Basement membranes are crucial for proper olfactory placode shape, position and boundary with the brain, and for olfactory axon development
Figures
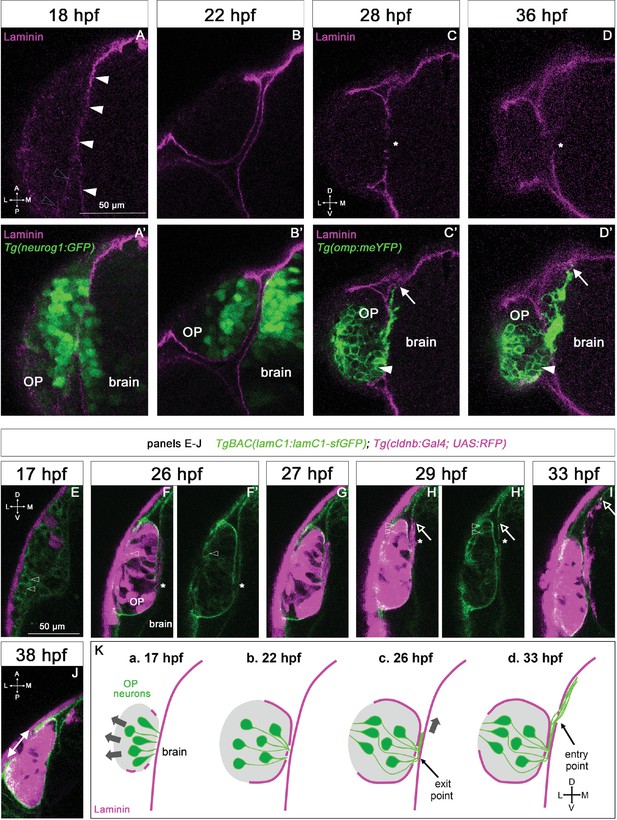
Expression profile of Laminin in relation with the development of the olfactory system.
(A–D) Immunostaining for Laminin magenta on Tg(neurog1:GFP) embryos (green) at 18 and 22 hpf (A-B’, dorsal views), and on Tg(omp:meYFP) embryos (green) at 28 and 36 hpf (C-D’, frontal views). In A, white arrowheads = BM like Laminin staining around the brain, grey arrowheads = spotty Laminin accumulation around the OP. In C, D, asterisks = interruptions in the OP’s and brain’s BM where the YFP+ axons exit the OP and enter the brain. arrows = distalmost extremity of the YFP+ axon bundle, which is in close contact with the internal side of the brain’s BM. In (C’, D’), white arrowheads = ventro-medial unipolar neurons labelled by the Tg(omp:meYFP) line (see Miyasaka et al., 2005). All the images are single z-sections. (E–J) Images extracted from confocal live imaging on TgBAC(lamC1:lamC1-sfGFP); Tg(cldnb:Gal4; UAS:RFP) embryos (frontal view, except for J), dorsal view. LamC1-sfGFP expression in green, and RFP expression (OP cells and peridermal skin cells) in magenta. Arrowheads = OP cells with cytoplasmic LamC1-sfGFP accumulation. Asterisks in F and H=axon exit point and entry point, respectively. Arrows in H and I=distalmost extremity of the RFP+ axon bundle, located close to the brain’s BM. In J, double headed arrow = gap in the LamC1-sfGFP observed at the interface with the periderm, where the nostril orifice opens in the skin, as previously reported (Baraban et al., 2023). All the images are single z-sections. (K) Schematic representation of Laminin-containing BM (magenta) assembly during OP coalescence (a, b) of the formation of the exit/entry points, which often appear as zones with several, small BM interruptions (b, d) and the associated axonal behaviours: retrograde axon extension and lateral movement in the OP (a, b, grey arrows), growth as a fasciculated bundle, initially between the BMs of the OP and the brain (c), and then migration of the axonal tips along the internal side of the brain’s BM (d) Scale bar: 50 µm.
BM assembly around the brain and the OP during OP coalescence, related to Figure 1.
Same embryo as the z-section shown in Figure 1J. Confocal live imaging performed on a TgBAC(lamC1:lamC1-sfGFP);Tg(cldnb:Gal4;UAS:RFP) embryo in which Laminin γ1-sfGFP is expressed under the control of its own promoter (green), and OP and peridermal skin cells are labelled in with cytoplasmic RFP (magenta). Pink arrowheads indicate the BM surrounding the brain, which is already assembled and continuous when the movie starts (at 17 hpf), while the BM surrounding the OP (shown with yellow arrowheads) starts to assemble later, during OP coalescence. Grey dots indicate mesenchymal cells exhibiting Laminin γ1-sfGFP cytoplasmic expression and migrating around the OP. Yellow dots indicate RFP-positive OP cells also showing Laminin γ1-sfGFP cytoplasmic expression. Only the left side of the embryo is shown. One z-section, dorsal view, anterior to the top and lateral to the left, delta t = 10 min. Scale bar: 25 µm.
Formation of the exit and entry points in the BMs surrounding the OP and the brain, related to Figure 1.
Same embryo as the z-sections shown in Figure 1E-I and in Figure 1—video 4. Confocal live-imaging performed on a TgBAC(lamC1:lamC1-sfGFP);Tg(cldnb:Gal4;UAS:RFP) embryo in which Laminin γ1-sfGFP is expressed under the control of its own promoter (green), and OP and peridermal skin cells are labelled in with cytoplasmic RFP (magenta). The yellow arrowhead shows the exit point, which appears as a small interruption (or several small interruptions, see also Figure 1—video 4) in the BM of the OP resulting from its incomplete assembly in this specific area. The blue arrowhead indicates the opening of the entry point (which can also be formed by several gaps in somes instances, see also Video 4) in the already assembled BM of the brain, which is concomitant with the arrival of the RFP-positive axons. Pink dots indicate brain cells expressing Laminin γ1-sfGFP cytoplasmic expression. Only the left side of the embryo is shown. One z-section, frontal view, dorsal to the top and lateral to the left, delta t = 10 min. Scale bar: 25 µm.
Formation of the exit and entry points in the BMs surrounding the OP and the brain visualised with a mosaic labelling of OP axons, related to Figure 1.
Confocal live-imaging performed on a TgBAC(lamC1:lamC1-sfGFP);Tg(cldnb:Gal4;UAS:lyn-TagRFP) embryo in which Laminin γ1-sfGFP is expressed under the control of its own promoter (green), and OP and peridermal skin cells are mosaically labelled in with membrane TagRFP (magenta). The yellow arrowhead shows the exit point on the last timepoint of the first movie, which appears as a small interruption (or several small interruptions) in the BM of the OP resulting from its incomplete assembly in this specific area. A lyn-TagRFP-positive individual axon can be seen exiting the OP through the exit point. The blue arrowhead indicates the opening of the entry point (which can also be formed by several gaps in some instances) in the already assembled BM of the brain, which is concomitant with the arrival of a few lyn-TagRFP-labelled axons. Only the left side of the embryo is shown. Average projection of two consecutive z-sections (z-step of 1 µm), frontal view, dorsal to the top and lateral to the left, delta t = 10 min. Scale bar: 25 µm.
3D z-stacks showing the exit and entry points, related to Figure 1.
Same embryo as the z-sections shown in Figure 1E-I and Figure 1—video 2. Confocal z-stacks performed on a live TgBAC(lamC1:lamC1-sfGFP);Tg(cldnb:Gal4;UAS:RFP) embryo in which Laminin γ1-sfGFP is expressed under the control of its own promoter (green), and OP and peridermal skin cells are labelled in with cytoplasmic RFP (magenta). The two z-stacks correspond to the last time points of the two time-lapses showing exit and entry point formation in single z-sections in Figure 1—video 2. The yellow arrowheads point to several interruptions in the BM of the OP (exit point). The blue arrowheads indicate the opening of several gaps in the BM of the brain (entry point). Only the left side of the embryo is shown. Frontal view, dorsal to the top, the first z-sections are the most anterior and the z-stacks progressively goes posterior, z-step of 1 µm. Scale bar: 25 µm.
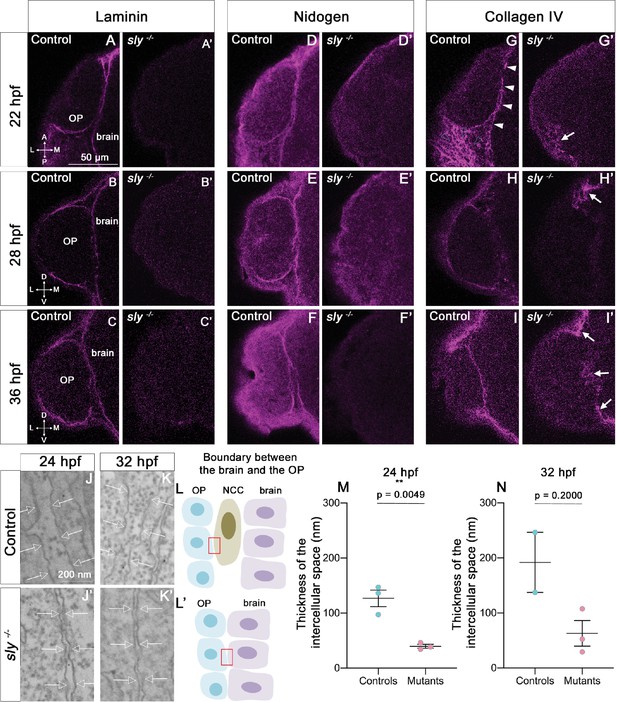
The integrity of the BMs of OP and brain tissues is strongly affected in sly mutants.
(A–I) Immunostaining for Laminin (A–C), Nidogen (D–F) and Collagen IV (G–I) (magenta) on sly mutants and control siblings at 22 (dorsal view), 28, and 36 hpf (frontal view). For Laminin and Nidogen, the linear, BM-like staining seen in controls around the OP and brain tissues is not detected in sly mutants. In G, white arrowheads = BM like linear Collagen IV staining, grey arrowheads = fibrous staining around the OP. In sly mutants, the linear Collagen IV pattern is dramatically disrupted, with, however, remaining fibrous patches of Collagen IV in some discrete areas located at the margin of the OP and/or between the OP and brain (arrows in G’, H’, I’) Scale bar: 50 µm. (J, K). Examples of EM images of the intercellular space between NCC and the OP in control siblings (J, K) and the OP and the brain in sly mutants (J’, K’), at 24 (J, J’) and 32 hpf (K, K’). Arrows = plasma membranes. The pictures were taken in the areas depicted with red boxes in L, L’. (L) Schematic view of the brain/OP boundary and of the areas (red boxes) where the pictures were taken in controls (L) and sly mutants (L’). OP, brain and NCC were identified by their position and shape: migrating NCC showed an elongated morphology along the AP axis, which differed from the round OP cell bodies and from brain neuroepithelial cells elongated along the ML axis. (M, N) Thickness of the intercellular space in sly mutants (between OP and brain cells) and control siblings (between NCC and brain or OP cells) at 24 hpf (n=3 controls; n=3 mutants) and 32 hpf (n=2 controls; n=3 mutants). For 24 hpf, unpaired, two-tailed t test. For 32 hpf, Mann-Whitney test.
-
Figure 2—source data 1
Thickness of the intercellular space in sly mutants (between OP and brain cells) and control siblings (between NCC and brain or OP cells).
- https://cdn.elifesciences.org/articles/92004/elife-92004-fig2-data1-v1.xlsx
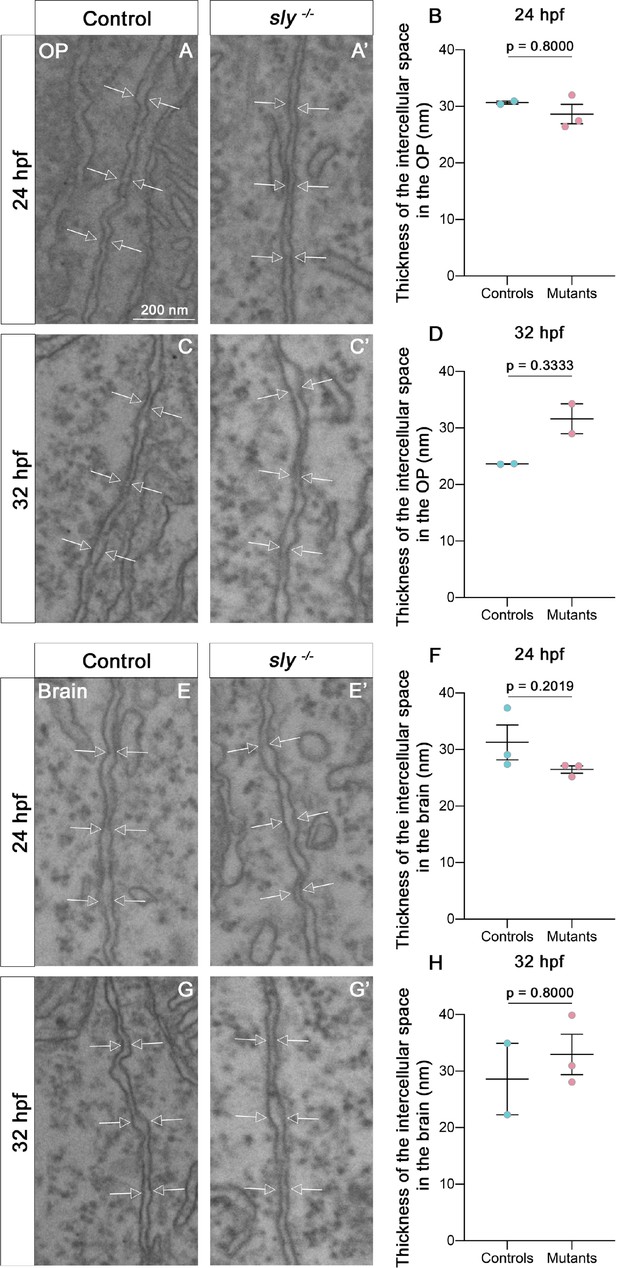
Electron microscopy analysis of intercellular spaces in the OP and brain tissues.
A, A’ and C, C’ Examples of images of the intercellular space located between OP cells in control siblings (A, C) and in sly mutants (A’, C’), at 24 and 32 hpf. Arrows = plasma membranes. (B, D) Thickness of the intercellular space in the OP tissue in sly mutants and control siblings at 24 (n=2 controls; n=3 mutants) and 32 hpf (n=2 controls; n=2 mutants). (E, E’ and G, G’) Examples of images of the intercellular space located between brain cells in control siblings (A, C) and in sly mutants (A’, C’), at 24 and 32 hpf. Arrows = plasma membranes. (F, H) Thickness of the intercellular space in the brain tissue in sly mutants and control siblings at 24 (n=3 controls; n=3 mutants) and 32 hpf (n=2 controls; n=3 mutants). Mann Whitney tests except for F: unpaired, two-tailed t-test.
-
Figure 2—figure supplement 1—source data 1
Thickness of the intercellular space within OP and brain tissues in sly mutants and control siblings.
- https://cdn.elifesciences.org/articles/92004/elife-92004-fig2-figsupp1-data1-v1.xlsx
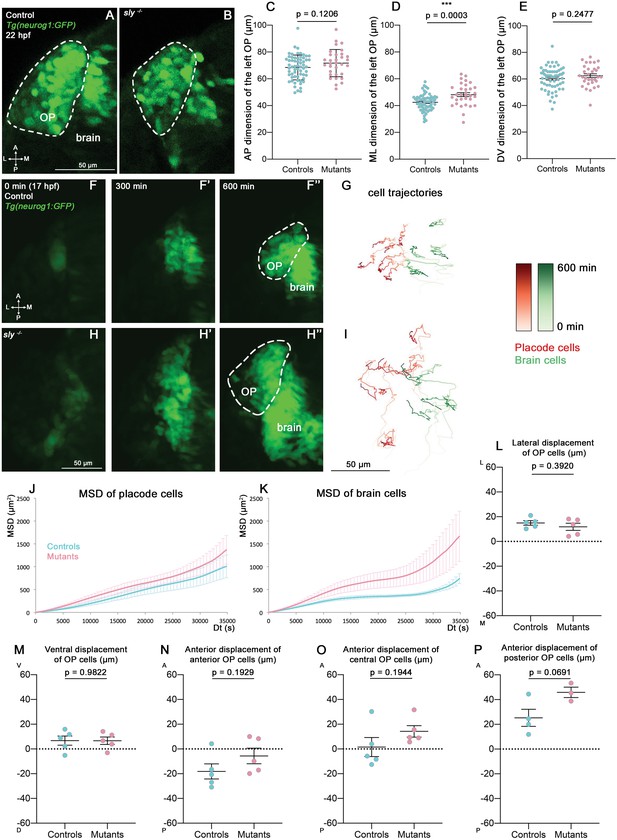
Analysis of OP coalescence in sly mutants and control siblings.
(A, B) Images (dorsal views, 1 z-section) of representative OPs from a Tg(neurog1:GFP); sly -/- mutant (right) and a control Tg(neurog1:GFP) sibling (left) at the end of OP coalescence (22 hpf). The Tg(neurog1:GFP)+ OP clusters are surrounded by dotted lines. (C–E) Graphs showing the anteroposterior (AP, in C), the mediolateral (ML, in D), and dorsoventral (DV, in E) dimensions of the Tg(neurog1:GFP)+ OP clusters in sly mutants (pink) and control siblings (blue) at 22 hpf (n=62 controls and n=32 mutants from two independent experiments). Ectopic fluorescent cells (cells that are physically separated from the main cluster) were not taken into account for the measurement of OP dimensions. Unpaired, two-tailed t test. (F-F’’ and H-H’) Images extracted from confocal live imaging on Tg(neurog1:GFP) control (F-F’’) and sly mutant (H-H’’) embryos during OP coalescence, dorsal view, average projection. Only the left side of the embryo is shown. (G, I) Examples of 2D tracks (ML along X and AP along Y) of Tg(neurog1:GFP)+ OP cells (red) and Tg(neurog1:GFP)+ brain cells (green) in a control (G) and a sly mutant embryo (I) Only the left side of the embryo is shown. The time is colour-coded: light colours at the beginning of the trajectory (17 hpf) towards dark colours for the end of the track (600 min later). (J, K) MSD analysis for OP cells (J) and brain cells (K) in sly mutants and control siblings (n=5 controls and n=5 mutants from three independent experiments, 10–14 cells analysed in each tissue). (L, M) Graphs showing the total lateral (L) and ventral (M) displacement of OP cells, starting at 17 hpf and during 600 min of time lapse (n=5 control placodes and n=5 mutant placodes from three independent experiments, 10–14 cells per placode, unpaired, two-tailed t test). (N–P) Graphs showing the total anterior displacement of anterior, central and posterior OP cells (as defined in Breau et al., 2017), starting at 17 hpf and during 600 min of time lapse (n=5 control placodes and n=5 mutant placodes from three independent experiments, mean calculated from 1 to 12 cells per placode, unpaired, two-tailed t test). Note that in some of the OPs we could not find any trackable (i.e. expressing H2B-RFP) posterior OP cell, which explains why there are only 4 control points and 3 mutant points in the graph showing the anterior displacement of posterior cells.
-
Figure 3—source data 1
OP dimensions at 22 hpf, and MSD analysis and cell displacements during OP coalescence in sly mutants and control siblings.
- https://cdn.elifesciences.org/articles/92004/elife-92004-fig3-data1-v1.xlsx
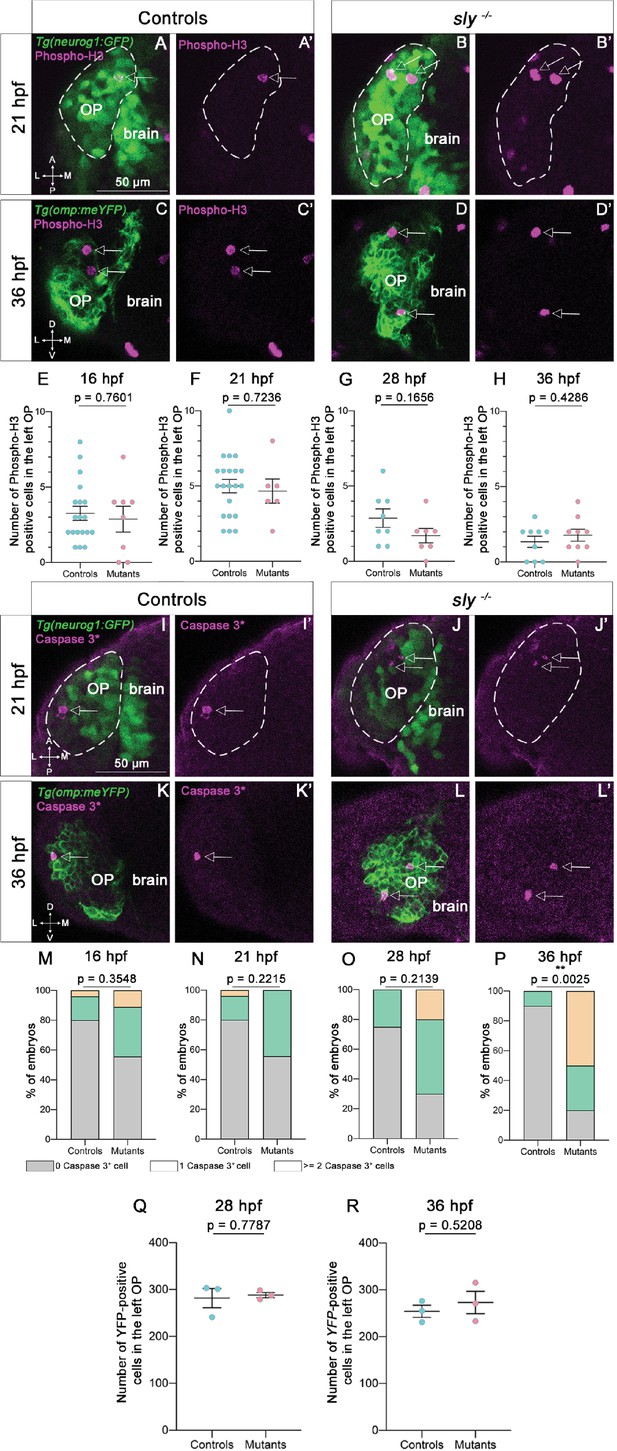
Analysis of proliferation and apoptosis in the OPs of sly mutants and control siblings.
(A-D’) Immunostaining for Phospho-histone H3 (magenta) to label dividing cells on Tg(neurog1:GFP) embryos (green) at 16 hpf and 21 hpf (A-B’ show images at 21 hpf, dorsal view), and on Tg(omp:meYFP) embryos (green) at 28 and 36 hpf (C-D’ show images at 36 hpf, frontal view). In A-B’ the OP is surrounded by a dotted line. Arrows = instances of Phospho-histone H3 positive cells in the placodal Tg(neurog1:GFP)+ or Tg(omp:meYFP)+ clusters. (E–H) Number of Phospho-histone H3 positive cells in the OP at 16 hpf (n=19 controls; n=8 mutants), 21 hpf (n=21 controls; n=6 mutants), 28 hpf (n=8 controls; n=7 mutants) and 36 hpf (n=9 controls; n=9 mutants) in control embryos and sly mutants. Unpaired, two-tailed t test. (I-L’) Immunostaining for Activated Caspase 3 (magenta) to label apoptotic cells/debris on Tg(neurog1:GFP) embryos (green) at 16 hpf and 21 hpf (I-J’ show images at 21 hpf, dorsal view), and on Tg(omp:meYFP) embryos (green) at 28 and 36 hpf (K-L’ show images at 36 hpf, frontal view). In I-J’ the OP is surrounded by a dotted line. Arrows = instances of Activated Caspase 3 positive cells or debris in the OP or in contact with the placodal Tg(neurog1:GFP)+ or Tg(omp:meYFP)+ clusters. (M–P) % of left OPs with zero, one or two and more Activated Caspase 3 positive cells or debris in the OP or very close to the OP at 16 hpf (n=25 controls; n=9 mutants), 21 hpf (n=25 controls; n=9 mutants), 28 hpf (n=8 controls; n=10 mutants) and 36 hpf (n=10 controls; n=10 mutants) in control embryos and sly mutants. Chi2 test. (Q, R) Total number of YFP+ cells in control and mutant Tg(omp:meYFP)+ OPs at 28 hpf (Q) and 36 hpf (R) (n=3 control placodes; n=3 mutant placodes for each stage). Unpaired, two-tailed t test.
-
Figure 3—figure supplement 1—source data 1
Analysis of OP proliferation and apoptosis and total number of YFP+ cells in sly mutants and control siblings.
- https://cdn.elifesciences.org/articles/92004/elife-92004-fig3-figsupp1-data1-v1.xlsx
OP coalescence in a control embryo and a sly mutant, related to Figure 3.
Same embryos as the images and analyses shown in Figure 3F-I. Confocal live imaging showing OP coalescence movements in sly mutant and control Tg(neurog1:GFP) embryos, in which OP and brain neurons are labelled with cytoplasmic GFP. On the last time point, the dotted lines outline the OPs. Note that on the sly mutant movie, a marked drift movement occurs at t = 170 min, which has been taken into account and substracted from the data in our cell tracking analysis. These embryos were injected with H2B-RFP mRNA to label nuclei and allow individual cell tracking (not shown). Average projection, dorsal view, anterior to the top, delta t = 10 min. Scale bar: 50 µm.
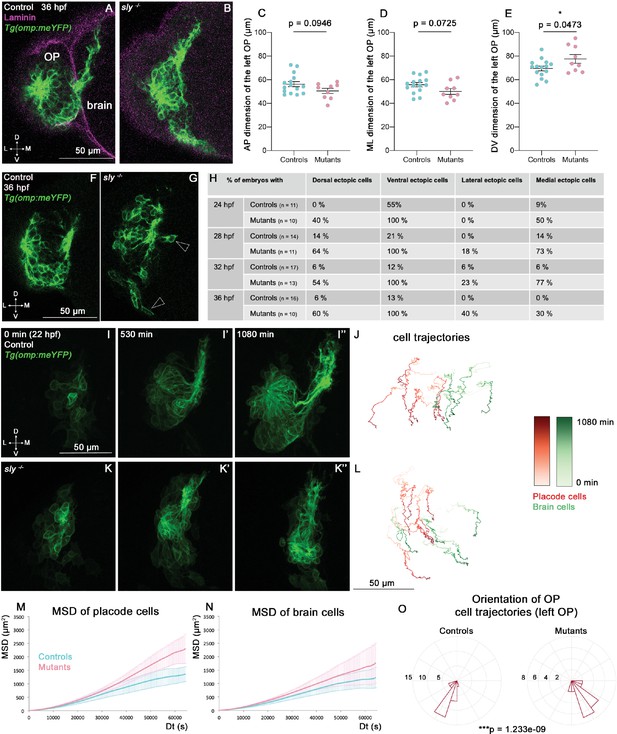
Analysis of OP and brain morphogenesis in sly mutants and control siblings during the forebrain flexure.
(A, B) Images (frontal view, 1 z-section) of representative placodes from a Tg(omp:meYFP); sly -/- mutant (right) and a control Tg(omp:meYFP) sibling (left) at 36 hpf. Laminin immunostaining in magenta. (C–E) Graphs showing the anteroposterior (AP, in C), the mediolateral (ML, in D), and dorsoventral (DV, in E) dimensions of the Tg(omp:meYFP)+ OP clusters in sly mutants (pink) and control siblings (blue) at 36 hpf (n=15 controls and n=9 mutants from four independent experiments). Ectopic fluorescent cells (cells that are physically separated from the main cluster) were not taken into account for the measurement of OP dimensions. Unpaired, two-tailed t test. Similar measurements performed at younger stages are shown in Figure 4—figure supplement 1A–L. (F, G) Examples of images used for the analysis of ectopic cells, defined as Tg(omp:meYFP)+ cells being physically separated from the main YFP+ cluster. Arrowheads show instances of ectopic cells in a sly mutant. (H) Table showing the % of control and mutant embryos with at least one ectopic cell located dorsally, ventrally, laterally, and medially to the main YFP+ cluster. The numbers of analysed embryos are indicated in the table. (I-I’’ and K-K’’) Images extracted from confocal live imaging on Tg(omp:meYFP) control (I-I’’) and sly mutant (K-K’’) embryos during the forebrain flexure, from 22 hpf and over 1080 min, frontal view, maximum projection. Only the left side of the embryo is shown. (J, L) Examples of 2D tracks (ML along X and DV along Y) of Tg(omp:meYFP)+ OP cells (red) and adjacent brain cells (green) in a control (J) and a sly mutant (L). The time is colour-coded: light colours at the beginning of the trajectory (22 hpf) towards dark colours for the end of the track (1080 min later). Only the left side of the embryo is shown. (M, N) 3D MSD analysis of OP (M) and brain cells (N) in sly mutants and control siblings (n=4 controls and n=3 mutants from five independent experiments, 10–14 cells analysed in each tissue). (O) Rose plots indicating the orientation of the movement for control and mutant left OP cells (data pooled from n=4 controls and n=3 mutants from five independent experiments). Numbers = number of cells. Dorsal to the top, lateral to the left. There is a statistical difference in cell track orientations between controls and mutants (circular analysis of variance based on the likelihood ratio test: p=1.233e-09 for the left OPs, and p=3.439e-08 for the right OPs, the graphs for the rigth OPs are not shown).
-
Figure 4—source data 1
OP dimensions at 36 hpf, and MSD analysis and angles of OP cell trajectories from 22 hpf in sly mutants and control siblings.
- https://cdn.elifesciences.org/articles/92004/elife-92004-fig4-data1-v1.xlsx
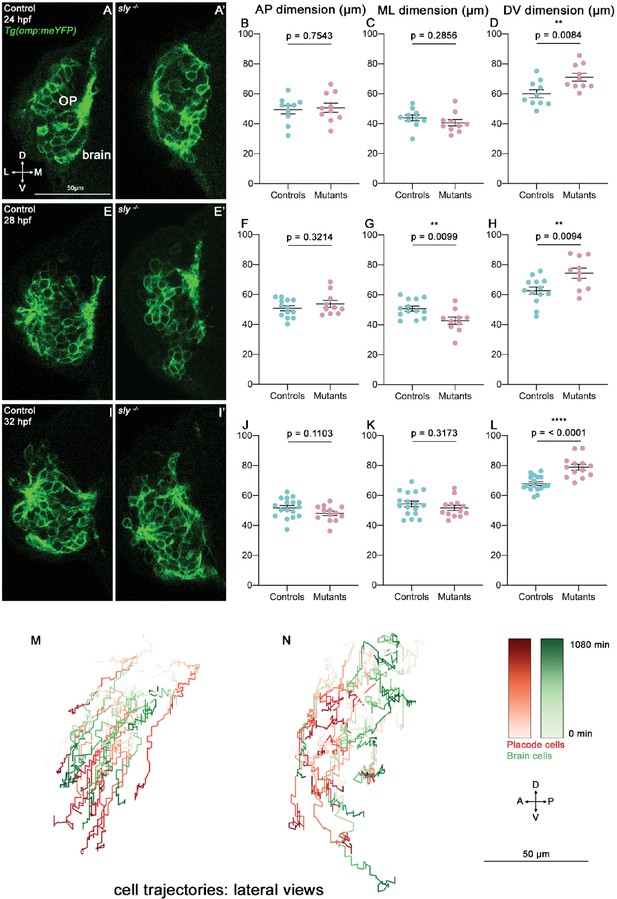
Additional results on the OP morphogenesis defects observed in sly mutants.
(A-L) Analysis of OP dimensions at 24, 28, and 32 hpf. (A, A’, E, E’ and I, I’) Images (frontal views, 1 z-section) of representative OPs from Tg(omp:meYFP); sly -/- mutants (right) and control Tg(omp:meYFP) siblings (left) at 24 (A, A’), 28 (E, E’) and 32 hpf (I, I’). B–D Graphs showing the AP, ML, and DV dimensions of the Tg(omp:meYFP)+ OP clusters in sly mutants and control siblings at 24 hpf (n=10 controls and n=10 mutants from three independent experiments). Ectopic fluorescent cells (cells that are physically separated from the main cluster) were not taken into account for the measurement of OP dimensions. Unpaired, two-tailed t test. (F–H). Similar quantifications at 28 hpf (n=13 controls and n=10 mutants from two independent experiments). Unpaired, two-tailed t tests. (J–L). Similar quantifications at 32 hpf (n=17 controls and n=13 mutants from two independent experiments). Unpaired, two-tailed t tests. Quantifications performed at 36 hpf are shown in Figure 4C–E. (M, N) Examples of 2D tracks plotted in a lateral view (AP along X and DV along Y) of Tg(omp:meYFP)+ OP cells (red) and adjacent brain cells (green) in a control (M) and a sly mutant embryo (N). The time is colour-coded: light colours at the beginning of the trajectory (22 hpf) towards dark colours for the end of the track (1080 min later).
-
Figure 4—figure supplement 1—source data 1
OP dimensions at 24, 28, and 32 hpf in sly mutants and control siblings.
- https://cdn.elifesciences.org/articles/92004/elife-92004-fig4-figsupp1-data1-v1.xlsx
Behaviour of the OP neurons during the brain flexure movement in a control embryo and a sly mutant, related to Figure 4.
Same embryos as the images and analyses shown in Figure 4I-L. Confocal live imaging showing OP cell movements and axon behaviours in sly mutant and control Tg(omp:meYFP) embryos, in which ompb-expressing OP neurons and their axons are labelled with membrane YFP. Note the global ventral movement occuring during the time-lapse, which corresponds to the movements of the OPs accompanying the brain flexure. These embryos were injected with H2B-RFP mRNA to label nuclei and allow individual cell tracking (not shown). Maximum projection, frontal view, dorsal to the top, delta t = 10 min. Scale bar: 50 µm.
3D stacks showing the axonal defects and ectopic OP cells observed in sly mutants, related to Figure 4, Figure 6—figure supplement 1.
The embryos are at 28 hpf and carry the Tg(omp:meYFP) transgene to visualise the membrane of ompb-expressing OP neurons and their axons (green). In control embryos, the bundle of axons exit the OP in a ventral position and migrate dorsally towards the presumptive OB. In mutant embryos, only a few axons manage to exit the OP in a dorsal position and reach the presumptive OB (white arrow). Most of them appear to be stalled at the OP/brain boundary (grey arrow). The mutant embryo also exhibits ectopic OP cells that are detached from the main OP cluster (magenta dots). White dots indicate the centre of the rosette of OP neurons that assemble in the dorso-lateral region of the OP. Only the left side of the embryo is shown. Frontal views, dorsal to the top, lateral to the left, the first z-sections are the most anterior and the z-stack progressively goes posterior, z-step of 1 µm. Scale bar: 25 µm.
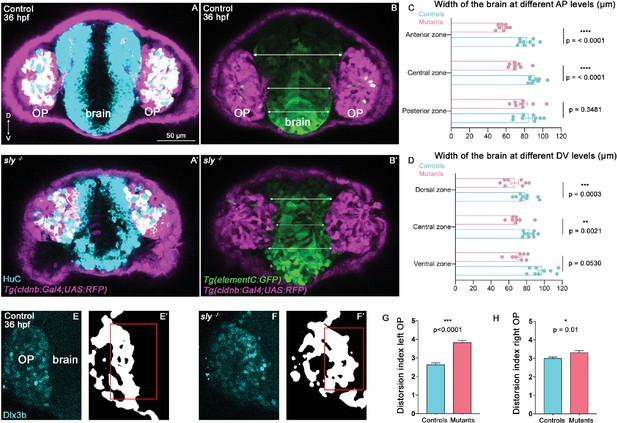
Analysis of brain width and brain/placode boundary in sly mutants and control siblings.
(A, A’) Immunostaining for HuC (cyan) at 36 hpf on Tg(cldnb:Gal4; UAS:RFP) (magenta) control and sly mutant embryos (frontal view). Similar immunostainings performed at 28 hpf are shown in Figure 5—figure supplement 1A, A’. (B, B’). Images of Tg(elementC:gfp); Tg(cldnb:Gal4; UAS:RFP) control and mutant embryos at 36 hpf (frontal view), similar images acquired at 28 hpf are shown in Figure 5—figure supplement 1B, B’. GFP (green) is expressed by the forebrain and a few OP cells. Arrows indicate where the brain width was measured (in three distinct positions along the DV axis). Measurements were also carried out at three distinct AP levels (through the z-stack). (C, D) Width of the forebrain in 36 hpf controls and sly mutants, at three different DV and three different AP levels (n=9 controls and n=8 mutants from four independent experiments, unpaired, two-tailed t test). Quantifications for the 28 hpf stage are shown in Figure 5—figure supplement 1C, D. (E-F’) Immunostaining for the OP marker Dlx3b (cyan) was performed on 36 hpf sly mutants and control siblings (frontal view). The signal was segmented using deep learning approaches (white signal), and the distortion index (see Materials and methods) of the OP/brain boundary was calculated in the regions outlined with red boxes. (G, H) Graphs showing the distortion indexes in controls and mutants at 36 hpf, for the left and right OPs (n=3 controls and n=3 mutants). ANOVA test (mixed models, with animals as random effect and genotype and side as fixed effects).
-
Figure 5—source data 1
Brain width measurements and distortion index of the OP/brain boundary in sly mutants and control siblings.
- https://cdn.elifesciences.org/articles/92004/elife-92004-fig5-data1-v1.xlsx
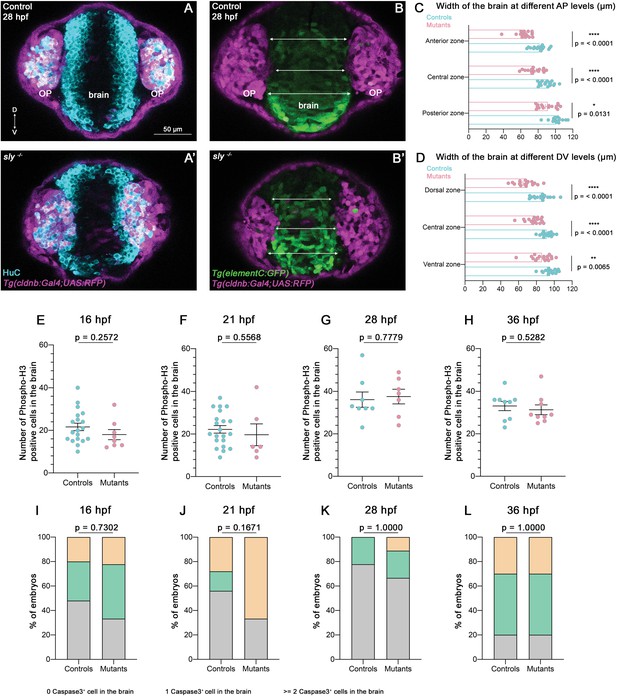
Additional results for the analysis of brain shape and proliferation/apoptosis in sly mutants and control siblings.
(A, A’) Immunostaining for HuC (cyan) at 28 hpf performed on Tg(cldnb:Gal4; UAS:RFP) (magenta) control and sly mutant embryos (frontal view). Similar immunostainings performed at 36 hpf are shown in Figure 5A and A’. (B, B’). Images of Tg(elementC:gfp); Tg(cldnb:Gal4; UAS:RFP) control and mutant embryos at 28 hpf (frontal view), similar images acquired at 36 hpf are shown in Figure 5B and B’. GFP (green) is expressed by the forebrain, and a few OP cells. Arrows indicate where the thickness of the forebrain was measured (in three distinct positions along the DV axis). Measurements were also carried out at three distinct AP levels (through the z-stack). (C, D) Width of the forebrain in 28 hpf controls and sly mutants, at three different DV and three different AP levels (n=17 controls and n=17 mutants from five independent experiments, unpaired, two-tailed t tests except for anterior and posterior zones, for which a Mann-Whitney test was applied). Quantifications for the 28 hpf stage are shown in Figure 5C and D. (E–H) Number of Phospho-histone H3 positive cells in the brain at 16, 21, 28, and 36 hpf in control embryos and sly mutants. Unpaired, two-tailed t test. (I–L) % of embryos with zero, one, or two and more Activated Caspase 3 positive cells or debris in the brain at 16, 21, 28, and 36 hpf in control embryos and sly mutants. Chi2 test.
-
Figure 5—figure supplement 1—source data 1
Brain width measurements and analysis of brain proliferation and apoptosis in sly mutants and control siblings.
- https://cdn.elifesciences.org/articles/92004/elife-92004-fig5-figsupp1-data1-v1.xlsx
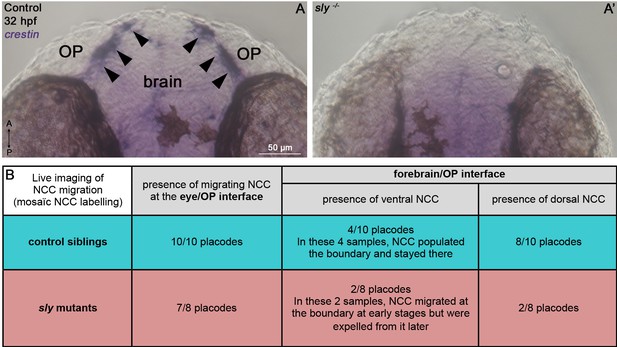
NCC defects in sly mutants.
(A, A’) In situ hybridisation for the NCC marker crestin on 32 hpf controls and sly mutants. Arrowheads = crestin-positive NCC cluster at the OP/brain interface, which is absent in sly mutants. (B) Table reporting our observations from live imaging of cranial NCC migration in sly mutants and control siblings (see Figure 5—video 2). Confocal live imaging was performed from 16 to 32 hpf on sly mutant and control Tg(neurog1:GFP);Tg(UAS:RFP) embryos injected with the sox10(7.2):KalTA4 plasmid, allowing a mosaïc labelling of NCC.
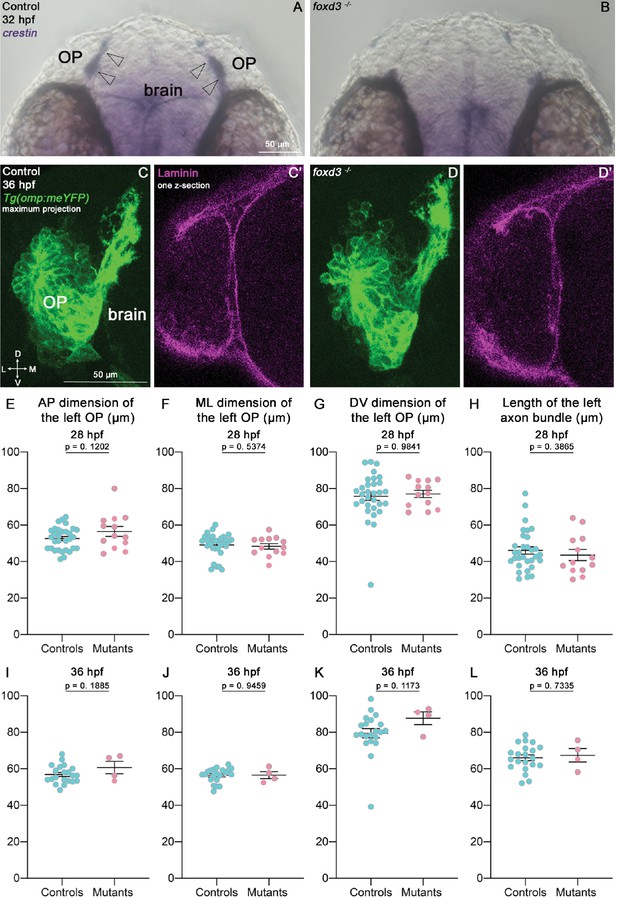
Olfactory system development in foxd3 mutants.
(A, B) In situ hybridisation for the NCC marker crestin on 32 hpf controls and foxd3 mutants. Arrowheads = crestin-positive NCC cluster at the OP/brain interface, which is absent in foxd3 mutants. (C, D). Images (frontal view, maximum projection) of representative OPs of Tg(omp:meYFP); foxd3 -/- mutants and control siblings. (C’, D’) Laminin immunostaining (1 z-section) on the same embryos. (E–H) OP dimensions and length of the axon bundle at 28 hpf in foxd3 mutants and control siblings (n=31 controls and n=13 mutants from two independent experiments). (I–L) OP dimensions and length of the axon bundle in foxd3 mutants and control siblings at 36 hpf (n=21 controls and n=4 mutants from two independent experiments). Unpaired, two-tailed t tests for E, I, J, L and Mann-Whitney tests for F, G, H, K.
-
Figure 5—figure supplement 3—source data 1
OP dimensions and length of the axon bundle in foxd3 mutants and controls siblings.
- https://cdn.elifesciences.org/articles/92004/elife-92004-fig5-figsupp3-data1-v1.xlsx
3D stacks showing the organisation of brain and OP neurons in control embryos and sly mutants, related to Figure 5, Figure 5—figure supplement 1.
sly mutant and control Tg(cldnb:Gal4;UAS:RFP) embryos, in which OP and peridermal skin cells are labelled in with cytoplasmic RFP (magenta), were immunostained for HuC (cyan) at 28 hpf and 36 hpf to visualise the neurons in the OP and the brain. Note that in control embryos, the frontier between the OP and the brain appears as a straight line whereas in mutant embryos, the boundary between the two tissues is curved and not well defined, and the OPs appear to be partially embedded into the brain tissue. Frontal views, dorsal to the top, the first z-sections are the most anterior and the z-stack progressively goes posterior, z-step of 1 µm. Scale bar: 50 µm.
NCC migration in sly mutants and control siblings, related to Figure 5—figure supplement 2.
Confocal live imaging from 16 to 32 hpf showing OP and NCC cell movements in sly mutant and control Tg(neurog1:GFP);Tg(UAS:RFP) embryos injected with the sox10(7.2):KalTA4 plasmid. The DNA injection in this transgenic background allows the mosaic RFP labelling of NCC and their derivatives. Note that in the mutant, a few neuroepithelial brain cells also express RFP. The blue arrowheads point to instances of NCC migrating between the eye and OP tissues, the yellow arrowheads indicate some NCC populating the OP/forebrain interface. In the sly mutant embryo, the NCC migrating at the OP/forebrain boundary appear to be expelled from the interface, and one of them eventually dies. Only the left side of the embryo is shown. Average projection, dorsal view, lateral to the left, delta t = 10 min. Scale bar: 20 µm.
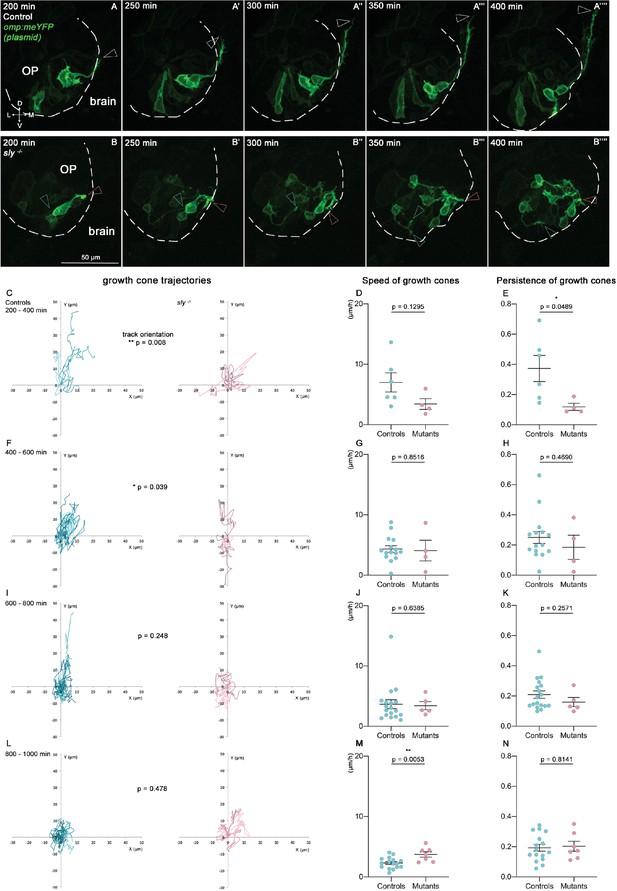
Quantitative live imaging of axonal behaviours in sly mutants and control siblings.
(A, B) Images extracted from confocal live imaging on control (A-A’’’’) and sly mutant (B-B’’’’) embryos injected with the omp:meYFP plasmid to obtain a mosaic labelling of OP neurons and their axons (frontal view, maximum projections). The OP neurons and their axons were imaged every 10 min over 1000 min from 22 hpf. Here, only the 200–400 min time window is shown as an example. Arrowheads = positions of individual growth cones over time. (C–N). Individual YFP+ growth cones, as well as YFP+ cell bodies in the OP, were tracked during 4 consecutive periods of 200 min each (from 200 min of imaging, since before no growth cone could be detected, n=5 mutants and n=5 controls from six independent experiments). The mean movement of OP cell bodies was substracted from the growth cone tracks to get rid of the global flexure movement. 200–400 min: 6 growth cones in controls, 4 in mutants; 400–600 min: 15 growth cones in controls, 4 in mutants; 600–800 min: 18 growth cones in controls, 4 in mutants; 800–1000 min: 16 growth cones in controls, 7 in mutants. (C, F, I, L) Tracks of the growth cones merged at their origin for the 4 consecutive periods of 200 min. For each time window, the difference in the orientation of the tracks was analysed using the circular analysis of variance based on the likelihood ratio test. (D, G, J, M) Mean speed of the growth cones. Unpaired, two-tailed t tests. (E, H, K, N) Persistence of the growth cones, defined as the distance between the initial and final positions of the growth cones divided by the total length of their trajectory. Unpaired, two-tailed t tests, except for the analysis of the persistence at 400–600 min, and for the speed and persistence at 600–800 min, where Mann-Whitney tests were performed.
-
Figure 6—source data 1
Mean speed and persistence of growth cones in sly mutants and control siblings.
- https://cdn.elifesciences.org/articles/92004/elife-92004-fig6-data1-v1.xlsx
-
Figure 6—source data 2
Angles of growth cone trajectories in sly mutants and control siblings.
- https://cdn.elifesciences.org/articles/92004/elife-92004-fig6-data2-v1.xlsx
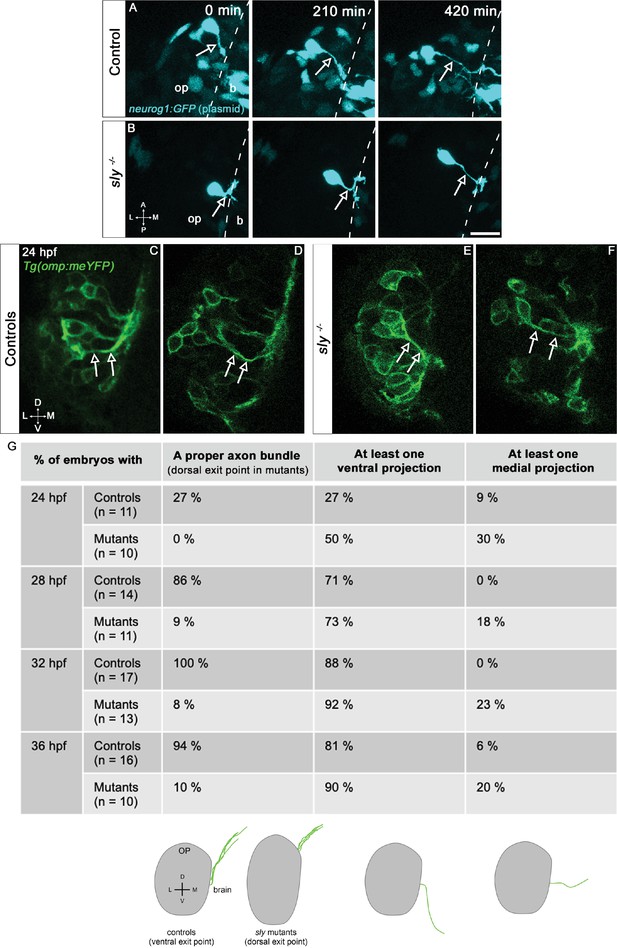
Additional results on olfactory axon development in sly mutants and control siblings.
(A, B) Images extracted from confocal live imaging experiments performed during OP coalescence on Tg(cldnb:Gal4; UAS:RFP) embryos injected with the neurog1:GFP plasmid, allowing a sparse labelling of neurog1:GFP +neurons/axons. The pictures illustrate instances of retrograde axon extension/cell body mediolateral movement occuring in a sly mutant and a control sibling. Arrows = proximal portions of the axons extending in the OP. Dotted line = brain/OP boundary (identified with the RFP signal which is not shown here). op = olfactory placode, b=brain. Scale bar: 20 µm. (C–F) Images (frontal view, 1 z-section) of representative OPs from Tg(omp:meYFP); sly -/- mutant (E, F) and control Tg(omp:meYFP) siblings (C, D) at 24 hpf. Arrows = instances of proximal portions of the axons in the OP. (G) Table describing the % of control and sly mutant Tg(omp:meYFP) embryos with a proper axonal bundle (left column), with at least one ventral projection (middle column) and with at least one medial projection (right column) at 24, 28, 32 and 36 hpf. The numbers of embryos analysed at each stage are indicated in the table. The schematic view under the table illustrates the phenotypes assessed in each column. Note that in the mutants, as shown on the schematics, when a proper axon bundle is observed (left column), the exit point of the axons is more dorsal than in controls (see Figure 4A, B, Figure 4—figure supplement 1E’ for instance).
Tables
Reagent type (species) or resource | Designation | Source or reference | Identifiers | Additional information |
---|---|---|---|---|
Strain (Danio rerio) | Zebrafish wild-type hybrid (TL x AB) strains | IBPS aquatic facility, Paris | N/A | |
Strain (Danio rerio) | slywi390 (sly/lamc1) | Wiellette et al., 2004; PMID:15593329 | ZDB-ALT-050317–6 | |
Strain (Danio rerio) | foxd3zdf10 | Stewart et al., 2006; PMID:16499899 | ZDB-ALT-060519–4 | |
Strain (Danio rerio) | Tg(–8.4neurog1:GFP)sb1 | Blader et al., 2003; PMID:12559493 | ZDB-ALT-030904–6 | |
Strain (Danio rerio) | Tg(–2.0ompb:gapYFP)rw032 | Sato et al., 2007; PMID:17301169 | ZDB-ALT-050513–2 | |
Strain (Danio rerio) | Tg(–4.0cldnb:GalTA4, cry:RFP)nim11 | Breau et al., 2013; PMID:24082091 | ZDB-ALT-130822–6 | |
Strain (Danio rerio) | Tg(14XUAS:mRFP,Xla.Cryg:GFP)tpl2 | Balciuniene et al., 2013; PMID:24034702 | ZDB-ALT-131119–25 | |
Strain (Danio rerio) | TgBAC(lamc1:lamc1-sfGFP,cryaa:Cerulean)sk116Tg | Yamaguchi et al., 2022; PMID:35165417 | ZDB-ALT-241010–5 | |
Strain (Danio rerio) | Tg(eltC:GFP)zf199Tg | Stedman et al., 2009; PMID:19152797 | ZDB-ALT-101103–3 | |
Strain (Danio rerio) | Tg(UAS:lyn-tagRFP) | This study | Lab of Filippo Del Bene, Institut de la Vision, Paris | |
Antibody | Rabbit anti-Laminin polyclonal | Sigma-Aldrich | Cat# L9393; RRID:AB_477163 | 1/200 |
Antibody | Rabbit anti-Nidogen polyclonal | Abcam | Cat# ab14511, RRID:AB_301290 | 1/200 |
Antibody | Rabbit anti-Collagen IV polyclonal | Abcam | Cat# ab6586, RRID:AB_305584 | 1/200 |
Antibody | Chicken anti-GFP polyclonal | Aves labs | Cat# GFP-1020, RRID:AB_10000240 | 1/200 |
Antibody | Rabbit anti-DsRed polyclonal | Takara Bio | Cat# 632496; RRID:AB_10013483 | 1/300 |
Antibody | Rabbit anti phospho-Histone H3 polyclonal | Millipore | Cat# 06–570; RRID:AB_310177 | 1/200 |
Antibody | Rabbit anti Caspase 3, active form polyclonal | R and D Systems | Cat# AF835; RRID:AB_2243952 | 1/200 |
Antibody | Mouse anti-HuC/HuD clone 16A11 | ThermoFisher scientific | Cat# A-21271, RRID:AB_221448 | 1/200 |
Antibody | Mouse anti-Dlx3b monoclonal | ZIRC | Cat# anti-DLX3b, RRID:AB_10013771 | 1/500 |
recombinant DNA reagent | 10XUAS:lyn-tagRFP plasmid | This study | Gateway cloning, Lab of Filippo Del Bene, Institut de la Vision, Paris | |
recombinant DNA reagent | –2.0ompb:gapYFP plasmid | Miyasaka et al., 2005; PMID:15716341 | ZDB-TGCONSTRCT-070117–121 | |
recombinant DNA reagent | sox10(7.2):KalTA4 plasmid | Almeida and Lyons, 2015; PMID:26485616 | ZDB-TGCONSTRCT-170418–9 | |
sequence-based reagent | FW genotyping primer for slywi390 | This study | CATGACGGCAAAGTTGGTGA | |
sequence-based reagent | RV1 genotyping primer for slywi390 | This study | CCATGCCTTGCAAAATGGCGTTA CTTAA | |
sequence-based reagent | RV2 genotyping primer for slywi390 | This study | TGTAGGAGAGAAGTCGCGAG | |
sequence-based reagent | crestin PCR amplification for ISH probe synthesis FW primer | This study | AAGCCCTCGAAACTCACCTG | |
sequence-based reagent | crestin PCR amplification for ISH probe synthesis RV primer | This study | CCACTTGATTCCCACGAGCT | |
Commercial assay or kit | Multisite Gateway system kit | Invitrogen | Cat# 12537–023 | |
software, algorithm | LASX | Leica | RRID:SCR_013673 | |
software, algorithm | Fiji | https://imagej.net/Fiji/Downloads | RRID:SCR_002285 | |
software, algorithm | Ilastik | https://www.ilastik.org/ | RRID:SCR_015246 | |
software, algorithm | Prism | GraphPad https://www.graphpad.com/ | RRID:SCR_002798 | |
software, algorithm | Numpy library, Python | Harris et al., 2020 | RRID:SCR_008633 | |
software, algorithm | Matlab | The Mathworks, Inc. https://fr.mathworks.com/products/matlab.html | RRID:SCR_001622 |