Structure and dynamics of cholesterol-mediated aquaporin-0 arrays and implications for lipid rafts
Figures
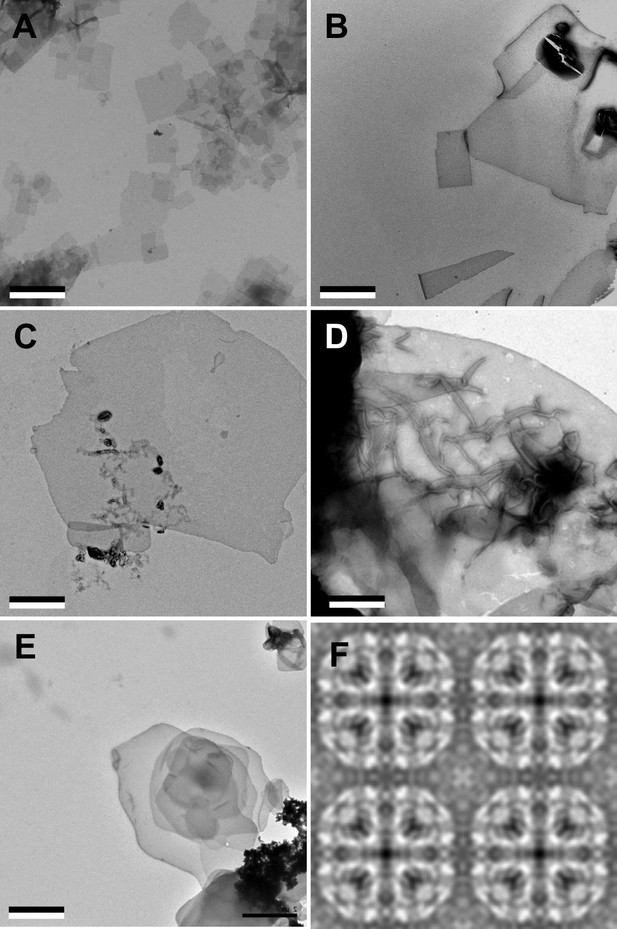
Aquaporin-0 (AQP0) forms two-dimensional (2D) crystals with all tested sphingomyelin/cholesterol mixtures.
(A–E) AQP0 purified from sheep lenses was reconstituted with pure sphingomyelin (A), sphingomyelin/cholesterol mixtures at molar ratios of 2:1 (B), 1:2 (C), and 1:4 (D), as well as pure cholesterol (E). AQP0 was reconstituted under all conditions and formed diffracting 2D crystals. The scale bars are 2 μm. (F) Projection map of AQP0 reconstituted with pure cholesterol at 3.2 Å resolution. The 2D crystals show p422 symmetry and have the typical lattice constants for AQP0 crystals of a=b=65.5 Å, and γ=90°. The panel shows two-by-two unit cells. See also Figure 1—figure supplements 1 and 2 and Tables 1 and 2.
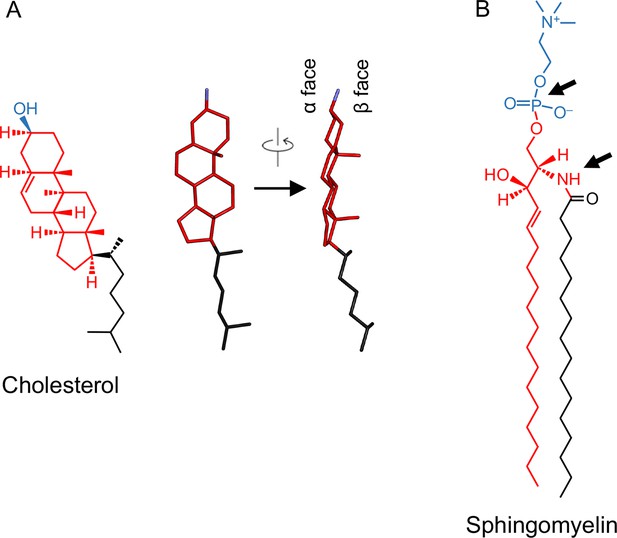
Chemical structures of raft lipids.
(A) Cholesterol consists of a planar four-aromatic ring structure (red) with a short isooctyl alkyl chain (black) and a small 3-β-hydroxyl head group (blue). The side view shows the asymmetric distribution of the aliphatic groups linked to the ring system and the resulting smooth (α) and rough (β) faces of the cholesterol molecule. (B) Sphingomyelin consists of a sphingosine amino alcohol (red) with a fatty acid (black) and phosphocholine head group (blue).
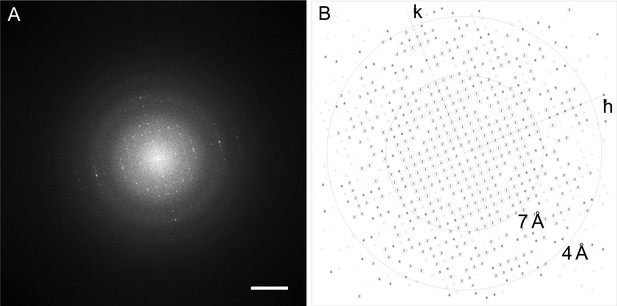
Diffraction of an image of an aquaporin-0 (AQP0) two-dimensional (2D) crystal grown with pure cholesterol.
(A) Power spectrum of a raw image of an AQP0 2D crystal in a pure cholesterol membrane. The scale bar represents (10 Å)–1. (B) Intensity quotient (IQ) plot after computational lattice unbending. After lattice unbending of the image in 2dx (Gipson et al., 2007b), the IQ plot showed reflections with IQ values of 3 (corresponding to a peak-to-background ratio of 2.3) to a resolution better than 4 Å.
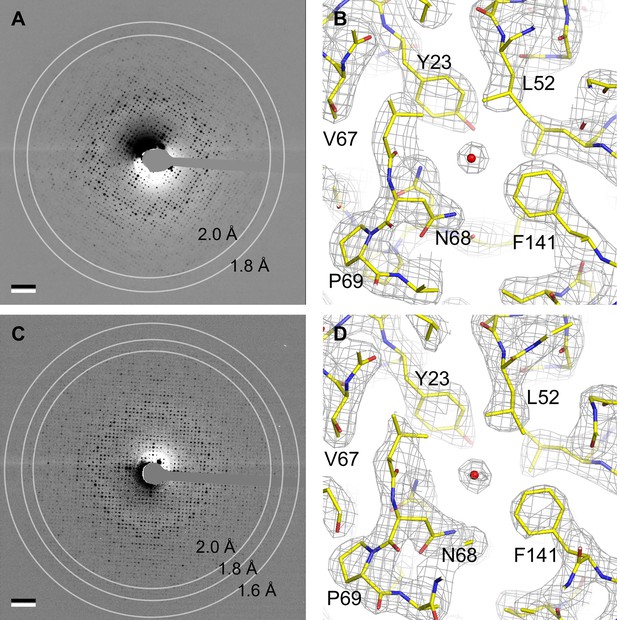
Electron crystallography provides structures of aquaporin-0 (AQP0) in sphingomyelin/ cholesterol bilayers at 2.5 Å resolution.
(A) Electron diffraction pattern of an untilted AQP0 two-dimensional (2D) crystal reconstituted at a sphingomyelin:cholesterol ratio of 2:1, showing reflections to ~2 Å resolution. Scale bar indicates (10 Å)–1. (B) Density map at 2.5 Å resolution used to build the AQP02SM:1Chol structure. A region of the water-conducting pathway close to the NPA (asparagine-proline-alanine), the AQP signature motif, is shown. The 2Fo-Fc map contoured at 1.5σ is shown as gray mesh, the AQP0 model is shown in yellow with oxygen atoms in red and nitrogen atoms in blue. The red sphere represents a water molecule. (C) A diffraction pattern of an untilted AQP0 2D crystal reconstituted at a sphingomyelin:cholesterol ratio of 1:2, showing reflections to better than 1.6 Å resolution. Scale bar indicates (10 Å)–1. (D) Density map at 2.5 Å resolution used to build the AQP01SM:2Chol structure. The same region as in (B) is shown with the same color code. See also Figure 2—figure supplement 1.
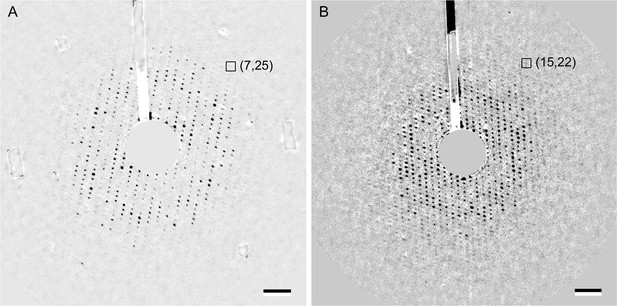
Electron diffraction patterns of two-dimensional (2D) crystals reconstituted with sphingomyelin/cholesterol mixtures tilted to 60°.
(A) Background-subtracted electron diffraction pattern of a 60° tilted aquaporin-0 (AQP0) 2D crystal reconstituted at a molar sphingomyelin:cholesterol ratio of 2:1. The boxed reflection corresponds to a resolution of 2.52 Å resolution. (B) Background-subtracted electron diffraction pattern of a 60° tilted AQP0 2D crystal reconstituted at a molar sphingomyelin:cholesterol ratio of 1:2. The boxed reflection corresponds to a resolution of 2.46 Å resolution. Scale bars indicate (10 Å)–1.
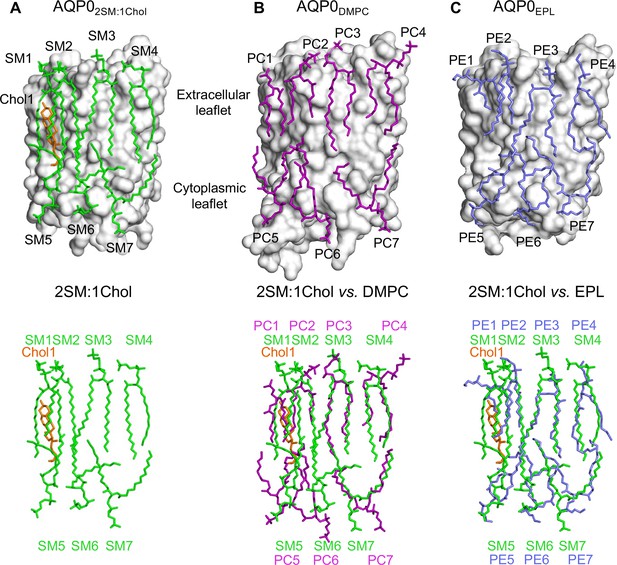
The 2:1 sphingomyelin/cholesterol bilayer surrounding aquaporin-0 (AQP0) is similar to bilayers formed by phosphoglycerolipids.
(A) The top panel shows the seven sphingomyelins (light green sticks) and one cholesterol (orange sticks) molecules forming the bilayer around an AQP0 subunit (gray surface). The bottom panel shows just the lipid bilayer. (B) The top panel shows dimyristoyl phosphatidylcholine (DMPC) lipids (purple sticks) surrounding an AQP0 subunit (Gonen et al., 2005) and the bottom layer shows an overlay of the DMPC bilayer with the 2:1 sphingomyelin/cholesterol bilayer. (C) The top panel shows an Escherichia coli polar lipids extract (EPL) bilayer (modeled as PE lipids) (light brown sticks) surrounding an AQP0 subunit (Hite et al., 2010) and the bottom layer shows an overlay of the EPL bilayer with the 2:1 sphingomyelin/cholesterol bilayer. See also Figure 3—figure supplements 1–3 and Table 3.
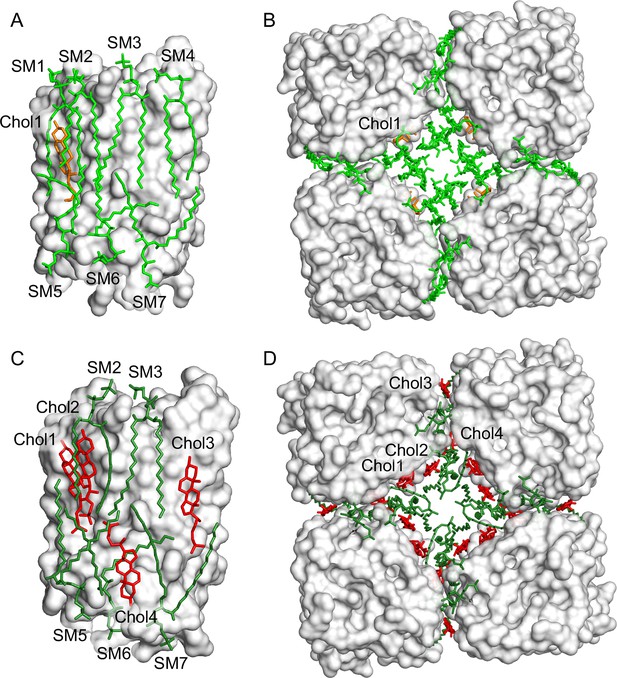
Distribution of sphingomyelin and cholesterol molecules around aquaporin-0 (AQP0) in two-dimensional (2D) crystals.
(A–B) 2D crystals grown with a molar sphingomyelin:cholesterol ratio of 2:1. (C–D) 2D crystals grown with a molar sphingomyelin:cholesterol ratio of 1:2. Lipid distribution in AQP0 arrays formed with a molar sphingomyelin:cholesterol ratio of 2:1. Panels (A) and (C) show the side view of a single AQP0 subunit, and panels (B) and (D) show a top view of four subunits (from different tetramers) in the area where four different tetramers come together in the array. Note that lipids at the crystallographic fourfold axis could not be modeled.
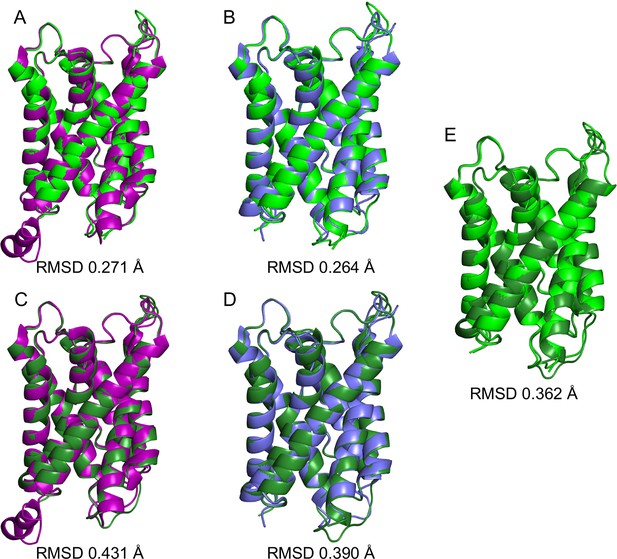
Structure comparisons of aquaporin-0 (AQP0) in different lipid bilayers.
The root mean square deviation (RMSD) between the Cα atoms of AQP0 structures determined in membranes formed with different lipids is very low and variations between structures are largely constrained to extramembranous loops. (A) AQP02SM:1Chol (light green) versus AQP0DMPC (purple). (B) AQP02SM:1Chol (light green) versus AQP0EPL (blue). (C) AQP01SM:2Chol (dark green) versus AQP0DMPC (purple). (D) AQP01SM:2Chol (dark green) versus AQP0DMPC (purple). (E) AQP02SM:1Chol (light green) versus AQP01SM:2Chol (dark green).
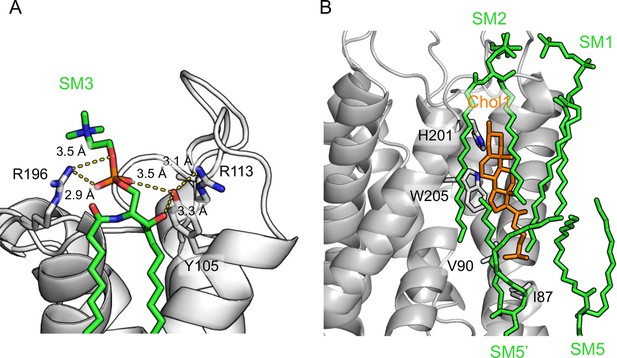
Interactions of sphingomyelin lipids in AQP02SM:1Chol with aquaporin-0 (AQP0) and Chol1.
(A) The head group of sphingomyelin SM3 interacts with AQP0 residues Tyr-105 and Arg-196. Yellow dashed lines indicate the hydrogen bonds. (B) The acyl chains of sphingomyelins SM1, SM2, SM5, and SM5’ have hydrophobic interactions with cholesterol Chol1, as well as AQP0 residues of His-201, Trp-205, Val-90, and Ile-87.
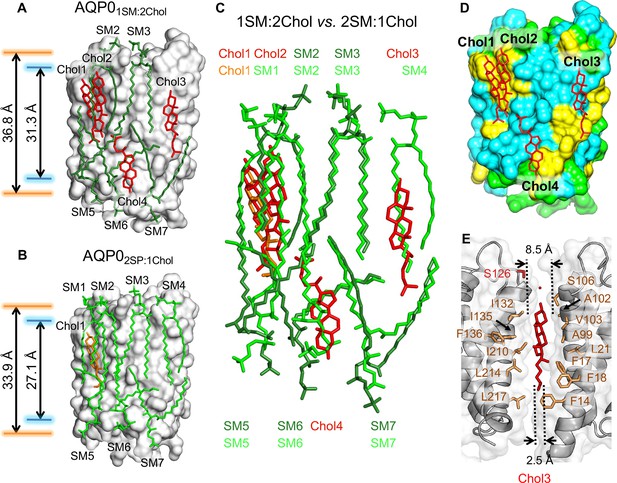
The 1:2 sphingomyelin/cholesterol bilayer surrounding aquaporin-0 (AQP0) and comparison with the 2:1 sphingomyelin/cholesterol bilayer.
(A) The five sphingomyelins (dark green sticks) and four cholesterol (red sticks) molecules surrounding an AQP0 subunit (gray surface). The arrows between the orange and blue lines indicate the average distances between the phosphorus atoms of the phosphodiester groups and the nitrogen atoms of the amide groups in the two leaflets, respectively. (B) The AQP02SM:1Chol structure shown for comparison with the AQP01SM:2Chol structure in (A). Arrows as in (A). (C) Overlay of the lipid bilayers in the AQP02SM:1Chol and AQP01SM:2Chol structures. (D) Location of the four cholesterols (red sticks) in the AQP01SM:2Chol structure with respect to AQP0 surface characteristics. Color coding: yellow, aromatic residues; cyan, hydrophobic residues; and light green, polar and charged residues. (E) Position of cholesterol Chol3 (red sticks) in the AQP01SM:2Chol structure and its interaction with residues of two adjacent AQP0 tetramers (brown sticks). The dotted lines indicate the distance between the two adjacent AQP0 tetramers at the positions of the ring system (~8.5 Å) and the acyl chain (~2.5 Å). See also Figure 4—figure supplements 1 and 2 and Table 3.
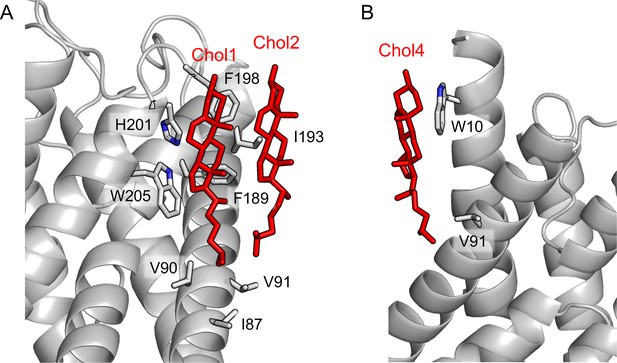
Interactions of cholesterol molecules in AQP01SM:2Chol with aquaporin-0 (AQP0).
(A) The cholesterols in the extracellular leaflet, Chol1 and Chol2. (B) The cholesterol in the cytoplasmic leaflet, Chol4. All cholesterol molecules interact with hydrophobic residues on the AQP0 surface. The ring systems of Chol1, Chol2, and Chol4 also make π-stacking interactions with aromatic residues Trp-205, Phe-198, and Trp-10, respectively.
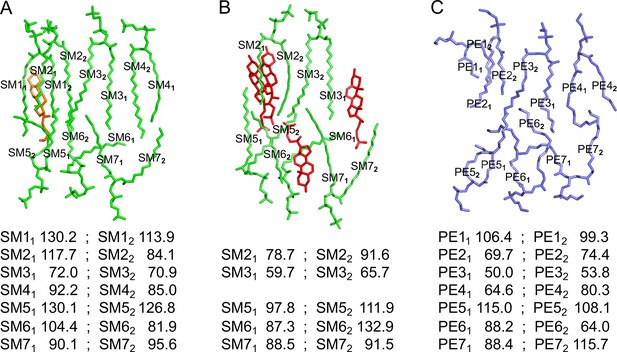
Average B-factors of acyl chains in structures of aquaporin-0 (AQP0) in different lipid bilayers.
(A) AQP0 in a bilayer formed with a molar sphingomyelin:cholesterol ratio of 1:2. (B) AQP0 in a bilayer formed with a molar sphingomyelin:cholesterol ratio of 2:1. (C) AQP0 in a bilayer formed with E. coli polar lipids. The two acyl chains of each lipid are labeled in the top panel with subscripts and their average B-factors are tabulated below.
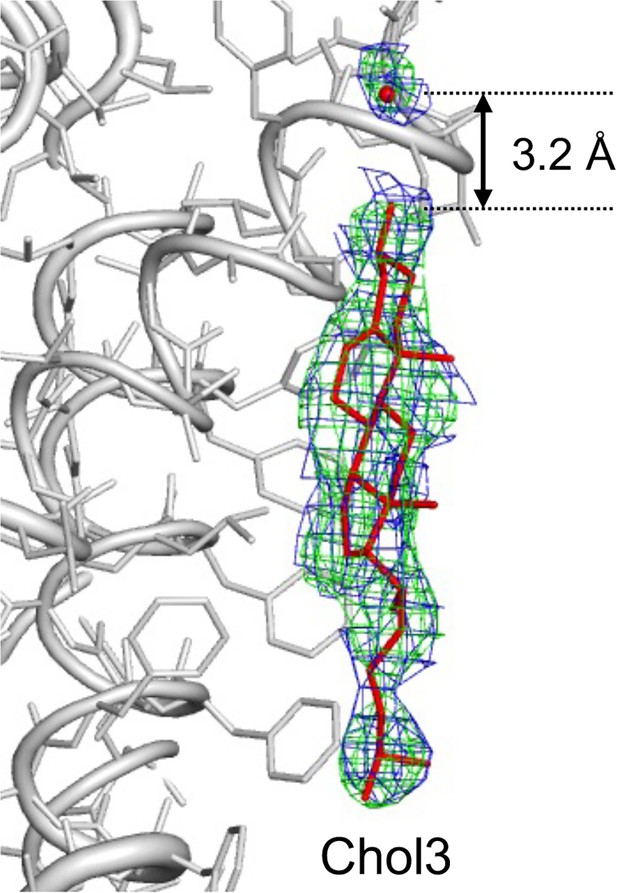
The hydroxyl head group of Chol3 makes a hydrogen bond with a water molecule.
The distance of the water molecule from the Chol3 hydroxyl group is 3.2 Å. Aquaporin-0 (AQP0) is shown in gray ribbon and sticks representation, Chol3 is shown as red sticks, and water as a red sphere. The 2Fo-Fc map for Chol3 and the water molecule is shown in green and the composite omit map in blue.
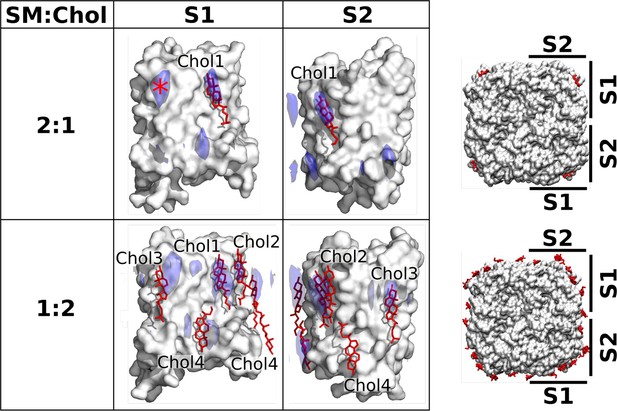
Localization of cholesterol around aquaporin-0 (AQP0) monomers from unbiased molecular dynamics (MD) simulations of individual AQP0 tetramers in sphingomyelin (SM) membranes with low and high cholesterol concentration.
Density maps representing the localization of cholesterol around AQP0 over time were computed from simulations starting from unbiased cholesterol positions in membranes at the indicated SM:Chol ratios. After combining the four maps calculated individually for the four subunits of the tetramer, cholesterol densities were projected (blue areas) onto the surface of a single AQP0 monomer (white surface). Projections are shown for the S1 and S2 monomer surfaces, as defined in the representations to the right. Lipids seen in the electron crystallographic structures obtained in membranes at the respective SM:Chol ratios are displayed as sticks and labeled according to the electron crystallographic structures. Densities are contoured at 10σ for the 2:1 SM:Chol membrane and at 9σ for the 1:2 SM:Chol membrane. The density hotspot indicated with an asterisk coincides with the Chol3 position seen at the 1:2 SM:Chol ratio. See also Figure 5—figure supplements 1–3.
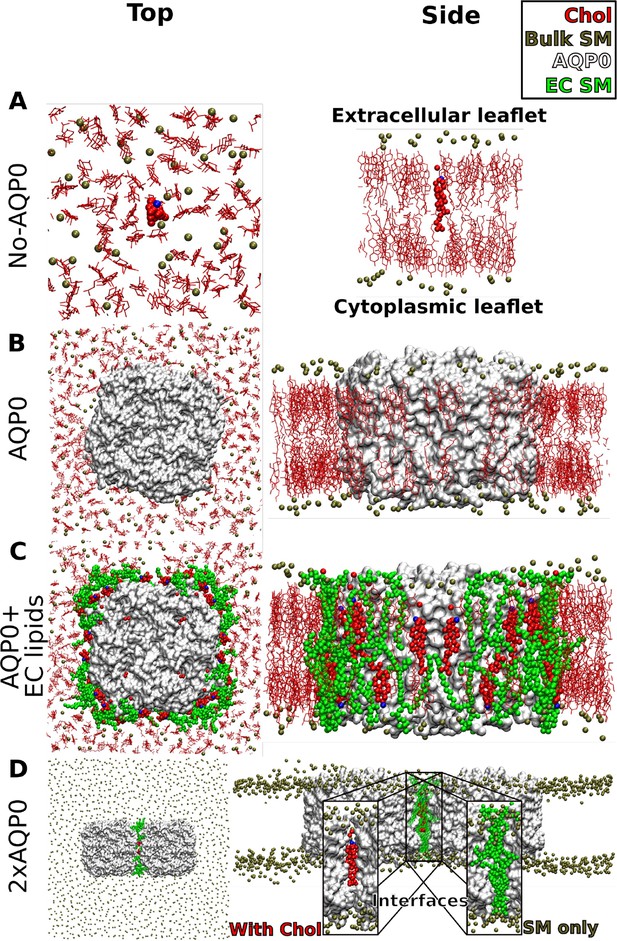
Molecular dynamics simulations of aquaporin-0 (AQP0) in sphingomyelin (SM)/cholesterol (Chol) membrane systems.
(A–D) Top views (left panels) and side views (right panels) of the simulated systems. AQP0 tetramers are shown in white surface representation. Lipids in positions seen in the electron crystallographic AQP01SM:2Chol structure are shown in VDW representation; SM in green and Chol in red and blue, respectively. Bulk cholesterol molecules are shown in red stick representation, whereas only the phosphorous atoms of bulk SM lipids are shown as dark tan spheres. The membranes were solvated by explicit water molecules (not shown for clarity). (A) In the ‘No AQP0’ systems used as control, lipid-only bilayers consisting of molar SM:Chol mixtures of 1:0, 2:1, and 1:2 (shown) were simulated. In each system, one cholesterol molecule was inserted in the position of the deep cholesterol seen in the AQP01SM:2Chol structure. (B) In the ‘AQP0’ system, a single AQP0 tetramer by itself without the annular lipids was inserted into membranes consisting of molar SM:Chol mixtures of 2:1 and 1:2 (shown). (C) In the ‘AQP0+EC lipids’ system, a single AQP0 tetramer with the lipids observed in the AQP01SM:2Chol structure was inserted into membranes consisting of molar SM:Chol mixtures of 1:0, 2:1, and 1:2 (shown). Note the deep cholesterols at the center. (D) In the ‘2×AQP0’ system, a pair of AQP0 tetramers was inserted into a pure SM membrane. The interfacial lipids between the pair of tetramers were either pure SM (inset ‘SM only’) from the AQP02SM:1Chol structure or a hybrid interface that replaces the two central SM molecules with the EC deep cholesterol molecules seen in the AQP01SM:2Chol structure (shown, inset ‘with Chol’).
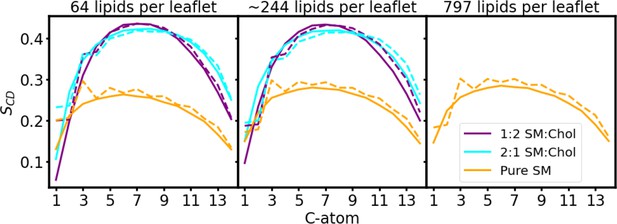
Deuterium order parameters for the membrane patches in equilibration.
Deuterium order parameters SCD for the hydrophobic acyl chains for the sphingomyelin (SM) lipids in a pure SM bilayer (Pure SM, orange), and in SM:Chol membranes at molar mixing ratios of 2:1 (2:1 SM:Chol, cyan) and 1:2 (1:2 SM:Chol, purple). The continuous line shows the values for the SN1 acyl chains and the dashed line shows the values for the SN2 acyl chains. The values correspond to the last 50 ns of simulation, independent of the patch size and total equilibration time. The three boxes correspond to the pure lipid membrane patches, used for the No-AQP0, AQP0, and 2×AQP0 simulations, respectively, before the protein was inserted in the latter two cases. Membrane patch size indicated on top of each box.
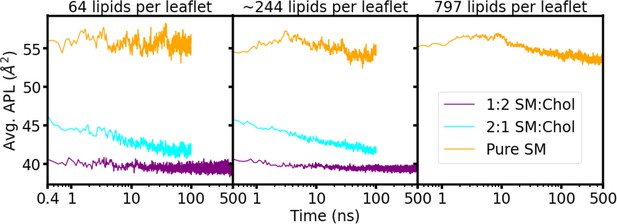
Average area-per-lipid (APL) for the membrane patches in equilibration.
Average APL as a function of time (log scale) is shown for the warm-up NPT simulations after removal of position restraints. The average APL value for the last 50 ns of simulation for the pure sphingomyelin (SM) systems were 55.5±0.7 Å2, 54.2±0.5 Å2, and 53.7±0.2 Å2, for the system with 64, ~244, and 797 lipids/leaflet, respectively.
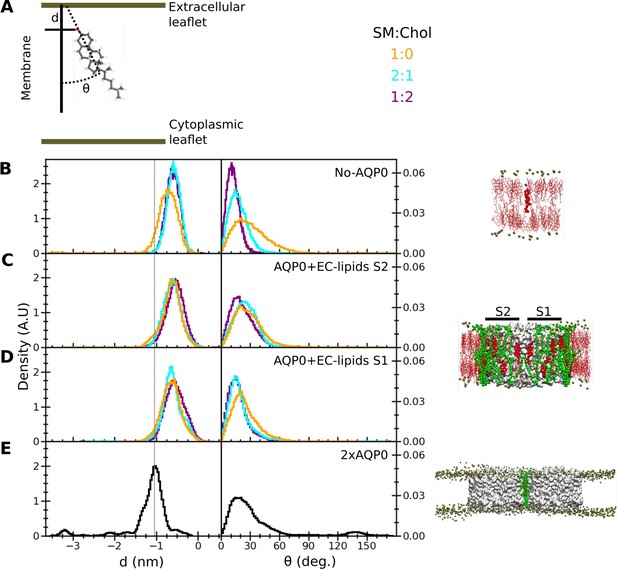
Insertion depth and orientation of a cholesterol at the interface between two aquaporin-0 (AQP0) tetramers.
(A) Schematic figure illustrating how the insertion depth, d, and orientation angle, θ, of the cholesterol were measured. The cholesterol insertion depth was defined as the distance in z direction of the cholesterol oxygen atom (red stick representation) from the center of mass of the phosphorus atoms of the nearby sphingomyelin (SM) molecules in the extracellular leaflet (top green horizontal line). The cholesterol orientation was defined as the angle between the membrane normal (simulation box z-vector) and the vector along the rings of the cholesterol molecule (black dashed line). (B–E) Right panels: The three different systems that were simulated, namely (B) ‘No AQP0’, a pure lipid membrane without AQP0, (C, D) ‘AQP0+EC lipids S2/1’, a membrane with one AQP0 tetramer surrounded by the annular lipids seen in the AQP01SM:2Chol structure, and (E) ‘2×AQP0’, a membrane containing a pair of AQP0 tetramers together with the lipids in between them from a hybrid AQP02SM:1Chol structure that replaces the two central SM molecules with the EC deep cholesterol molecules found in the AQP01SM:2Chol structure. Left panels: Graphs showing normalized histograms for the insertion depth, d, and orientation angle, θ, for the monitored deep cholesterol in membranes with different SM:Chol ratios (see color code in panel A), except for the 2×AQP0 system, which was only simulated in a pure SM membrane. For the simulations with one AQP0 tetramer, insertion and orientation were computed separately for the deep cholesterol located at surface S2 (C) and S1 (D). The vertical line indicates the most probable cholesterol position in the 2×AQP0 system (E). See also Figure 6—figure supplement 1.
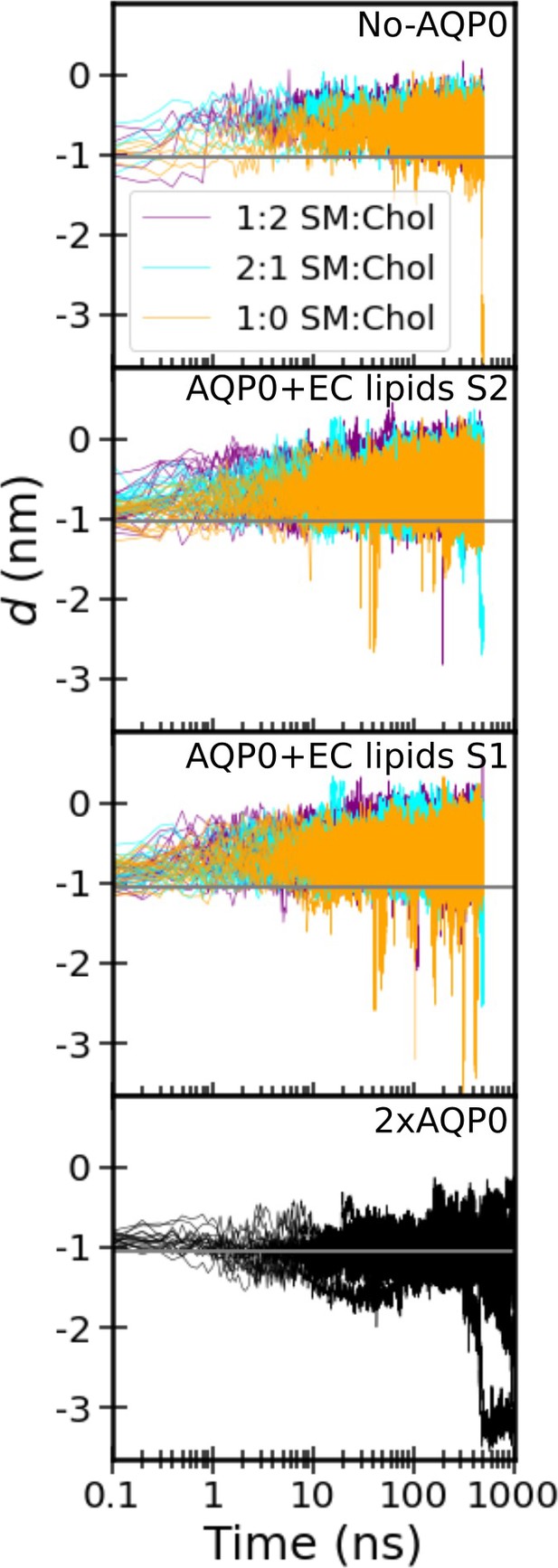
Time trace of the insertion depth of cholesterol.
Each panel corresponds to one of the four studied systems: ‘No AQP0’, a pure lipid membrane without AQP0, ‘AQP0+EC lipids S2/1’, a membrane with one AQP0 tetramer surrounded by the annular lipids seen in the AQP01SM:2Chol structure, and ‘2×AQP0’, a membrane containing a pair of AQP0 tetramers together with the lipids in between them from a hybrid AQP02SM:1Chol structure that replaces the two central sphingomyelin (SM) molecules with the electron crystallographic (EC) deep cholesterol molecules found in the AQP01SM:2Chol structure. Color indicates the different SM:Chol ratio and the different curves represent the different replicas for each condition. The horizontal gray line indicates the most probable cholesterol position in the 2×AQP0 system. Distributions shown in main Figure 6 were obtained from these time traces after discarding the first 100 ns that were considered equilibration time (300 ns for the 2×AQP0 system).
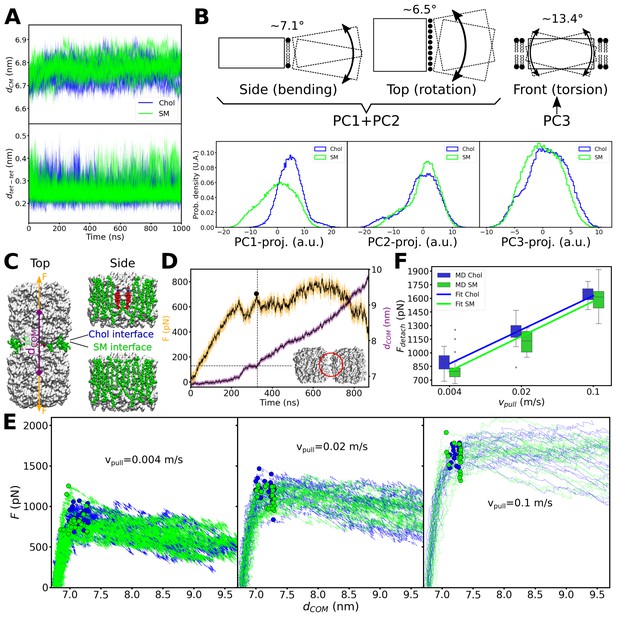
Equilibrium molecular dynamics (MD) simulations of pairs of associated aquaporin-0 (AQP0) tetramers and force-induced separation of two associated AQP0 tetramers.
(A) Distance between the centers of mass dCM (top) and minimum distance dtet-tet (bottom) between the pair of AQP0 tetramers during the 2×AQP0 simulations in equilibrium for the interface containing only sphingomyelin (SM) molecules (SM, green) and the interface containing the deep cholesterol (Chol, blue) (n=10 simulations for each case). (B) Principal component (PC) analysis of the relative movements between the two tetramers. Here, the motion of one of the tetramers (dashed-line rectangle) relative to the other tetramer (solid-line rectangle) was monitored. The three main PC accounted for 67.9% of the total relative motion between tetramers (PC1: 34.3%, PC2: 24.7%, and PC3: 8.9%). The schematic drawings illustrate the three main modes of motion: bending (depicted in the drawing as viewed from the side of the membrane), lateral rotation (depicted in the drawing as viewed from the top of the membrane), and torsion (depicted in the drawing as viewed from the side of the membrane with one tetramer in front of the other). Lipids at the interface between the two tetramers (first two panels) and lipids surrounding the two tetramers (last panel) are shown. PC1 plus PC2 capture the bending and rotation while PC3 corresponds to torsion. The histograms in the bottom panels show the projections of the MD trajectories onto the three main PC vectors, for the interfaces containing only sphingomyelin (SM, green) or containing the deep cholesterols (Chol, blue). The approximate angular extent for each of the modes, attributed to these projections, is indicated (in degrees). The distributions with and without cholesterol are very similar, except for PC1. Nevertheless, PC1 relates to a small angular variation (~7.1° bending together with ~6.5° rotation). (C) Two AQP0 tetramers (white surface) arranged as in two-dimensional (2D) crystals and embedded in a pure SM membrane were pulled apart by exerting a harmonic force F on them in the direction that connects their centers of mass (dCOM). Two different interfaces were studied: the ‘SM interface’ consisted solely of SM lipids (green spheres) as seen in the AQP02SM:1Chol structure, whereas the ‘Chol interface’ contained the deep cholesterols seen in the AQP01SM:2Chol structure. The reference position of the harmonic springs used to exert the force F was moved at a constant velocity Vpull/2. (D) Force (F) and distance (dCOM) time traces are shown for one of the simulations using a Vpull of 0.004 m/s. A Gaussian smoothing function (black continuous lines) was applied to the curves (yellow and purple). The detachment force (black circle) was computed as the highest recorded force when dCOM started to increase and below a cut-off distance dCOM of 7.3 nm (horizontal dashed line indicates the cut-off distance and the vertical dashed line indicated the time when this value was surpassed). Note that using different cut-off distances did not change the overall trend (see Figure 7—figure supplement 1). The inset shows an example of the arrangement of the tetramers at the moment of detachment. The red circle indicates the last contact. (E) Force–distance profiles for the two different interfaces are presented for the three indicated pulling velocities (n=20 for each case). Dots indicate the point of detachment. (F) Detachment force is presented as a function of the pulling rate for the two interfaces (box plots, n=20). A fit of the form Fdetach = A + B*log(Vpull) is shown to guide the eye with lines (Fdetach = [2164+232*log(Vpull)] for the ‘Chol’ interface and Fdetach = [2116+239*log(Vpull)] for the ‘SM’ interface). p-Values comparing the two datasets, separately for each pulling velocity, are 0.022 (Vpull = 0.004 m/s), 0.015 (Vpull = 0.02 m/s), and 0.262 (Vpull = 0.1 m/s) (Mann–Whitney U test). Furthermore, a two-way ANOVA test, considering the three pulling velocities at once, retrieved a p-value of 0.003 for the lipid interface change (i.e. Chol vs. SM). See also Figure 7—figure supplement 1.
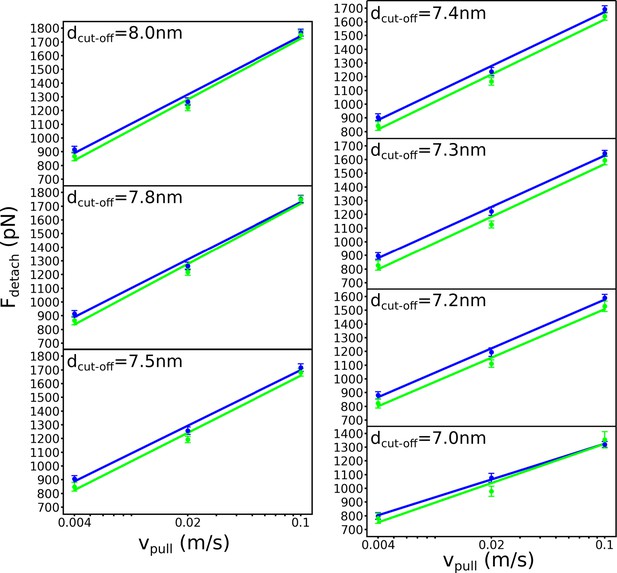
Detachment force versus pulling rate for different dcut-off criteria.
The cut-off value chosen to define tetramer separation does not alter the conclusion that the presence of deep cholesterol at the interface (blue) results in a more stable association between the tetramers (i.e. requiring a high detachment force) than when the interface only contains sphingomyelin (green) (n=20 for each case).
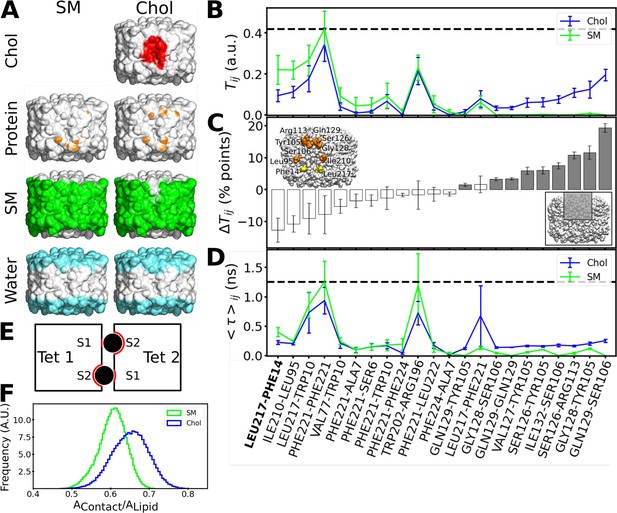
Interactions formed between adjacent aquaporin-0 (AQP0) tetramers and lipid-AQP0 surface complementarity.
(A) Equilibrium molecular dynamics (MD) simulations were performed with pairs of AQP0 tetramers without cholesterol (left panels) or with cholesterol (right panels) at the interface. Density maps were calculated for different components next to the AQP0 surface over time and these maps were projected onto the surface of one of the AQP0 tetramers to represent their localization. For clarity, the second tetramer, which would be in front of the shown tetramer, is not shown. The density displayed at arbitrary density units is color-coded and shows the position of cholesterol (red), protein, i.e., the neighboring AQP0 tetramer (brown), sphingomyelin (green), and water (cyan). (B) Tij is defined as the fraction of time during which residues (i, j) from opposite tetramers are in contact. This quantity was extracted from the equilibrium simulations for the pure sphingomyelin interface Tij(SM) and for the interface containing cholesterol Tij(Chol). Tij = 0 means that i and j were never in contact and Tij = 1 means that they were always in contact. (C) The pairwise difference ΔTij = Tij(Chol) - Tij(SM) is shown, discarding insignificant changes (ΔTij<1 percentage, %, points). Accordingly, a value of ΔTij>0 (ΔTij<0) corresponds to protein–protein contacts that were more often observed in the simulations with cholesterol (sphingomyelin). For instance, the residue pair Gln129–Ser106 was observed almost 20 percentage points more time in the simulations with cholesterol. The color of the bars indicates the location of the residues (gray: inner part of the extracellular leaflet, i.e., where deep cholesterol resides; white: the rest of the interfacial protein surface, see inset at lower right). Residues involved in a high ΔTij>10 percentage points are highlighted in orange in the inset (yellow for the contact Leu217–Phe214 observed in the electron crystallographic structure). (D) The average duration for every established protein–protein contact, <τ>ij, is also displayed for the two different lipid interfaces. In (B) and (D), the horizontal dashed lines indicate the highest value observed for all possible residue pairs. Contacts observed in the electron crystallographic structures are highlighted in bold letters. In B–D, the avg ± s.e.m. is presented (n=20, i.e. 10 independent simulation times with two symmetric monomeric interfaces). (E) The schematic drawing depicts a top view of the two associated AQP0 tetramers (squares, ‘Tet 1’ and ‘Tet 2’) with the two central lipids sandwiched between them (black circles). The other lipids at the interface are not shown for clarity. The respective monomer surfaces S1 and S2 are indicated. The region of the surface of the two tetramers that is in total covered by a lipid, AContact, (red line) was normalized by the surface area of the lipid, ALipid (here corresponding to the perimeter of the circles). This ratio gives a measure of the surface complementarity between the tetramers and the sandwiched lipids, i.e., the higher the value of AContact/ALipid the more the two surfaces complement each other. (F) Normalized histograms of AContact/ALipid obtained from the equilibrium MD simulations are shown for the central sphingomyelin (SM) and cholesterol (Chol). See also Figure 8—figure supplements 1–3.
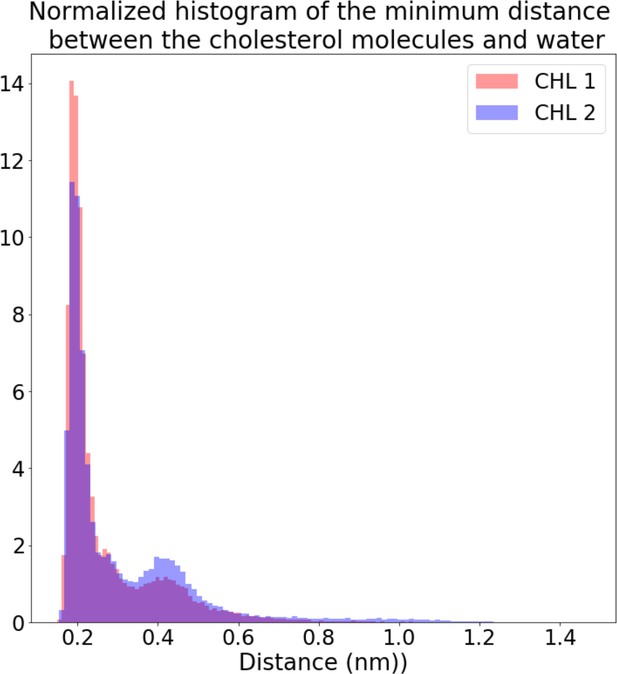
Distance from each deep cholesterol OH group to the nearest water molecule.
Minimum distance between the hydroxyl oxygen atom in each deep cholesterol molecule and the closest water molecule in the 2×AQP0 simulations in equilibrium shows that they were nearly always in contact.
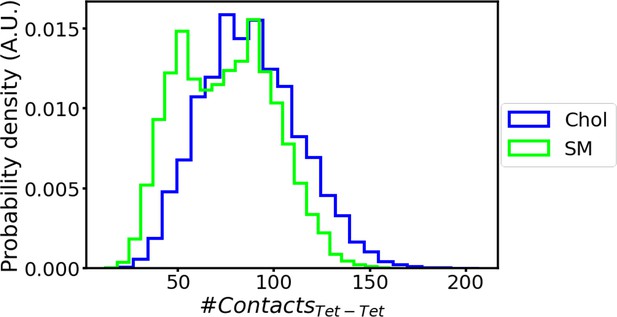
Contacts between tetramers in 2×AQP0 simulations.
Number of contacts between the two tetramers during molecular dynamics (MD) simulations of the 2×AQP0 system in equilibrium for the interface containing only sphingomyelin (SM) and the interface containing deep cholesterols (Chol). The distributions show more protein contacts for the interface with cholesterol in accordance with Figure 8A–C.
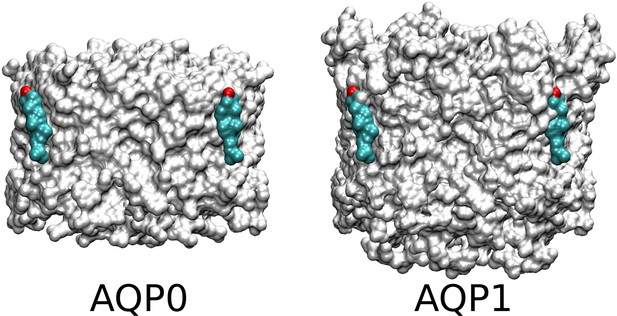
Surfaces of aquaporin-0 (AQP0) and AQP1.
The position of Chol1 seen in the AQP0 structures is indicated at the left and overlapped with the AQP1 structure at the right, which results in steric clashes. The difference in the lateral surfaces of AQP0 and AQP1 may result in a greater association of cholesterol in the Chol1 position in the case of AQP0.
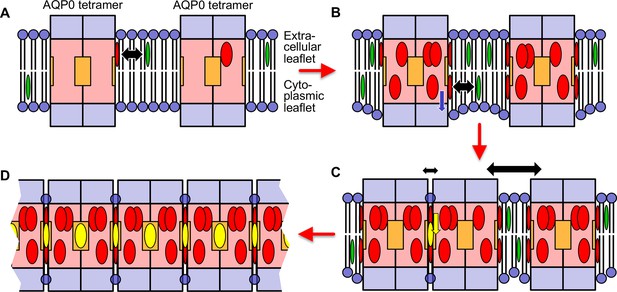
Proposed model for how an increasing cholesterol concentration drives aquaporin-0 (AQP0) two-dimensional (2D) array formation in the native lens membrane.
(A) At a low cholesterol concentration, AQP0 tetramers are mostly surrounded by phospholipids and sphingomyelin. Free cholesterol in the membrane (green ovals) only associates with the highest affinity cholesterol-binding sites. Cholesterols occupying these peripheral binding sites are shown as red ovals and the black double-headed arrow indicates the transient nature of this interaction. The deep cholesterol-binding sites (orange squares) are not occupied. (B) With increasing cholesterol concentration, more cholesterols associate with the AQP0 surface. These cholesterols cause the interacting lipids in the cytoplasmic leaflet to move out from the bilayer center (blue arrow), resulting in the annular lipid shell that has a bigger hydrophobic thickness than the surrounding membrane, creating a hydrophobic mismatch that results in membrane deformation. (C) To minimize hydrophobic mismatch, AQP0 tetramers cluster. Cholesterol in between adjacent tetramers can move into the deep binding sites (yellow arrow) and cholesterol occupying deep binding sites (yellow ovals) act as glue that increases that association of the adjacent tetramers (indicated by the small double-headed black arrow) as compared to adjacent tetramers that do not sandwich a deep-binding cholesterol (indicated by the large double-headed black arrow). Clustering of proteins to minimize hydrophobic mismatch and stabilization by deep cholesterol-mediated protein–protein interactions may be the basis for the formation of transient lipid rafts. (D) Each AQP0 tetramer has four deep cholesterol-binding sites. As a result of the avidity effect, AQP0 can form large and stable 2D arrays.
Tables
Internal phase residuals of all rectangular plane groups for an image of an aquaporin-0 (AQP0) crystal in a pure cholesterol membrane.
Space group | Phase residual (degrees)* | Number of comparisons | Target residual (degrees)† |
---|---|---|---|
p1 | 12.5 ‡ | 240 | |
p2 | 33.8 | 120 | 17.8 |
p12b | 14.5 § | 108 | 12.9 |
p12a | 31.7 | 109 | 12.9 |
p121b | 83.7 | 108 | 12.9 |
p121a | 80.0 | 109 | 12.9 |
c12b | 14.5 § | 108 | 12.9 |
c12a | 31.7 | 109 | 12.9 |
p222 | 27.0 | 337 | 14.4 |
p2221b | 60.0 | 337 | 14.4 |
p2221a | 68.2 | 337 | 14.4 |
p22121 | 69.7 | 337 | 14.4 |
c222 | 27.0 | 337 | 14.4 |
p4 | 26.3 | 352 | 14.3 |
p422 ¶ | 24.5 | 791 | 13.3 |
p4212 | 62.6 | 791 | 13.3 |
-
Internal residuals were determined using the ALLSPACE program (Valpuesta et al., 1994) using spots from IQ1 to IQ5 to a resolution of 6 Å.
-
*
Phase residual versus other spots (90° random).
-
†
Target residual based on the statistics taking Friedel weight into account.
-
‡
Note that no phase comparison is possible in space group p1, so that the listed numbers are theoretical phase residuals based on the signal-to-noise ratio of the observed diffraction spots in the Fourier transform.
-
§
Within 5° of target residual.
-
¶
The symmetry indicated in bold was used to calculate the final 2D projection map.
Phase residuals for the merging of 15 images of aquaporin-0 (AQP0) two-dimensional (2D) crystals in pure cholesterol membranes.
Plane group symmetry | p422 |
---|---|
Unit cell dimensions | a=65.5 Å, b=65.5 Å, and γ=90° |
Number of processed electron micrographs | 15 |
Resolution limit for merging | 3.0 Å |
Number of phases | 2773 |
Phase residuals in resolution bins | |
1000.0 Å – 11.6 Å | 21.7° |
11.6 Å – 8.2 Å | 31.2° |
8.2 Å – 6.7 Å | 34.1° |
6.7 Å – 5.8 Å | 47.2° |
5.8 Å – 5.2 Å | 43.7° |
5.2 Å – 4.7 Å | 50.4° |
4.7 Å – 4.4 Å | 58.0° |
4.4 Å – 4.1 Å | 62.3° |
4.1 Å – 3.9 Å | 62.3° |
3.9 Å – 3.7 Å | 64.0° |
3.7 Å – 3.5 Å | 70.8° |
3.5 Å – 3.4 Å | 69.1° |
3.4 Å – 3.2 Å | 71.0° |
3.2 Å – 3.1 Å | 88.0° |
3.1 Å – 3.0 Å | 86.0° |
Overall | 54.5° |
Statistics of electron crystallographic structures for aquaporin-0 (AQP0) in sphingomyelin/cholesterol membranes.
2SM:1Chol | 1SM:2Chol | |
---|---|---|
Crystal parameters | ||
Space group | p422 | |
Unit cell constants | a=b=65.5 Å, γ=90° | |
Assumed thickness | 200 Å | |
Electron diffraction | ||
Diffraction patterns | 214 | 241 |
(0°:16; 20°:19; 45°:77; 60°:84; 65°:17; 70°:1) | (0°:15; 20°:18; 45°:51; 60°:86; 65°:30; 70°:41) | |
Maximum tilt angle | 71.72° | 72.35° |
Upper resolution limit for merging | 2.3 Å | 2.3 Å |
RFriedel | 0.149 | 0.138 |
Rmerge | 0.216 | 0.199 |
Observed amplitudes | 122,501 | 127,703 |
Unique reflections | 16,437 | 17031 |
Minimum of Fobs/Sigmaobs | 1.33 | 1.33 |
Fourier space sampled | 87.0% | 90.7% |
Multiplicity | 6.2 | 6.3 |
(2.3 Å: 4.9) | (2.3 Å: 4.6) | |
Crystallographic refinement | ||
Resolution range | 13.8–2.35 Å | 11.8–2.35 Å |
Rwork/Rfree* | 0.260/0.286 | 0.262/0.287 |
Atoms | ||
Protein | 1663 | 1663 |
Lipids | 336 | 341 |
Water | 11 | 11 |
RMS deviation | ||
Bond length (Å) | 0.008 | 0.005 |
Bond angle (degrees) | 1.096 | 0.842 |
Model validation | ||
Clashscore | 8.86 | 6.98 |
MolProbity | 1.79 | 1.79 |
Rotamer outliers (%) | 0.00 | 0.60 |
C-beta deviation | 0 | 0 |
Ramachandran plot (%) | ||
Disallowed | 0.00 | 0.00 |
Allowed | 4.59 | 5.96 |
Favored | 95.41 | 94.04 |
-
*
Rfree was calculated from randomly selected 10% of total reflections, and Rwork was calculated from the remaining 90% of reflections.
Summary of the simulations.
Scheme | Protein system | Membrane system | Pulling rate (m/s) | Number of replicas | Length per replica (ns) |
---|---|---|---|---|---|
Equilibrium simulations | No AQP0 | Pure SM | N/A | 5 | 500 |
2:1 SM:Chol | |||||
1:2 SM:Chol | |||||
One AQP0 tetramer without EC lipids | Pure SM | 5 | 500 | ||
2:1 SM:Chol | |||||
1:2 SM:Chol | |||||
One AQP0 tetramer with EC lipids | 2:1 SM:Chol | 5 | 500 | ||
1:2 SM:Chol | |||||
Two AQP0 tetramers with deep cholesterol | Pure SM | 10 | 1000 | ||
Two AQP0 tetramer without deep cholesterol | Pure SM | ||||
Force probe | Two AQP0 tetramers with deep cholesterol | Pure SM | 0.1 | 20 replicas for each velocity | ~55 |
0.02 | ~230 | ||||
0.004 | ~900 | ||||
Two AQP0 tetramers without deep cholesterol | Pure SM | 0.1 | 20 replicas for each velocity | ~55 | |
0.02 | ~230 | ||||
0.004 | ~900 |