’s color), and transgene insertions in the Cas9 (Nup50-Cas9 strain) and gRNAdsx,ix,βTub#1 strains are indicated in the three pairs of Ae. aegypti chromosomes. (C) Three independent gRNAdsx,ix,βTub strains were generated and assessed by crossing to the Cas9 strain and comparing the pgSIT phenotypes induced in each trans-hemizygous progenies. The survival, sex ratio, and fertility of trans-hemizygous and hemizygous gRNAdsx,ix,βTub mosquitoes were scored for each of three gRNAdsx,ix,βTub strains and compared to the corresponding values found for gRNAdsx,ix,βTub#1 strain. The bar plot shows means ± standard deviation (SD) over triple biological replicates (n = 3, all data can be found in Supplementary file 1d). Statistical significance of mean differences was estimated using a two-sided Student’s t-test with equal variance (ns: p ≥ 0.05). Source data are provided in Supplementary file 1.

Determination of transgene copy number for the gRNAdsx,ix,βTub#1 strain using Oxford Nanopore genome sequencing.
tandard box plot depicting the coverage distributions of the three chromosomes and the Cas9 and gRNAdsx,ix,βTub transgenes in trans-hemizygous gRNAdsx,ix,βTub#1/Cas9 (aka. precision-guided sterile insect technique [pgSIT]) mosquitoes. The center line is median, first and third quartiles are the bounds of the box, upper and lower whiskers extend from the box to the largest and lowest observed value, but no further than 1.5* interquartile range (IQR) from the box. Sequencing depths for chromosomes 1, 2, 3 and for Cas9 and gRNAdsx,ix,βTub transgenes were 9.71, 9.47, 9.17, 12.23, 9.50, respectively. Normalized sequencing depths were 1.03, 1.00, 0.97, 1.30, 1.01 consistent with the transgenes being present at single copy. The Nanopore sequencing data have been deposited to the NCBI sequence read archive (SRA) under BioProject ID PRJNA942966 and BioSample ID SAMN33705934.

Oxford Nanopore sequencing results validating disruptions in the target sites.
Integrated genome browser snapshots are zoomed in at genome regions containing gRNA target sites at dsx (A), ix (B), and βTub (C) genes in precision-guided sterile insect technique (pgSIT) ♂’s and ♀’s. The direction of DNA strand and scal is indicated above generated reads. Sampled deletion mutations localize to gRNA target sites (yellow overlay) (A).

Integrative genome browser snapshot of dsx validating gRNA target disruption in both the DNA and RNA.
Oxford Nanopore reads are aligned on the top and RNAseq reads for the various genotypes indicated are aligned. On the bottom in blue are the gRNA target sites.

Integrative genome browser snapshot of ix validating gRNA target disruption in both the DNA and RNA.
Oxford Nanopore reads are aligned on the top and RNAseq reads for the various genotypes indicated are aligned. On the bottom in blue is the gRNA target site.

Integrative genome browser snapshot of βTub validating gRNA target disruption in both the DNA and RNA.
Oxford Nanopore reads are aligned on the top and RNAseq reads for the various genotypes indicated are aligned. On the bottom in blue are the gRNA target sites.

Transcription profiling and expression analysis of Ae. aegypti liverpool and precision-guided sterile insect technique (pgSIT) mosquito samples.
(A) Principal Component Analysis (PCA) analysis and (B) hierarchical clustering of twelve samples used for RNA sequencing. MA plots showing the differential expression patterns between: (C) pgSIT ♂’s vs wild-type (WT) ♂’s, and (D) pgSIT ⚥’s vs WT ♀’s. Blue bots indicate significantly differentially expressed genes (False Discovery Rate (FDR) <0.05), while non-significantly differentially expressed genes are indicated by gray dots (FDR >0.05). Targeted and transgenic genes are depicted by the larger blue or gray dots.

Longevity, fecundity, and developmental times of the generated precision-guided sterile insect technique (pgSIT) mosquitoes.
(A) Survival plots of 20 adult ♀’s or ⚥’s either cohabitated with ♂’s or not, and (B) 20 adult ♂’s either cohabitated with ♀’s or not over three independent experiments (n = 3). Survival means ± standard errors (SE) over days following adult eclosion are plotted. Vertical lines and values present median survivals for each tested group. Mosquito survival curves for each tested group were compared to the curve for wild-type (WT) mosquitoes of the corresponding sex. The departure significance was assessed with the Log-rank (Mantel–Cox) test and is indicated above median values. (C) Female fecundity plots, measured as egg laying and hatching rates, of 60 adult ♀’s or ⚥’s of each tested group (n = 60). (D) Plots of larva-to-pupa and pupa-to-adult developmental times were measured in 60 ♂’s and ♀’s or ⚥’s for each tested group (n = 60). Both point plots in panels (C) and (D) show mean ± standard deviation (SD) (Supplementary file 1g). Statistical significance of mean differences was estimated using a two-sided Student’s t-test with equal variance (ns: p ≥ 0.05, **p < 0.01, and ***p < 0.001). Source data are provided in Supplementary file 1.
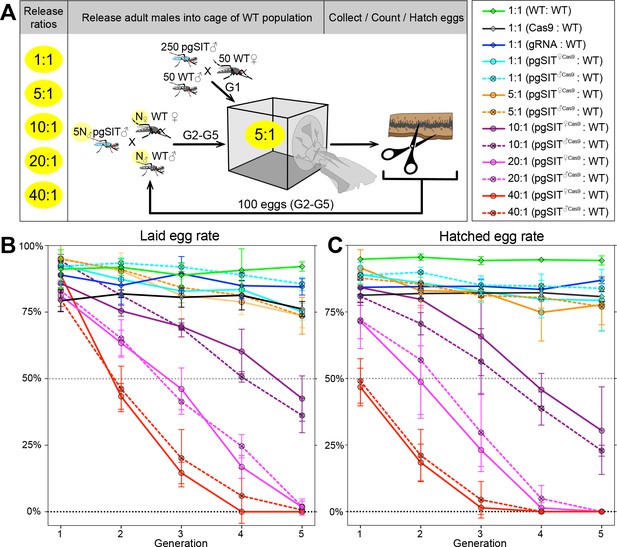
Population suppression in multigenerational population cage experiments.
(A) Multiple precision-guided sterile insect technique (pgSIT):wild-type (WT) release ratios, such as 1:1, 5:1, 10:1, 20:1, and 40:1, were tested in triplicate. A schematic diagram depicts the cage experiments for the 5:1 ratio. To start the first generation (G1) of multigenerational population cages, 250 mature pgSIT adult ♂’s were released with 50 similarly aged WT adult ♂’s into a cage, and in 1 hr 50 virgin ♀’s were added. The mosquitoes were allowed to mate for 2 days before all ♂’s were removed, and ♀’s were blood fed and eggs were collected. All eggs were counted, and 100 eggs were selected randomly to seed the next generation (G2). The sex-sorted adult ♂’s that emerged from 100 eggs (N♂) were released with five times more (5N♂) pgSIT adult ♂’s into a cage, and N♀ ♀’s were added later. The remaining eggs were hatched to measure hatching rates and score transgene markers. This procedure was repeated for five generations for each cage population (Supplementary file 1i). Two types of pgSIT ♂’s were assessed: those that inherited a maternal Cas9 (pgSIT♀Cas9), and those that inherited a paternal Cas9 (pgSIT♂Cas9). Multigeneration population cage data for each release ratio plotting the normalized percentage of laid eggs (B) and hatched eggs (C). Points show means ± standard deviation (SD) for each triplicate sample (n=3). Source data are provided in Supplementary file 1.
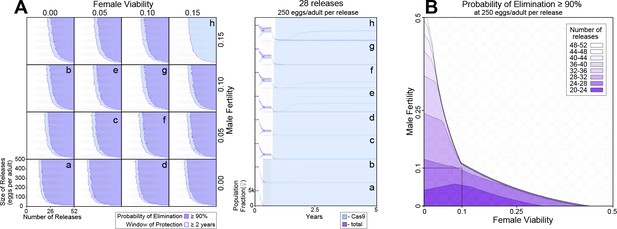
Model-predicted efficacy of precision-guided sterile insect technique (pgSIT) egg releases on Ae. aegypti population suppression as a function of release scheme, male sterility, and female viability.
Weekly releases were simulated in a randomly mixing population of 10,000 adult mosquitoes using the MGDrivE simulation framework2 and parameters described in Supplementary file 1l. Population suppression outcomes were identified as being most sensitive to model parameters describing the release scheme, male fertility, and female viability (Figure 4—figure supplement 1). (A) These parameters were varied in factorial experiments assessing suppression outcomes including probability of elimination (the percentage of 60 stochastic simulations that result in Ae. aegypti elimination), and window of protection (the time duration for which ≥50% of 60 stochastic simulations result in ≥90% suppression of the Ae. aegypti population). Female viability was varied between 0 (complete inviability) and 0.15, male fertility was varied between 0 (complete sterility) and 0.15, release size was varied between 0 and 500 eggs released per wild adult, and the number of weekly releases was varied between 0 and 52. Regions of parameter space for which the probability of elimination exceeds 90% are depicted in purple, and in which the window of protection exceeds two years in light blue. Time-series of population dynamics for select parameter sets are depicted in a–h. Here, the total female population is denoted in red, and the Cas9-carrying female population is denoted in blue. The light blue shaded region represents the window of protection. Imperfect female inviability and male sterility result in lower probabilities of elimination; however the window of protection lasts for several years for male fertility and female viability in the range 0–0.15 for simulated release schemes. (B) Regions of parameter space for which the probability of elimination exceeds 90% are depicted as a function of male fertility (x-axis), female viability (y-axis), and the minimum number of weekly releases required to achieve this (shadings, see key). Release size is set to 250 eggs per wild adult. The shaded square depicts the region of parameter space in which male fertility is between 0% and 10% and female viability is between 0% and 10%. A ≥90% elimination probability is achieved with ~20–32 weekly releases for pgSIT systems having these parameters. Source data are provided in Supplementary file 1.

Sensitivity of precision-guided sterile insect technique (pgSIT) population suppression outcomes to model parameters.
(A) Partial dependency (thin traces) and individual conditional expectation plots3 (magenta dashed lines) are depicted for regression models fitted to three model outcomes: (1) probability of elimination (POE), (2) window of protection (WOP) (measured in years), and (3) reduction in cumulative potential for transmission (RPT). These three outcomes were evaluated for several parameters – number of releases, size of releases (as a proportion of the number of wild adults in the environment), release interval (in days), gRNA cutting rate, Cas9 maternal deposition rate, male fertility, male mating competitiveness (relative to wild-type males), and female viability. Population suppression outcomes are most sensitive to release schedule parameters (number, size, and interval of releases), male fertility, and female viability. (B) Statistical sensitivity analysis metrics on the simulated data are compared to importance metrics for surrogate models. For the first-order statistical sensitivity analysis, we used Delta4, Fourier Amplitude Sensitivity Test (FAST)5, and High-Dimensional Model Representation (HDMR)6; while for the emulators we used Permutation feature importance7, and SHapley Additive exPlanations (SHAP)8. The normalized importance barcharts are in good agreement with the variable-to-outcome rankings (Supplementary file 1l, m). Source data are provided in Supplementary file 1.

Sensitive and rapid detection of transgenic DNA fragments with the sensitive enzymatic nucleic acid sequence reporter (SENSR) assay.
(A) Intensity of signal from SENSR assay for both Cas9 and gRNAdsx,ix,βTub constructs along a concentration gradient, as ratio of pgSIT♀Cas9 to wild-type (WT) mosquitoes, and No Template Control (NTP). The fluorescence signal represents the background-subtracted signal (n = 4). (B) Speed of cleavage along a ratio of pgSIT♀Cas9 to WT mosquitoes, as target abundance. The speed of collateral activity is represented using an half-maximum fluorescence (HMF) analysis (n = 4) (Supplementary file 1o).
Additional files
-
Supplementary file 1
Supplementary tables.
(a) gRNA and primer sequences. (b) Measurements of mosquito morphological structures. (c) Data for dsx and ix single-gene disruption. (d) Data from precision-guided sterile insect technique (pgSIT) genetic cross: dsx, ix, and βTub simultaneous gene disruption. (e) Data on female blood feeding assay. (f) Data on flight activity. (g) Fitness parameters and longevity. (h) pgSIT males sterilize WT females. (i) Data on population suppression. (j) RNA sequencing differential expression analysis using DeSeq2 comparing pgSIT female to WT Liverpool female. (k) RNA sequencing differential expression analysis using DeSeq2 comparing pgSIT male to WT Liverpool male. (l) Life history parameters used in mathematical modeling. (m) Network structure and training schemes for the regression surrogate models. (n) Primers and gRNA sequences for SENSR assay. (o) SENSR assay raw data.
- https://cdn.elifesciences.org/articles/90199/elife-90199-supp1-v1.xlsx
-
MDAR checklist
- https://cdn.elifesciences.org/articles/90199/elife-90199-mdarchecklist1-v1.pdf