Dual-color optical activation and suppression of neurons with high temporal precision
Figures

Reducing blue light-mediated excitation in red-shifted channelrhodopsins – proof of concept.
(A) Schematic representation of the approach. A red-shifted channelrhodopsin (R-ChR) is co-expressed with a blue-shifted anion channel (B-ACR). When red light is ON, there is an overall excitation of the cell due to the more dominant R-ChR response. When blue light is ON, the shunting inhibitory effect of B-ACR reduces the excitation induced by R-ChR to blue light. Created with BioRender. (B) Validation of the approach in flies expressing Chrimson, Chrimson and GtACR2, or GtACR2 alone. (B1) Experimental setup to record the courtship song of solitary male flies. Created with BioRender. (B2) Top: Example of a reconstructed neuronal arborization of P1 neurons, Bottom: example of a typical courtship song induced by LED pulses. (B3) Courtship song production of the solitary male flies during 10 s of constant illumination with 590 nm (amber) or 470 nm (blue) light. Each dot represents an individual Drosophila (mean ± SEM, n=5-18).
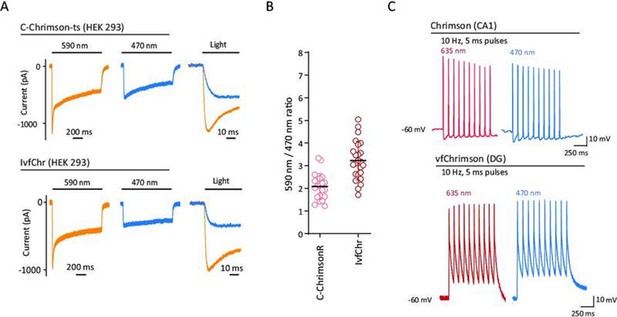
Red-shifted ChR variants are activated by 470 and 590 nm pulses of light.
(A) Typical photocurrents (left and middle traces) and on-kinetics (right traces) of ChrimsonR and IvfChr in HEK 293 cells with 590 and 470 nm illumination at 10 mW/mm2. The traces have been recorded 15 s after a 405-nm reconditioning pulse. (B) Comparison of 590/470 nm ratio for ChrimsonR (n=21) and IvfChr (n=24), measured at peak. Each dot represents a cell (mean ± SD). (C) As for red-light stimulation, blue light illumination of the Chrimson variants induces firing. This property is represented here with two examples of traces obtained from ChrimsonR-expressing CA1 neurons and vfChrimson-expressing DG neurons, in response to 10 Hz of 5 ms pulses at 635 and 470 nm.
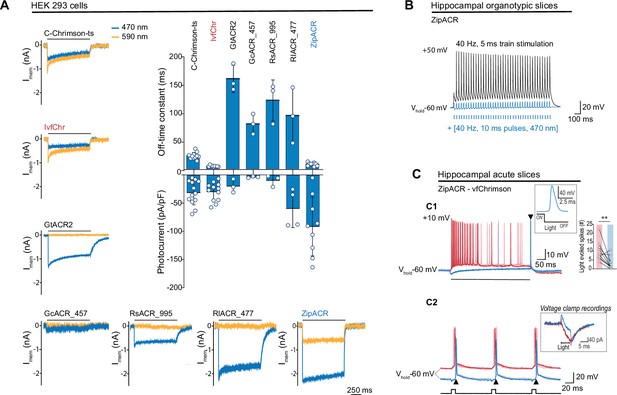
Comparison of channel properties of light-gated chloride channels and Chrimson variants.
(A) Representative photocurrents of the Chrimson and ACRs variants in whole-cell patch-clamp recordings from HEK 293 cells with 590 and 470 nm illumination (top black bars, 10 mW/mm2). The plot shows the off-time constant (top) following 1 s of 470 nm illumination, and the peak photocurrent (bottom) at –60 mV (mean ± SD, n = 3–13 cells). (B) Typical response of CA1 cells from hippocampal organotypic slices expressing ZipACR to 40 Hz, 5 ms pulses (top black trace) and to an illumination protocol using 40 Hz of 10 ms light pulses at 470 nm (4 mW/mm2) (bottom blue trace). (C) Recordings from dentate gyrus granule cells in acute slices expressing ZipACR and vfChrimson. (C1) Representative responses to 500-ms illumination (bottom black bar) at 470 and 635 nm (10 mW/mm2) (blue and red traces, respectively). Note, 635 nm illumination evoked action potential (AP) firing while 470 nm illumination only evoked a single AP time-locked to the end of the light pulse (arrowhead). Insets, example of an AP upon 470 nm light termination and plot showing the number of light-evoked spikes at 635 nm (red bar) and 470 nm (blue bar) illumination. n = 10 cells, paired t-test **p < 0.01. (C2) Example of light-induced firings by 635 and 470 nm illumination (red and blue traces, respectively, 5 ms pulses (black squares) at 10 Hz). The arrowheads show consistent APs time-locked to the end of the blue light pulse. Inset, example of a voltage-clamp recording of currents evoked by 5 ms pulses of 635 and 470 nm light (red and blue traces, respectively). Light-induced currents with 470 nm illumination were initially outward but turned inward gradually and peaked immediately following the light offset. Note that the late inward current matches the red light-induced current, reflecting a slower kinetics for vfChrimson compare to ZipACR.

Improved membrane trafficking of vfChrimson and ChR co-expression designs.
(A) Representative images of various vfChrimson-based expression cassette in HEK 293 cells and membrane tethered mCherry. (Left column) The expression pattern of palmitoylated mCherry on the cellular membrane (using a lysine rich and CaaX sequence). (Middle column) Images of the citrine attached to vfChrimson in the various designs. (Right column) Overlaid images of the two channels for co-expression visualization. vfChrimson is directly tethered to citrine (vfChrimson), or tethered to citrine with addition of trafficking sequence between ChR and citrine (C-vfChrimson), with the addition of N-terminal signaling peptide (from oChIEF, vfChrimson-ts) and with the inclusion of both trafficking sequence and N-terminal signaling peptide (denoted IvfChr). (Bottom panel) The comparison of the Pearson correlation coefficients of mCherry and citrine fluorescence intensities of the different constructs. Statistical tests were performed with Kruskal–Wallis test followed by Dunn’s multiple comparison tests between all pairs. IvfChr has the most optimized membrane trafficking followed by the 2A-based ZipV-IvfChr. (B) Left, examples of BiPOLES-vfChrimson and ZipV-IvfChr response to 590 nm light illumination at –40 mV holding potential. Right, peak photocurrent amplitude comparison between BiPOLES-vfChrimson and ZipV-IvfChr adjusted to membrane capacitance. Unpaired t-test was used. (C) Example of membrane enriched expression of 2A-based ZipT-IvfChr and ZipV-IvfChr in organotypic slices. (D) Comparison of the amplitudes of photocurrent of IvfChr and ZipV-IvfChr in HEK 293 cells to 590 nm light illumination.
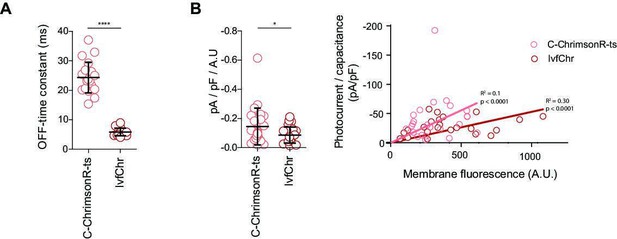
Membrane trafficking of the improved ultrafast vf-Chrimson, IvfChrimson (IvfChr).
(A) Off-time constant of C-ChrimsonR-ts and IvfChr after 1 s of 635 nm illumination at 10 mW/mm2. (B) Photocurrent amplitudes at the peak of C-ChrimsonR-ts and IvfChr, adjusted to membrane fluorescence (left), and comparison of current density and membrane fluorescence (right). The recordings have been obtained from HEK 293 cells at –60 mV. Each dot represents the values from a single cell. The horizontal black bars represent the mean ± SEM for (A) and mean ± SD for (B). Statistics for panels (A) ****p < 0.0001; unpaired t-test and (B) left, *p < 0.05; unpaired t-test (Welch correction): Right, p represents the significance of the deviation from zero, and R2 the goodness of the fit.
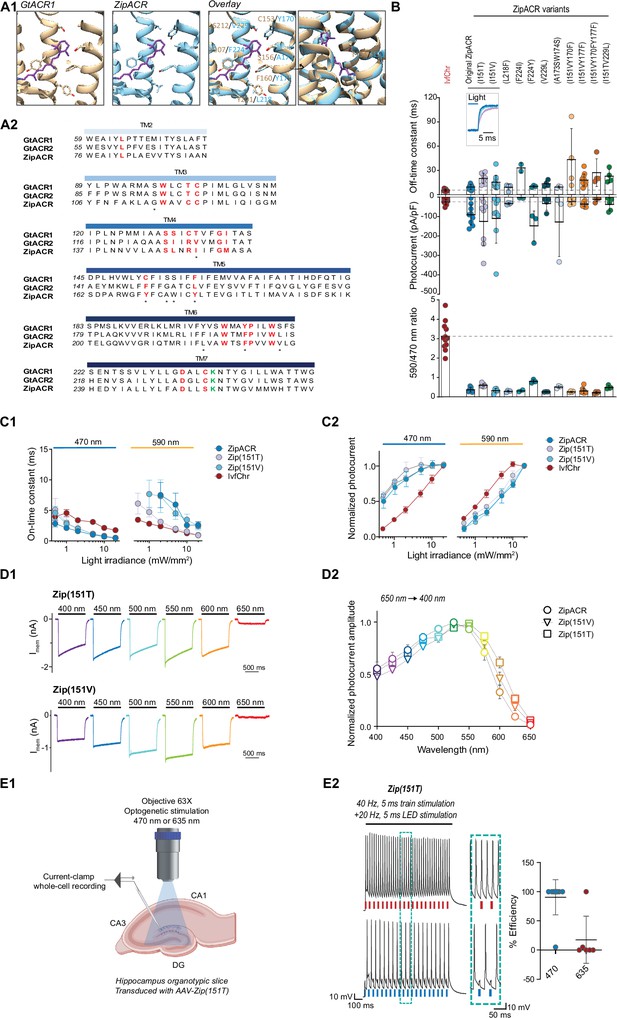
Characterization of the optimized variants of the ultrafast anion channelrhodopsin ZipACR.
(A) Structure-based design of ZipACR variants. (A1) The retinal binding pocket of GtACR1 and the putative retinal binding pocket of ZipACR homology model. (A2) The alignment of the transmembrane domains (TM) of GtACR1, GtACR2 and ZipACR. The residues surrounding the retinal binding pocket of GtACR1 and the corresponding residues from GtACR2 and ZipACR are highlighted in red. *marks the residues targeted for mutation. The lysine that forms the Schiff base with retinal is indicated in green. The numbers on the left correspond to the number of the first residue in each line. (B) Basic properties of the ZipACR variants compared to IvfChr. Off-time constant (top) following 1 s of 470 nm illumination, peak photocurrent (middle) and 590/470 ratio (bottom). The data have been obtained from whole-cell recordings in HEK 293 cells. Each dot corresponds to one cell (mean ± SD, n = 2–12). (C) On-time constant (C1) and normalized photocurrent (C2) of the selected variants Zip(151T) and Zip(151V) as compared to the original ZipACR and IvfChr, with 1 s 470 or 590 nm illumination of various light intensities (in mW/mm2). For the photocurrent, the values have been measured at the maximum response. For C1, the responses of ZipACR and Zip(151V) to low intensities of 590 nm light were too small for the accurate fitting of the on-time constant and were not recorded. The data points are the mean value ± SEM (n = 6–7). (D) The responses of the selected variants to various wavelengths of the same photon flux. (D1) The action spectra of ZipACR, Zip(151V) and Zip(151T) as measured from 650 to 400 nm (D2) For the action spectra, the maximum photocurrent amplitudes measured at each wavelength were normalized to the peak values obtained from the same cell across the spectrum. The data points are the mean value ± SEM (n = 6–7). (E) Validation of the efficiency of the Zip(151T) variant in slices. (E1) The response of dentate gyrus granule cells expressing Zip(151T) variant at 470 nm (4 mW/mm2) and 635 nm (7 mW/mm2) light pulses delivered through a 63× objective. Created with BioRender. (E2) Representative traces of the firing induced by current injection at 40 Hz. Overlapping 470 nm but not 635 nm pulses block action potentials. The plot on the right shows the efficiency (in %) of Zip(151T) in blocking individual action potentials at 470 and 635 nm light pulses (mean ± SD, n = 6–10 cells). The traces of the two outliers are presented in Figure 3—figure supplement 3A.
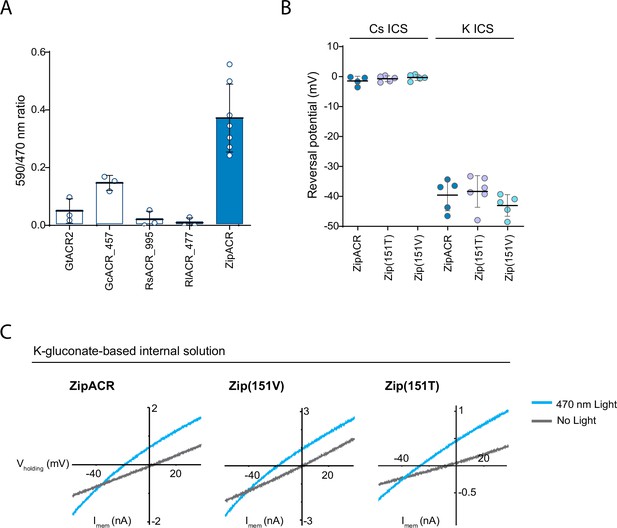
Channel properties of the ZipACR variants in HEK 293 cells.
(A) 590/470 nm ratio measured from the ACR variants. Each circle corresponds to value obtained from one cell (mean ± SD, n = 3–7). (B) Reversal potential of the selected variants Zip(151T) and Zip(151V) compared to the original ZipACR with cesium (Cs) or potassium gluconate (K) intracellular solution. Each dot corresponds to the value obtained from one cell (mean ± SD, n = 5–6). No significant differences were detected between the three variants in Cs- and K-based intracellular solutions (one-way ANOVA and p = 0.38 and p = 0.29 for Cs- and K-based intracellular solution, respectively). (C) Typical I/V relationship for the two variants and ZipACR. The recordings have been done with- or without 470 nm LED illumination. The reversal potential is measured at the point of the two curves crossing.
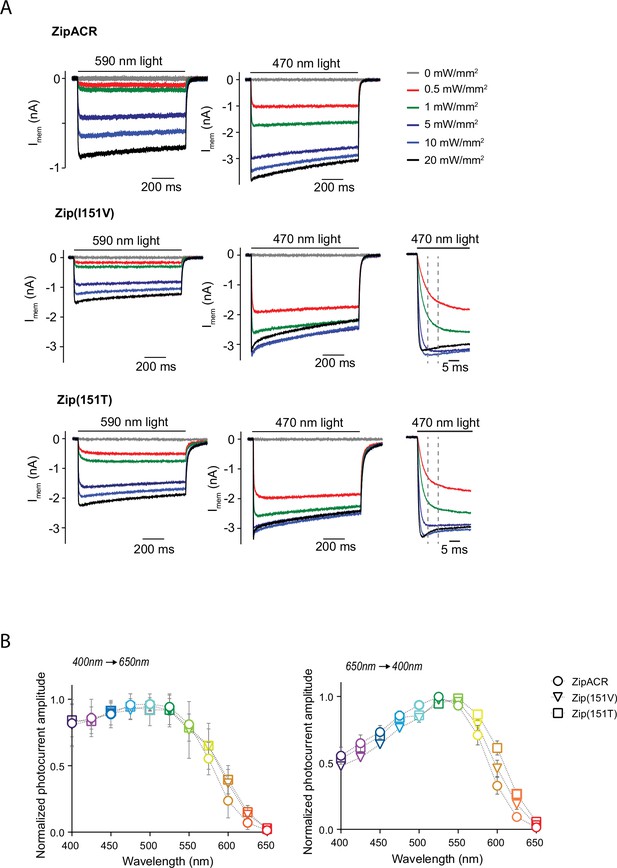
Light-induced responses and action spectra of ZipACR variants.
(A) Representative responses of the original ZipACR, Zip(151T), and Zip(151V) in HEK 293 cells in response to different light intensities. (B) Normalized photocurrent amplitude in response to 400–650 and 650–400 nm light pulses (as presented in Figure 3D2). The values have been measured on the photocurrents at the peak. The responses were normalized to the maximum response of each cell across the spectrum. The data points are the mean value ± SEM (n = 6–7).
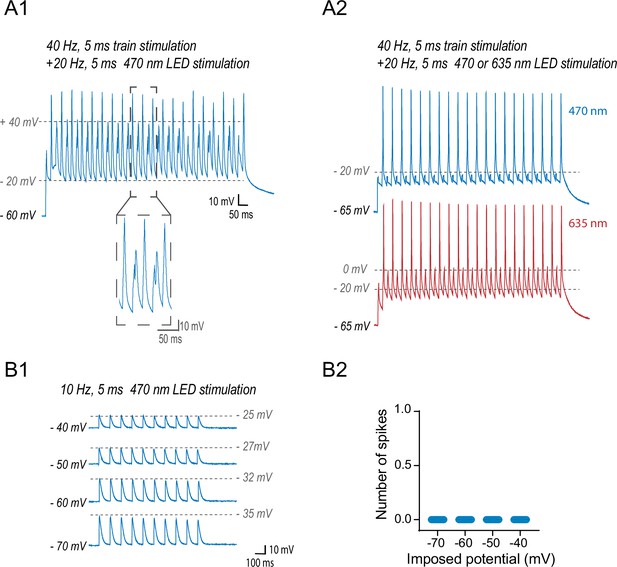
Zip(151T) fails to produce AP under 470 nm illumination.
(A) The traces of the two outliers in Figure 3E2. (A1) Here, 470 nm illumination reduced the amplitude of the spikes, but did not abolish the firing. (A2) 470 nm illumination as well as 635 nm light blocked the firing. This cell expressed Zip(151T) channels which was significantly more red-shifted compared to the mean (635/470 nm ratio: 23% for this cell vs 6% for the averaged population). (B) For a range of imposed potentials 470 nm illumination of Zip(151T) does not trigger action potentials. (B1) Typical traces of the DG neurons at various imposed potentials, in response to 10 Hz of 470 nm light illumination. The dotted lines show the potential of the maximal response. (B2) Plot showing the responses in current-clamp of the 10 neurons recorded at −70,–60, –50, and –40 mV imposed potential. Each dot represents a single cell.
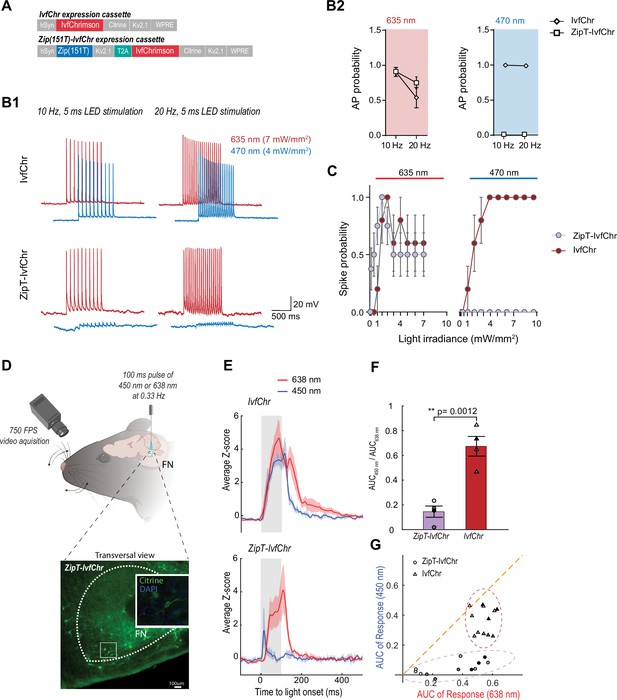
Zip(151T) variant co-expressed with IvfChr prevents blue light-induced action potentials, in vitro and in vivo.
(A) The expression cassette for IvfChr and bicistronic ZipT-IvfChr used in rAAV vector. The selected ZipACR variant Zip(151T) is co-expressed with the red-sensitive IvfChr by using the 2A self-cleaving peptide (T2A). All the opsins are soma-targeted with a Kv2.1 peptide. (B) Comparison of the high-frequency red and blue light-induced spiking (B1), and spike probability (B2), n = 6–13 for each opsin, of DG cells in organotypic slices expressing IvfChr or ZipT-IvfChr, with 10 and 20 Hz of light pulses (4–7 mW/mm2). Holding potential = –60 mV. (C) Blue and red light-driven spike fidelity at various light intensities in DG cells (n = 4–10 cells for each opsin; 10 ms pulse width, single pulse). (D) Experimental design. ZipT-IvfChr or IvfChr are expressed in the facial nucleus (FN) of the brainstem (top) and light-induced whisker movements are recorded. Example of soma-targeted expression of our ZipT-IvfChr construct in FN (bottom). Created with BioRender. (E) Averaged Z-scores of the angle of the whiskers under 450 and 638 nm illumination (20 mW/ mm2) of the FN motoneurons. As opposed to IvfChr, ZipT-IvfChr failed to reliably elicit whiskers movement under blue light (n = 4 animals per condition, see also Supplementary material 2). (F) Ratio of the area under the curve (AUC) of the responses to 450 and 638 nm illumination for ZipT-IvfChr and IvfChr (n = 4 animals per condition, **p < 0.01, t-test). (G) Distribution of the AUC for three individual trials from each animal at 450 and 638 nm illumination (n = 4 animals per condition).
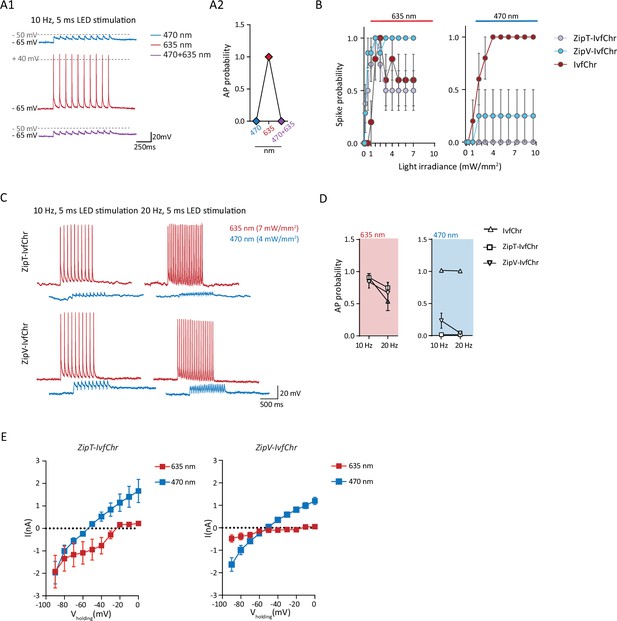
ZipV-IvfChr variant, as for ZipT-IvfChr, is effective in preventing blue light-induced action potentials (APs) in vitro.
(A) Example of the response (A1) of ZipT-IvChr-expressing DG neurons to 470 and 635 nm light pulses (10 Hz of 5 ms light pulses, 4–7 mW/mm2) and to a co-illumination protocol (470 + 635 nm). ZipT-IvChr is efficient in preventing the firing of APs to 470 nm and to a co-illumination protocol (A2), n = 6. (B) Blue and red light-driven spike fidelity at various light intensities in DG cells n = 4–8 cells for each opsin; 10 ms pulse width, single pulse. Under 470 nm illumination, neurons expressing ZipV-IvfChr, occasionally fired APs, which may be explained by the variant relatively faster closure rate (see Figure 3B). Comparison of the high-frequency red and blue light-induced spiking (C), and spike probability (D), n = 6–13 for each opsin, of DG cells expressing IvfChr or the Zip-IvfChr variants, with 10 and 20 Hz of light pulses (4–7 mW/mm2). Holding potential = –60 mV. (E) I–V curve measured for both variants ZipT-IvChr and ZipV-IvChr under 635 or 470 nm illumination (n = 8 per variant). Stimulation with 635 nm light induced larger photocurrents during the IV protocol in some cells expressing ZipT-IvfChr compared to ZipV-IvfChr, at hyperpolarized holding potentials. However, for both constructs, we observed as expected a reversal potential close to 0 under red light –20 and –10 mV for ZipT-IvfChr and ZipV-IvfChr, respectively, see Mager et al., 2018 and a reversal potential close to –60 mV (–55 mV for both constructs, see Govorunova et al., 2017) under blue light.
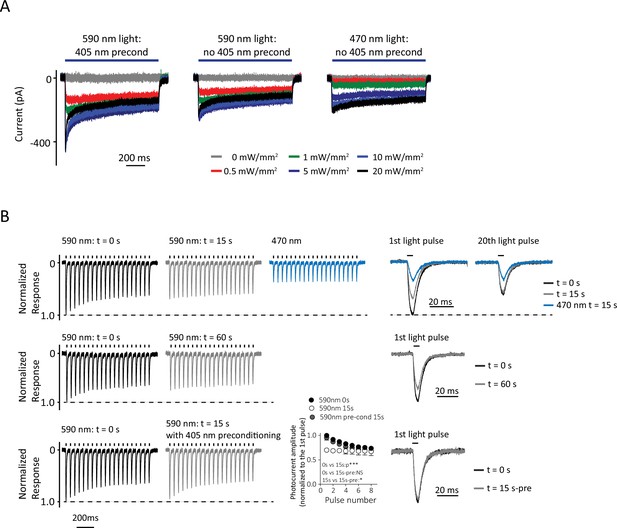
Desensitization of IvfChr in HEK 293 cells.
(A) Representative responses of IvfChr to 1 s of 470 and 590 nm with or without a preconditioning pulse at 405 nm. Desensitization is much less with 470 nm light stimulation. (B) Normalized responses in voltage-clamp to 590 nm pulses of light, at t = 0, t = 15, and t = 60 s without 405 nm preconditioning. The desensitization of IvfChr to 590 nm light does not recover fully at least within 1 min. A pre-reconditioning pulse at 405 nm enhanced the recovery of the 590 nm responses (Kruskal–Wallis test on the normalized responses of the first eight pics before the observable steady-state, for 0 s (n = 7), 15 s (n = 7), and 15 s with 405 nm preconditioning (15s+pre, n = 3), **p = 0.0010; Dunn’s multiple comparison test: 0 vs 15 s, p***; 0 vs 15s+pre, p = non-significant; 15 vs 15s+pre, p*).
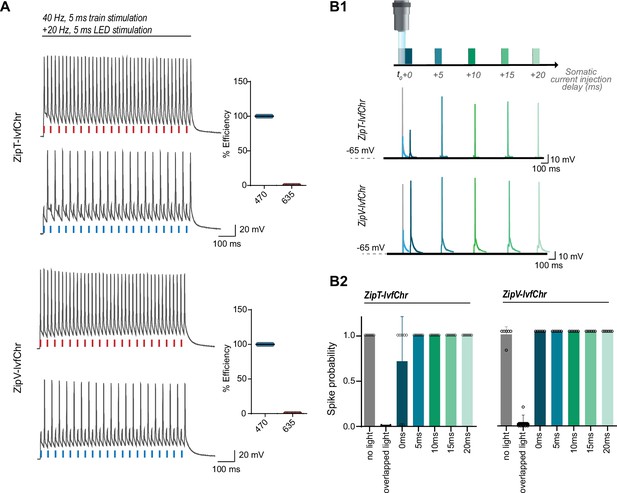
The blue light-induced suppression of action potentials is confined within the duration of the light pulse.
(A) Optical inhibition of spiking in DG cells expressing the ZipT-IvfChr and ZipV-IvfChr variants, in organotypic slices. Representative traces of the firing induced by current injection at 40 Hz. Overlapping 470 nm but not 635 nm light pulses block action potentials. Holding potential = –60 mV. Plots on the right shows the efficiency (in %) of ZipT-IvfChr in blocking individual action potentials at 470 and 635 nm (n = 9–10). (B) Temporal recovery of neuronal excitability following light-induced inhibition by the ZipACR variants. (B1) Experimental paradigm. DG granule cells expressing ZipT-IvfChr or ZipV-IvfChr have been stimulated by a somatic current injection (5 ms pulse) overlapping or preceded by a 470-nm pulse (5 ms, 4 mW/mm2) at 0, 5, 10, 15, or 20 ms intervals. The traces in current clamp (bottom) are representative of the responses to current injections. (B2) The spike probability shows a fast recovery of ZipT-IvfChr (<5 ms) and an immediate recovery of ZipV-IvfChr, from the off-set of the 470 nm light (n = 7 for ZipT-IvfChr, n = 6 for ZipV-IvfChr, mean ± SD).
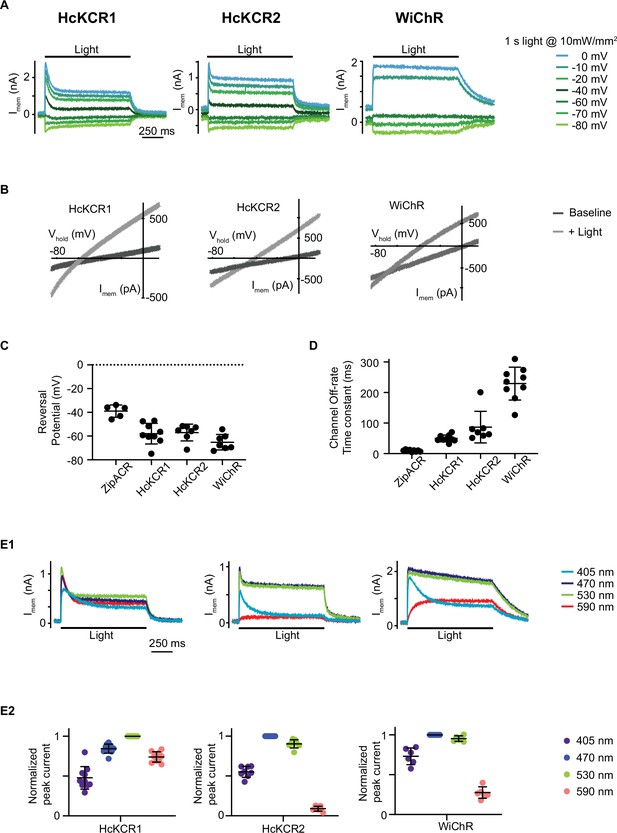
Evaluation of light-gated potassium channels HcKCR1, HcKCR2, and WiChR.
(A) Example of electrophysiological recordings of HEK 293 cells expressing HcKCR1, HcKCR2, and WiChR to 1 s of 470 nm light (HcKCR2 and WiChR) or 530 nm light (HcKCR1) at various membrane holding potentials. (B) Reversal potential generated with a ramp protocol. Dark gray light is the ramp profile in the absence of light and light gray light is the ramp profile during light illumination. Created with BioRender. (C) Measurements of the channels reversal potential. Each dot is a measurement from one cell and the lines represent the mean ± SD. (D) The channel off-rate time constant measured at –10 mV in response to 530 nm light (HcKCR1) and 470 nm light (HcKCR2 and WiChR). Each dot is a measurement from one cell and the lines represent the mean ± SD. (E1) Examples of recordings of HEK 293 cells expressing HcKCR1 (left), HcKCR2 (middle) and WiChR (right) to 1 s of 10 mW/mm2 light at the indicated wavelengths. The cells were held at –10 mV with voltage-clamp. (E2) The amplitudes of light-evoked response of HcKCR1, HcKCR2, and WiChR to 10 mW/mm2 of 405, 470, 530, and 590 nm light is normalized to the peak response of the cells for each of the four tested wavelengths. HcKCR1 has the peak response at 530 nm and HcKCR2 and WiChR have the peak responses at 470 nm.
Additional files
-
Supplementary file 1
The annotated sequence of the 2A-based ZipT-IvfChr design used in this study.
For ZipACR(151V), position 151 of ZipACR is valine and encoded by the codon GTC and the sequence of IvfChrimson-citrine starts at polypeptide sequence position 409. Note that the flexible linkers and amino acids introduced by the digestion sites are not annotated.
- https://cdn.elifesciences.org/articles/90327/elife-90327-supp1-v1.docx
-
Supplementary file 2
Area under the curve (AUC) for the whiskers protraction triggered by red or blue light illumination of the facial nucleus neurons expressing ZipT-IvfChr or IvfChrimson.
- https://cdn.elifesciences.org/articles/90327/elife-90327-supp2-v1.docx
-
MDAR checklist
- https://cdn.elifesciences.org/articles/90327/elife-90327-mdarchecklist1-v1.docx