When abstract becomes concrete, naturalistic encoding of concepts in the brain
Figures
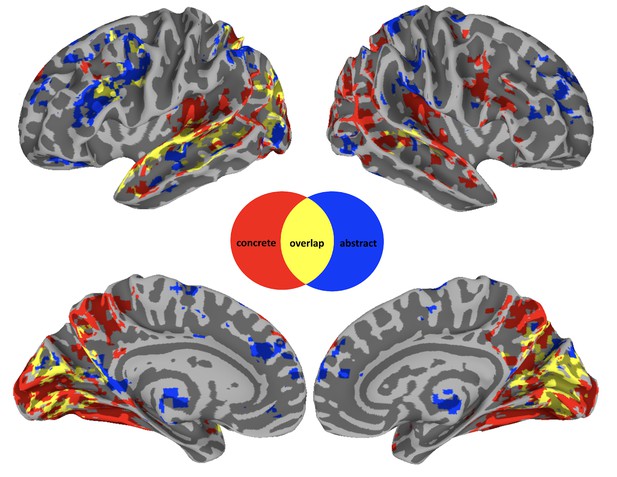
Neurobiology of conceptual processing across contexts.
Colored regions show group-level results from a linear mixed effect model and subsequent general linear tests contrasting activity for concrete (red) versus abstract (blue) modulation at each of 20 timepoints after word onset. Overlapping regions (yellow) indicate a concrete and abstract difference at one of these timepoints. Results are thresholded and corrected for multiple comparisons at α=0.01 and displayed with a cluster size ≧ 20 voxels.
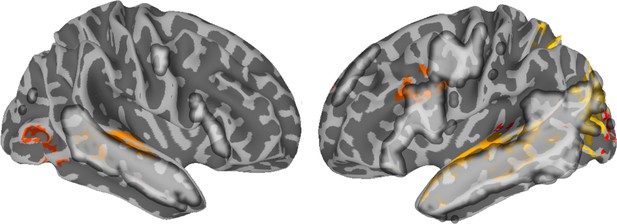
Comparison between overlap and language regions.
Comparison between the language network (outline mask extracted from Neurosynth) and overlap activity for both concrete and abstract words across context. Results are thresholded and corrected for multiple comparisons at α=0.01 and displayed with a cluster size ≧ 20 voxels.
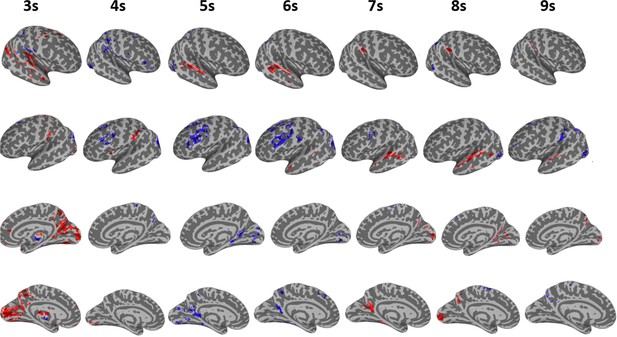
Time course of activation.
Voxel activation based on BOLD signal (seconds from word-onset), correlated with abstract (blue) and concrete (red). Activation for concrete and abstract concepts both started at 3 s. Processing of concrete concepts correlated more with activity in vision and motor systems, while processing of abstract concepts correlated with activation in the thalamus and somatosensory cortex. Activation for concrete concepts then spread into the superior temporal lobe (bilaterally), during 3–8 s. On the other hand, activation for abstract concepts started in the somatosensory cortex, thalamus. Activation then spreads into the anterior cingulate cortex (5 s) and spreads into medial prefrontal (LH) areas as well as Inferior frontal and angular gyrus (LH) during 5–9 s.
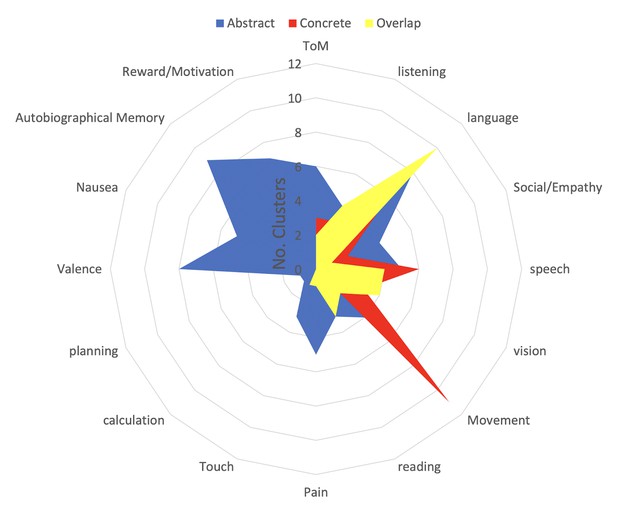
Meta-analytic description of conceptual processing across contexts.
We used the Neurosynth meta-analysis package to find the terms associated with the centers of mass for each concrete (red), abstract (blue), and overlap (yellow) cluster from Figure 1. Numbers refer to the number of activation clusters associated with each meta-analytic term. There were significantly more concrete than abstract clusters for the term ‘Movement’ (p<0.001), whereas there were more abstract compared to concrete clusters for ‘Autobiographical Memory,’ ‘Nausea,’ ‘Pain,’ ‘Theory of Mind,’ and ‘Valence’ (all p’s <0.05). The term 'language' was significantly more associated with overlap clusters compared to concrete (p<0.001) and abstract clusters (p=0.045).
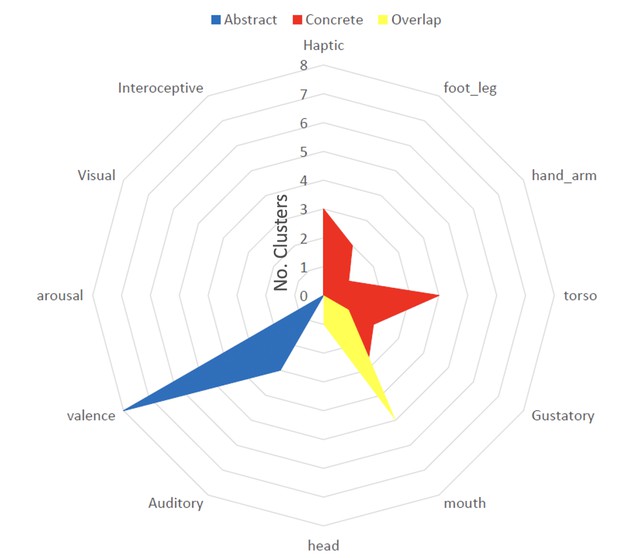
Peak and valley analysis results for understanding conceptual processing across contexts.
We extract the type of information processed in each activation cluster by looking at experience-based features of movie words that are aligned with significantly more peaks than valleys (see Figure 1). Words highly rated on the sensorimotor dimensions ‘Haptic,’ ‘Hand_Arm,’ and ‘Torso’ were significantly more associated with concrete clusters (red, all p’s <0.05), ‘Valence’ with abstract clusters (blue, p<0.001) and ‘Mouth’ with overlap clusters (yellow, p’s <0.05). For some features/terms, there were never significantly more words highly rated on that dimension occurring at peaks compared to valleys, so they do not have any significant clusters.
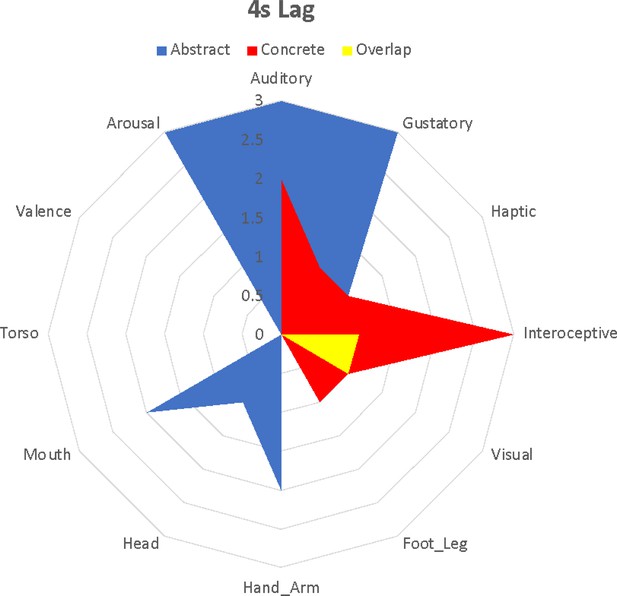
Peak and valley analysis for a 4 s lag.
A Kruskal-Wallis test shows that the distribution between sensorimotor and interoceptive/emotional dimensions for concrete and abstract words is significantly different from a 5 s H(2)=4,8, p=0.03, and 6 s (H(2)=5.3, p=0.02) lag.
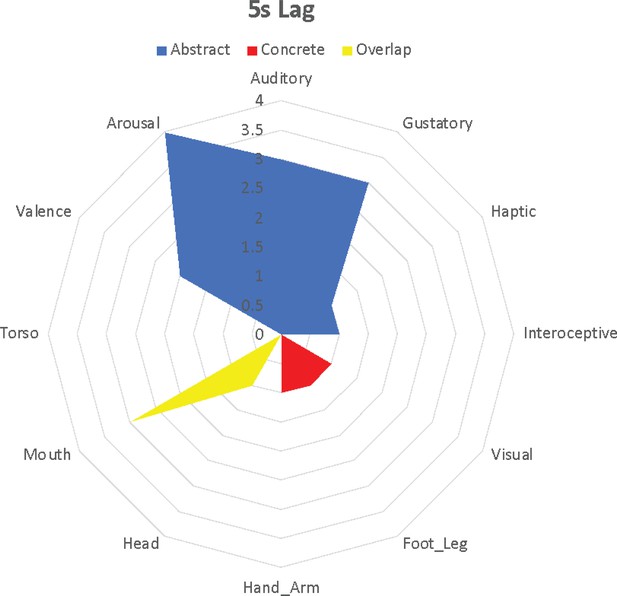
Peak and valley analysis at the individual participant level (5s lag).
Peak and valley Analysis for a 5 s lag. Internal dimensions Valence and Arousal are significantly more associated with peaks in abstract compared to concrete clusters (Valence: H(2) = 5.8, p=0.02; Arousal: H(2) = 6.7, p=0.01). Conversely, concrete clusters are more associated with sensorimotor dimensions (Hand_Arm, Foot_Leg and Visual) – though not significantly so. Overlap is significantly more associated with ‘Mouth’ (H(2) = 6.2, p=0.2).
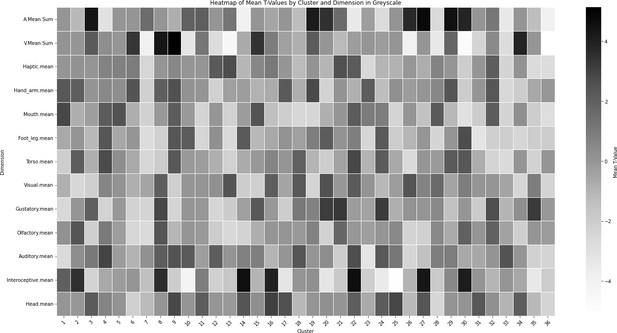
Peak and valley analysis after averaging results for individual participants for abstract clusters only.
Results show that the peaks in the BOLD response are most correlated with dimensions ‘A.Mean.Sum (Arousal),’ ‘V.Mean.Sum (Valence),’ and ‘Interoceptive.mean (Interoception).’
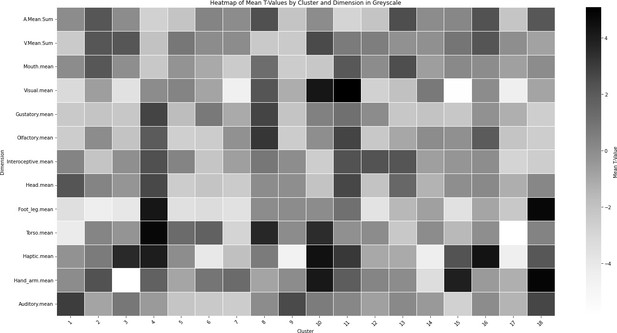
Peak and valley analysis after averaging results for individual participants for concrete clusters only.
Results show that the peaks in the BOLD response are most correlated with dimensions ‘Visual.mean (Visual),’ ‘Foot_leg.mean (Foot_Leg),’ ‘Torso.mean (Torso),’ ‘Haptic.mean (Haptic),’ and ‘Hand_arm.mean (Hand_arm).’
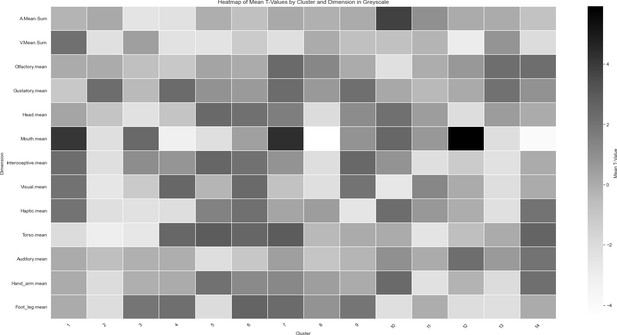
Peak and valley analysis after averaging results for individual participants for overlap clusters only.
Results show that the peaks in the BOLD response are most correlated with the dimension ‘Mouth.mean’ (Mouth).
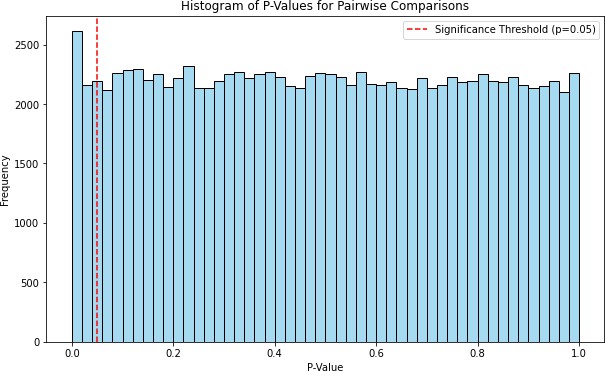
Test of nonlinearity for peak and valley analysis.
p-values for all possible pairwise correlations of BOLD responses related to each feature. Only 3.85% of responses were significantly different.
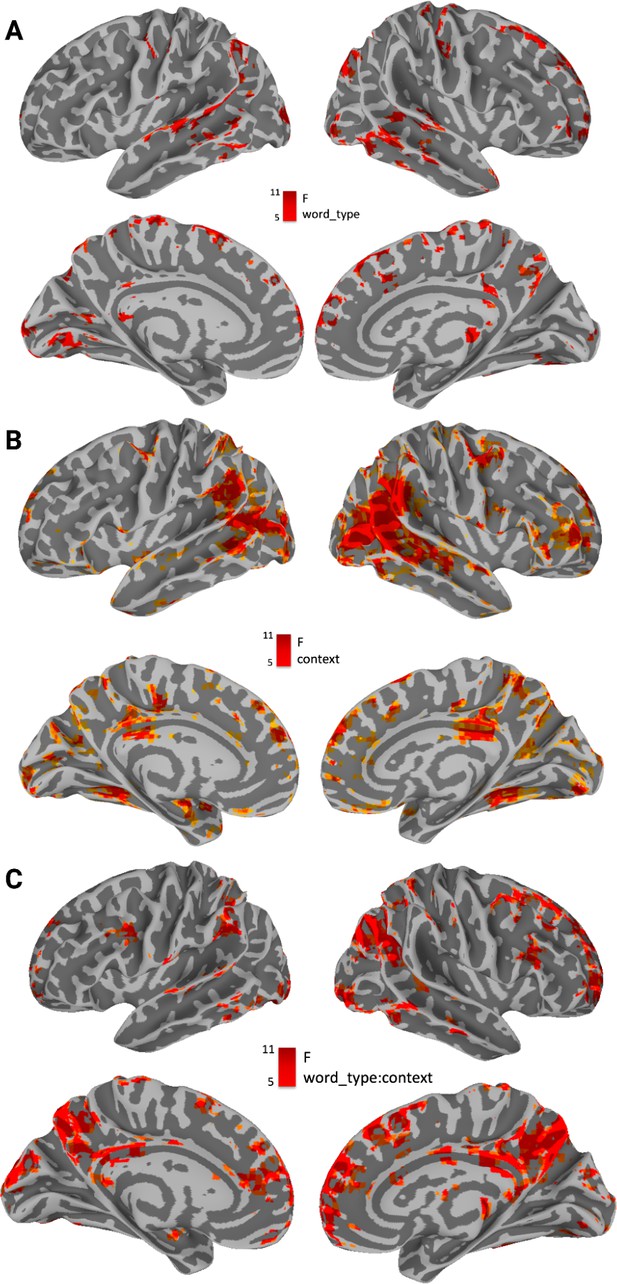
Main effects of word_type, context, and their interaction.
(A) Main effect of word-type. Most significantly modulated areas include superior temporal sulcus, superior temporal gyrus and middle temporal gyrus (bilateral), angular gyrus (bilateral), the central sulcus and precentral and postcentral gyrus (right hemisphere), as well as lateral and medial frontal cortices and the occipital lobe. (B) Main effect of context. Most significantly modulated areas include the intersection between posterior temporal and occipital lobe, the Precuneus, Middle Prefrontal Cortex, as well as Angular Gyrus, and right inferior frontal gyrus. (C) Interaction between context (high/low) and word type (abstract/concrete). Most significantly modulated areas include the Precuneus, Middle Prefrontal Cortex as well as Middle Frontal Gyrus, Angular Gyrus, and Posterior Cingulate Cortex. These correspond to the nodes of the default mode network, as well as areas commonly associated with semantic control. This was confirmed by using the neurosynth decoder on the unthresholded brain image - top keywords were ‘Semantic Control’ and ‘DMN.’ All displayed results are thresholded and corrected for multiple comparisons at α=0.01 and displayed with a cluster size ≧ 20 voxels.
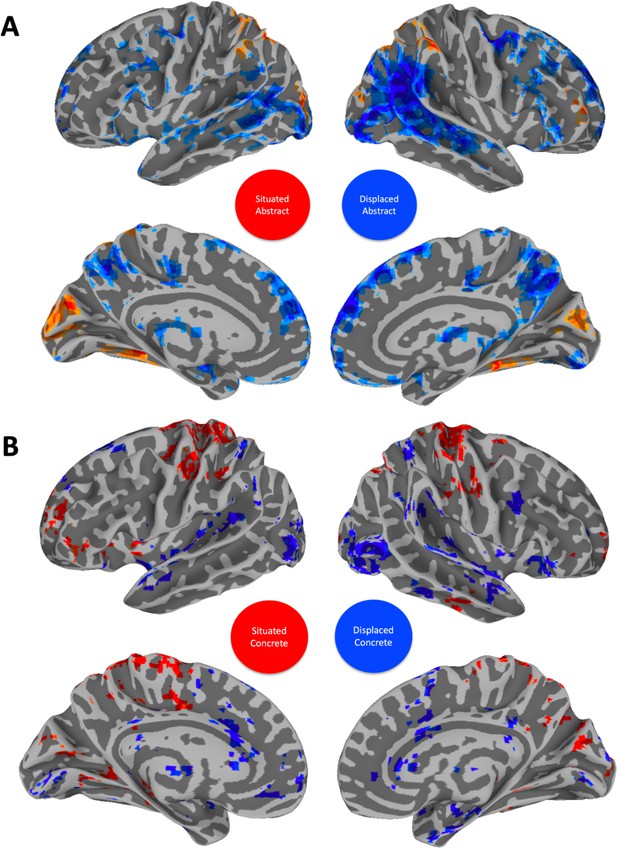
Contrasts between situated abstract and displaced abstract (A) as well as situated concrete and displaced concrete (B).
The displaced concrete activation mask was later correlated with abstract processing across context (see Figure 6). The situated abstract activation mask was later correlated with concrete processing across context (see Figure 6). Nodes of the default mode network (DMN) are especially active in the displaced condition for both abstract and concrete words. Visual and sensorimotor areas are especially active in situated conditions for both abstract and concrete words. Results are thresholded and corrected for multiple comparisons at α=0.01 and displayed with a cluster size ≧ 20 voxels.
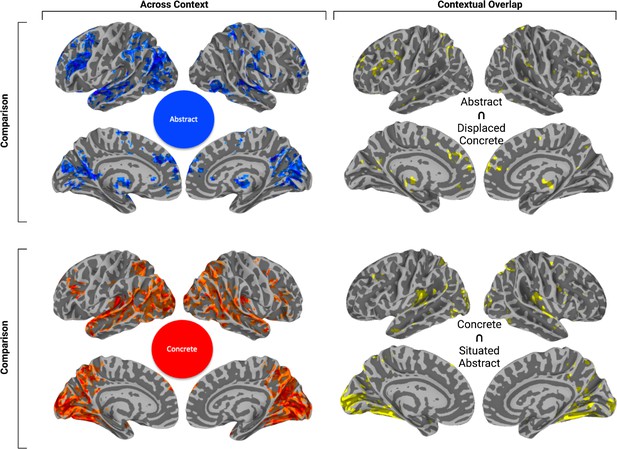
Spatial overlap between thresholded statistical brain images of concrete and abstract conceptual processing obtained from the original analysis across contexts situatedness/displacement contrasts (on the left).
Original brain maps of our analysis across context are split into abstract (top) and concrete (bottom) on the right. The overlap between displaced concrete and abstract was (r(72,964) = 0.49, p<0.001), the overlap between situated abstract and concrete was (r(72,964)=0.64, p<0.001). All maps were thresholded at α=0.01 with a cluster size ≧ 20 voxels.
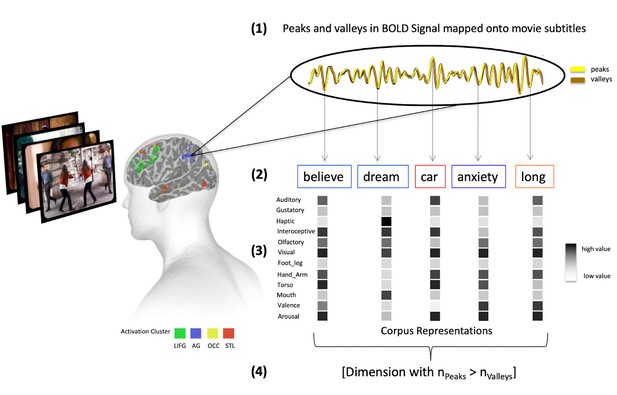
Overview of the peak and valley analysis method.
First, we average the fMRI time series for each participant, for each abstract, concrete, and overlap cluster of activity from Figure 1. Then we label peaks and valleys in these (1) and map them onto word on- and off-set times (2). Finally, we estimate sensorimotor as well as valence and arousal representations for each abstract (blue frame) and concrete word (red frame) (3) and determine which dimensions are associated with significantly more peaks than valleys across participants in each cluster using a Kruskal-Wallis test (4).
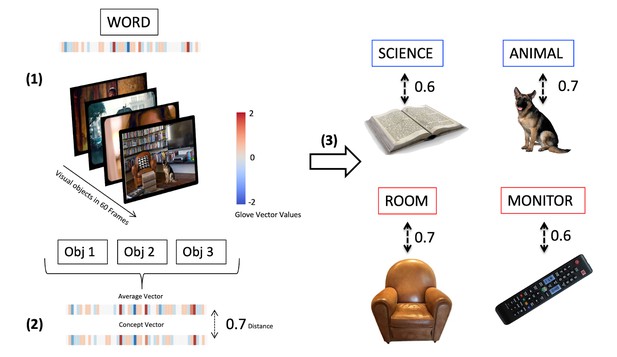
Method for estimating contextual situatedness for each concrete and abstract word to model context-dependent modulation of conceptual encoding.
We use visual recognition models for automatically extracting labels that were visually present in the scene (60 frames, ~2 s) before a given word was mentioned in the movie (1). We then correlate an average GloVe Vector embedding of all these labels with a GloVe Vector embedding of that word to estimate how closely related the labels of objects in the scene are to the word (2). Displayed are four randomly extracted measures of situated abstract (blue frame) and concrete (red frame) words (3) together with the objects that were visually present in the scene.
Tables
Complete Meta-analytic description of clusters.
Dimension | Abstract Clusters(N=35) | Concrete Clusters(N=20) | Kruskal-Wallis Test |
---|---|---|---|
Autob. Memory | 9 | 1 | H(2)=4, P=0.05 |
Valence | 8 | 0 | H(2)=5.6, P=.01 |
Theory of Mind | 6 | 0 | H(2)=4.8, P=0.03 |
Nausea | 6 | 0 | H(2)=4.8, P=0.03 |
Pain | 5 | 0 | H(2)=4, P=0.05 |
Movement | 2 | 11 | H(2)=12.4,P<.001 |
Social/Empathy | 4 | 1 | H(2)=0.7, P=0.42 |
Touch | 3 | 0 | H(2)=1.8, P=0.18 |
Speech | 5 | 6 | H(2)=1.1, P=0.29 |
Language | 8 | 4 | H(2)=0.08, P=0.78 |
Reading | 3 | 0 | H(2)=1.8, P=0.18 |
Reward/Motivation | 7 | 3 | H(2)=0.24, P=0.63 |
Vision | 0 | 3 | H(2)=5.3, P=0.02 |
Listening | 3 | 2 | H(2)=0.02, P=0.88 |
Planning | 1 | 0 | H(2)=1.7, P=0.19 |
Calculation | 1 | 0 | H(2)=1.7, P=0.19 |
Peak and valley results between concrete and abstract activation clusters.
Dimension | Abstract clusters(N=35) | Concrete clusters(N=20) | Kruskal-Wallis test |
---|---|---|---|
Valence | 9 | 1 | H(2)=4, p=0.05 |
Interoceptive | 8 | 0 | H(2)=5.6, p=0.01 |
Arousal | 6 | 0 | H(2)=4.8, p=0.03 |
Auditory | 6 | 0 | H(2)=4.8, p=0.03 |
Visual | 5 | 0 | H(2)=4, p=0.05 |
Head | 2 | 11 | H(2)=12.4, p<0.001 |
Haptic | 0 | 3 | H(2)=5.3, p=0.02 |
Foot_Leg | 0 | 2 | H(2)=3.5, p=0.06 |
Hand_Arm | 0 | 1 | H(2)=1.7, p=0.19 |
Torso | 0 | 4 | H(2)=7.3, p=0.01 |
Gustatory | 0 | 2 | H(2)=3.5, p=0.06 |
Mouth | 0 | 3 | H(2)=5.3, p=0.02 |
Head | 0 | 0 | / |