Neural attentional filters and behavioural outcome follow independent individual trajectories over the adult lifespan
Figures
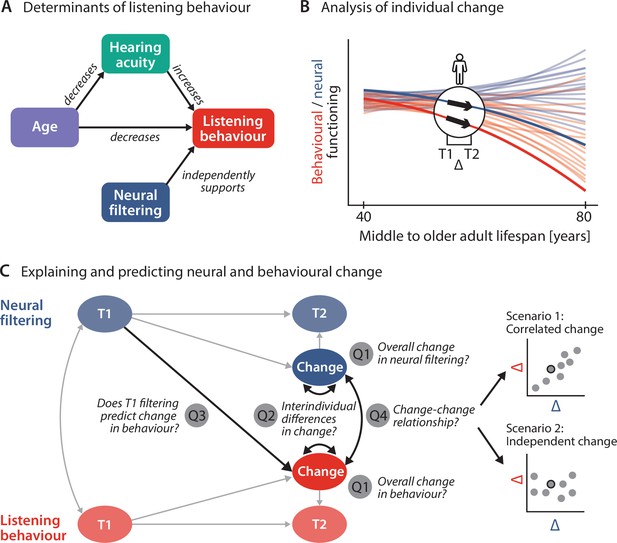
Schematic illustration of key assumptions and research questions.
(A) Listening behaviour at a given timepoint is shaped by an individual’s sensory and neural functioning. Increased age decreases listening behaviour both directly and indirectly via age-related hearing loss. Listening behaviour is supported by better neural filtering ability, independently of age and hearing acuity. (B) Conceptual depiction of individual 2-year changes along the neural (blue) and behavioural (red) domain. Thin coloured lines show individual trajectories across the adult lifespan, while thick lines and black arrows highlight 2-year changes in a single individual. (C) Left schematic diagram highlighting the key research questions detailed in the introduction and how they are addressed in the current study using latent change score modelling. Right: across individuals, co-occurring changes in the neural and behavioural domain may be correlated (top) or independent of one another (bottom).
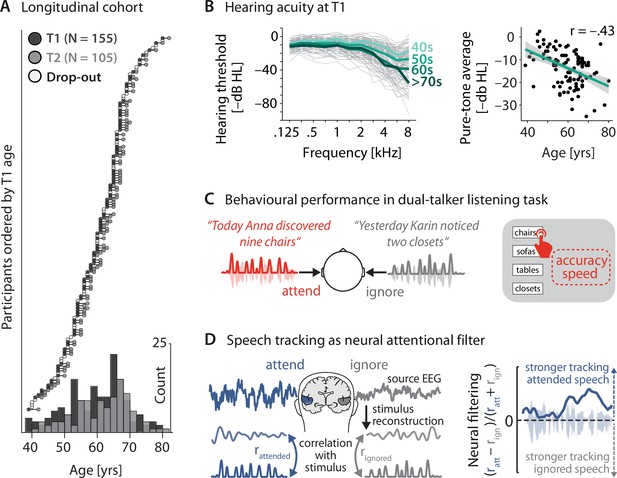
Key neural and behavioural metrics derived from a longitudinal cohort.
(A) Longitudinal cohort of healthy middle-aged and older adults measured twice, 2 y apart. Circles represent individual participants at a given measurement time (dark grey: timepoint [T] 1, light grey: T2, white: dropouts after T1). Bottom: age distribution at T1 and T2 across 5-year bins. (B) Left: T1(N = 155) air conduction hearing thresholds per individual (thin grey lines) and age group (thick coloured lines). Note that for didactic purposes, throughout the article, thresholds are expressed as –dB HL to highlight the decrease in hearing acuity with age (left). Right: Pure-tone average hearing acuity (0.5, 1, 2, and 4 kHz across both ears; higher is better) negatively correlates with age (N = 155; r = –0.43, p=3.73 × 10–6). (C) Participants listened to two sentences presented simultaneously to the left and right ear. In 50% of trials, a preceding visual cue indicated the to-be-attended target sentence. Listening behaviour is quantified via the accuracy and speed in identifying the final word of the target sentence. (D) Left: neural speech tracking as a proxy of an individual’s neural filtering ability. Stimulus envelopes of attended and ignored sentences were reconstructed from source-localised electroencephalogram (EEG) activity in auditory cortex (see ‘Materials and methods’ for details) and correlated with the actual envelopes. Right: better neural filtering results from stronger neural tracking of attended compared to ignored speech. We analysed neural filtering derived from the entire sentence presentation period.
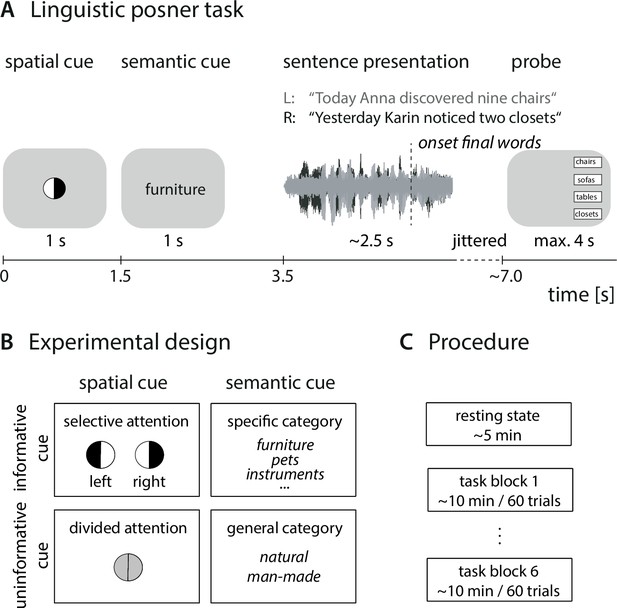
Experimental design and procedure.
(A) Trial structure of dichotic listening task. In each trial, participants listened to two dichotically presented short sentences spoken by the same speaker. They had to identify the final word in one of the two sentences. Sentence presentation was preceded by two visual cues. (B) 2 × 2 design in which the presentation of the spatial-attention and semantic cue was fully crossed. Each cue was presented in either an informative or uninformative neural version. (C) Prior to the listening task, we recorded resting-state electroencephalogram (EEG) data. The listening task was presented in six blocks of 60 trials with break in between.
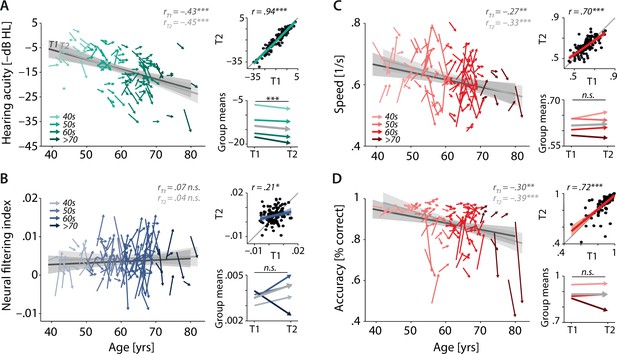
Characterising cross-sectional and longitudinal change along the auditory sensory (A), neural (B), and behavioural (C, D) domain.
For each domain, coloured vectors (colour coding four age groups for illustrative purposes, only) in the respective left subpanels show an individual’s change from T1 to T2 (N = 105), along with the cross-sectional trend plus 95% confidence interval (CI) separately for T1 (dark grey) and T2 (light grey). Top right subpanels: correlation of T1 and T2 as a measure of test–retest reliability along with the 45° line (grey) and individual data points (black circles). Bottom right panels: mean longitudinal change per age group (coloured vectors) and grand mean change (grey). Note that accuracy is expressed here as proportion correct for illustrative purposes, but was analysed logit-transformed or by applying generalised linear models.
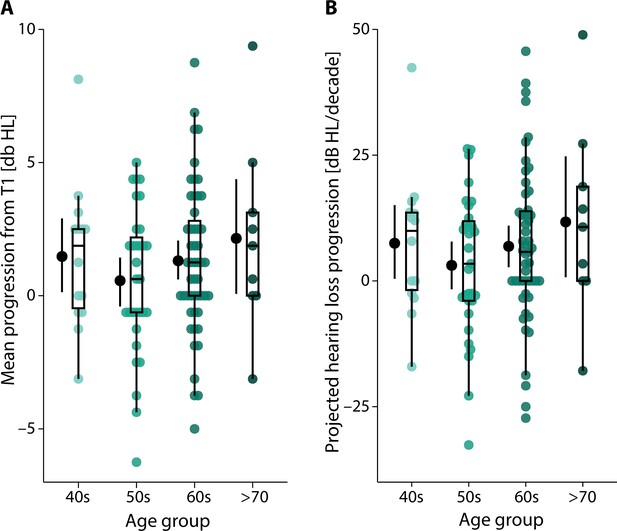
Observed and projected hearing loss progression.
(A) Mean progression of hearing loss from T1 to T2 as calculated from 2-year differences in the individual pure-tone average hearing thresholds at 0.5, 1,2, and 4 kHz. Coloured dots represent the single-subject difference across four age groups (total N = 105 participants), while black dots and error bars indicate grand-average and bootstrapped 95% confidence interval (CI). Box plots show median centre line, 25th to 75th percentile hinges, whiskers indicate minimum and maximum within 1.5× interquartile range. (B) Analogue to panel (A) but showing the projected hearing loss progression (increase in db HL per decade) assuming a constant rate of change per individual.
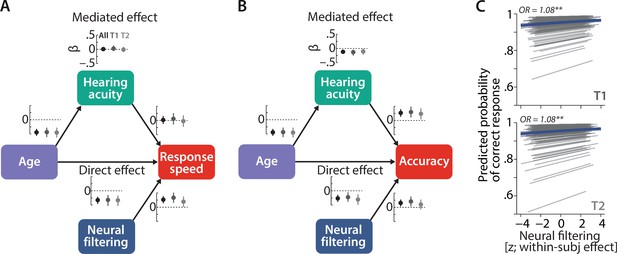
Longitudinal stability of sensory and neural determinants of listening behaviour.
Causal mediation analysis of age, hearing acuity, and neural filtering on response speed (A) and logit-transformed accuracy (B) of N = 105 individuals. Graphs next to each path indicate standardised coefficients plus 95% confidence interval (CIs) separately for the full dataset (black), T1 (dark grey), and T2 (light grey). We did not find any significant modulation of the observed effects with time (see text for results). (C) Neural filtering strength was found to be predictive of accuracy at the single-trial level at both T1 (top) and T2 (bottom). Grey lines show individual effects, while blue thick line shows the group-level fixed effect along with 95% CI. OR = odds ratio, **p<0.01.
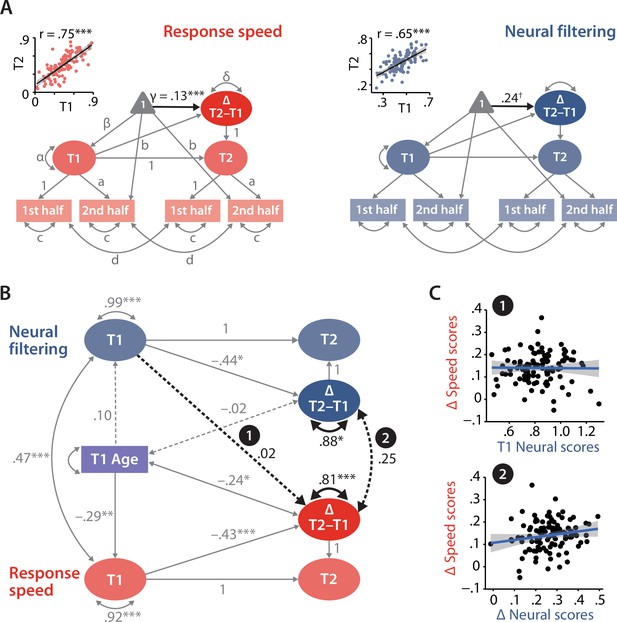
Modelling of univariate and bivariate change.
(A) Univariate latent change score models (LCSM) for response speed (left) and neural filtering (right). All paths denoted with Latin letters refer to freely estimated but constrained to be equal parameters of the respective measurement models. Greek letters refer to freely estimated parameters of the structural model. Highlighted in black is the estimated mean longitudinal change from T1 to T2. Scatterplots in the top-left corner illustrate how capturing T1 and T2 neural and behavioural functioning as latent factors improves their respective test–retest reliability. (B) LCSM relating 2-year changes in neural filtering strength to changes in response speed. Black arrows indicate paths or covariances of interest. Solid black arrows reflect freely estimated and statistically significant effects, while dashed black arrows reflect non-significant effects. All estimates are standardised. Grey arrows show paths that were freely estimated or fixed as part of the structural model but that did not relate to the main research questions. For visual clarity, manifest indicators of the measurement model and all symbols relating to the estimated mean structure are omitted but are identical to those shown in panel (A). ***p<0.001, **p<0.01, *p<0.05, ✝p=0.08. (C) Scatterplots of model-predicted factor scores that refer to the highlighted paths in panel (B). Top panel shows that baseline-level neural filtering did not predict 2-year change in behavioural functioning, while bottom panel shows the absence of a significant change–change correlation. All panels include data of N = 105 individuals.

Full bivariate latent change score model of response speed and neural filtering.
Model estimated brain–behaviour baseline–change (via regression) and change–change (via their covariance) relationship. First-order latent variables (T1, T2) were constructed from manifest neural and behavioural metrics extracted from the first and second half of the experiment, respectively. M, mean; COV, covariance; REG, regression path; RES, residual; λ,factor loading; Δ, change. Residuals and covariances of manifest variables of the same metric were each set to be equal.

Change in neural filtering does not predict change in response speed.
Bottom: N = 105 individuals sorted by their change in neural filtering. Positive values indicate an increase in neural filtering over time, while negative values indicae a decrease. Applying the same ordering to individuals’ longitudinal change in response speed (top) does not uncover a systematic relationship in cross-domain changes.
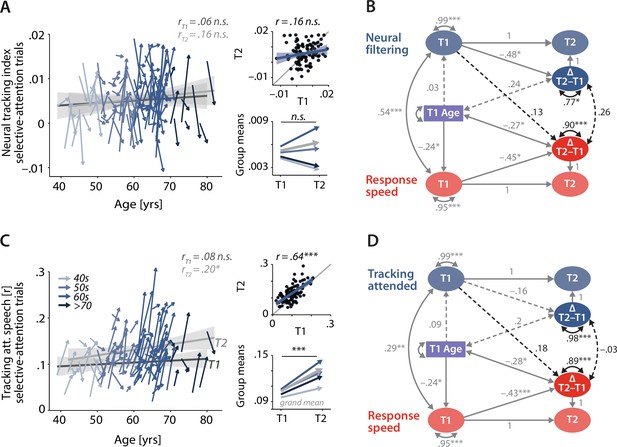
Control analyses corroborate the independence of neural and behavioural trajectories under selective attention.
Cross-sectional and longitudinal change in neural filtering (A) and neural tracking of attended speech (C) averaged across selective-attention trials, only. Coloured vectors (colour coding four age groups for illustrative purposes, only) in the left subpanels show individual T1–T2 change along with the cross-sectional trend plus 95% confidence interval (CI) separately for T1 (dark grey) and T2 (light grey). Top right: correlation of T1 and T2 as a measure of test–retest reliability along with the 45° line (grey) and individual data points (black circles). Bottom right: mean longitudinal change per age group and grand mean change (grey). (B, D) Latent change score model (LCSM) relating 2-year changes in neural filtering (B) /neural tracking (D) strength to changes in response speed. Black arrows show the paths or covariances of interest that were freely estimates, while grey arrows show paths that were freely estimated or fixed as part of the structural model but did not relate to the main research questions. Solid arrows indicate statistically significant effects, while dashed arrows reflect non-significant paths. All estimates are standardised. ***p<0.001, **p<0.01, *p<0.05. All panels include data from N = 105 individuals.
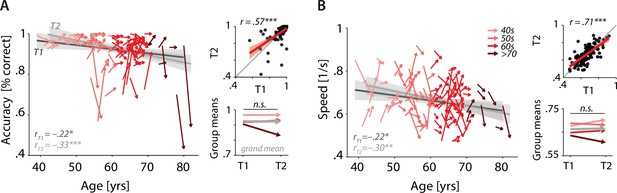
Cross-sectional and longitudinal change in accuracy and response speed averaged across selective-attention trials, only.
Coloured vectors (colour coding four age groups for illustrative purposes, only) in the left subpanels show individual T1–T2 change in N = 105 individuals along with the cross-sectional trend plus 95% confidence interval (CI) separately for T1 (dark grey) and T2 (light grey). Top right: correlation of T1 and T2 as a measure of test–retest reliability along with the 45° line (grey) and individual data points (black circles). Bottom right: mean longitudinal change per age group and grand mean change (grey).

Cross-sectional and longitudinal change in neural tracking of ignored speech averaged across selective-attention trials, only.
Coloured vectors (colour coding four age groups for illustrative purposes, only) in the left subpanels show individual T1–T2 change in N = 105 individuals along with the cross-sectional trend plus 95% confidence interval (CI) separately for T1 (dark grey) and T2 (light grey). Top right: correlation of T1 and T2 as a measure of test–retest reliability along with the 45° line (grey) and individual data points (black circles). Bottom right: mean longitudinal change per age group and grand mean change (grey).

Modelling of univariate and bivariate change.
(A) Univariate latent change score models for response speed (left) and neural filtering (right). All paths denoted with Latin letters refer to freely estimated but constrained to be equal parameters of the respective measurement models. Greek letters refer to freely estimated parameters of the structural model. Highlighted in black is the estimated mean longitudinal change from T1 to T2. Scatterplots in the top left corner illustrate how capturing T1 and T2 neural and behavioural functioning as latent factors improves their respective test-retest reliability. (B) Latent change score model (LCSM) relating two-year changes in neural filtering strength to changes in response speed. Black arrows indicate paths or covariances of interest. Solid black arrows reflect freely estimated and statistically significant effects, dashed black arrows reflect non-significant effects. All estimates are standardised. Grey arrows show paths that were freely estimated or fixed as part of the structural model but that did not relate to the main research questions. For visual clarity, manifest indicators of the measurement model and all symbols relating to the estimated mean structure are omitted but are identical to those shown in panel A. ***p<.001, **p<.01, *p<.05, p=.08. (C) Scatterplots of model-predicted factor scores that refer to the highlighted paths in panel B. Top panel shows that baseline-level neural filtering did not predict two-year change in behavioural functioning, bottom panel shows the absence of a significant change-change correlation.
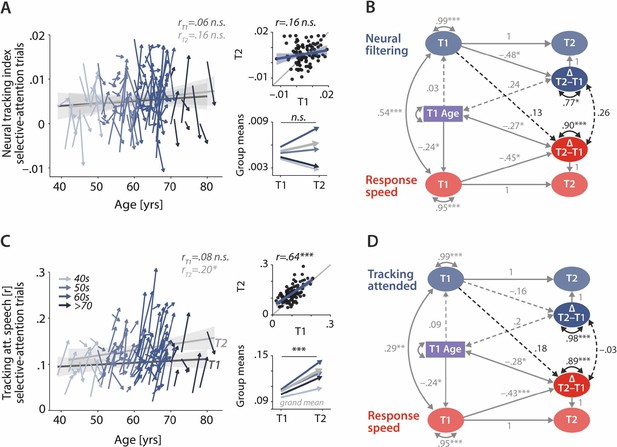
Control analyses corroborate the independence of neural and behavioural trajectories under selective attention.
Cross-sectional and longitudinal change in neural filtering (A) and neural tracking of attended speech (C) averaged across selective-attention trials, only. Coloured vectors (colour-coding four age groups for illustrative purposes, only) in the left subpanels show individual T1–T2 change along with the cross-sectional trend plus 95% confidence interval (CI) separately for T1 (dark grey) and T2 (light grey). Top right, correlation of T1 and T2 as measure of test-retest reliability along with the 45° line (grey) and individual data points (black circles). Bottom right, mean longitudinal change per age group and grand mean change (grey). (B, D) Latent change score model (LCSM) relating two-year changes in neural filtering (B) /neural tracking (D) strength to changes in response speed. Black arrows show the paths or covariances of interest that were freely estimates, grey arrows show paths that were freely estimated or fixed as part of the structural model but did not relate to the main research questions. Solid arrows indicate statistically significant effects, dashed arrows reflect nonsignificant paths. All estimates are standardised. ***p<.001, **p<.01, *p<.05.
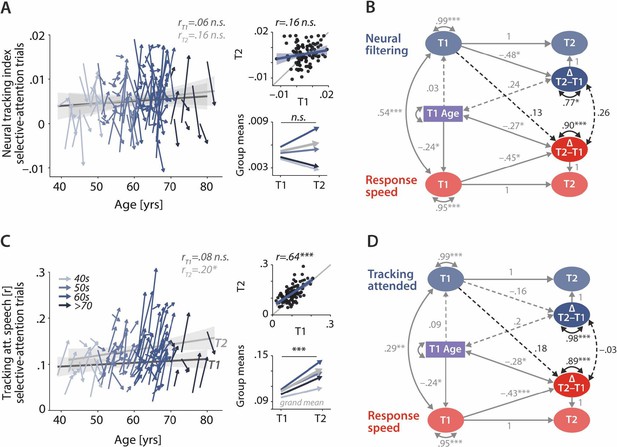
Control analyses corroborate the independence of neural and behavioural trajectories under selective attention.
Cross-sectional and longitudinal change in neural filtering (A) and neural tracking of attended speech (C) averaged across selective-attention trials, only. Coloured vectors (colour-coding four age groups for illustrative purposes, only) in the left subpanels show individual T1–T2 change along with the cross-sectional trend plus 95% confidence interval (CI) separately for T1 (dark grey) and T2 (light grey). Top right, correlation of T1 and T2 as measure of test-retest reliability along with the 45° line (grey) and individual data points (black circles). Bottom right, mean longitudinal change per age group and grand mean change (grey). (B, D) Latent change score model (LCSM) relating two-year changes in neural filtering (B) /neural tracking (D) strength to changes in response speed. Black arrows show the paths or covariances of interest that were freely estimates, grey arrows show paths that were freely estimated or fixed as part of the structural model but did not relate to the main research questions. Solid arrows indicate statistically significant effects, dashed arrows reflect nonsignificant paths. All estimates are standardised. ***p<.001, **p<.01, *p<.05.
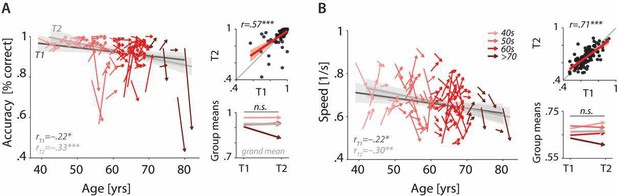
Cross-sectional and longitudinal change in listening behaviour under selective attention.

Cross-sectional and longitudinal change in neural tracking of ignored speech under selective attention.
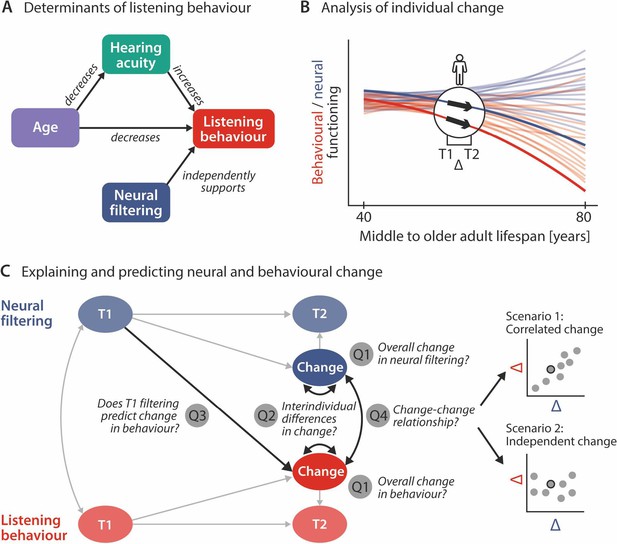
Schematic illustration of key assumptions and research questions.
(A) Listening behaviour at a given timepoint is shaped by an individuals’ sensory and neural functioning. Increased age decreases listening behaviour both directly, and indirectly via age-related hearing loss. Listening behaviour is supported by better neural filtering ability, independently of age and hearing acuity. (B) Conceptual depiction of individual two-year changes along the neural (blue) and behavioural (red) domain. Thin coloured lines show individual trajectories across the adult lifespan, thick lines and black arrows highlight two-year changes in a single individual. (C) Left, Schematic diagram highlighting the key research questions detailed in the introduction and how they are addressed in the current study using latent change score modelling. Right, across individuals, co-occurring changes in the neural and behavioural domain may be correlated (top) or independent of one another (bottom).
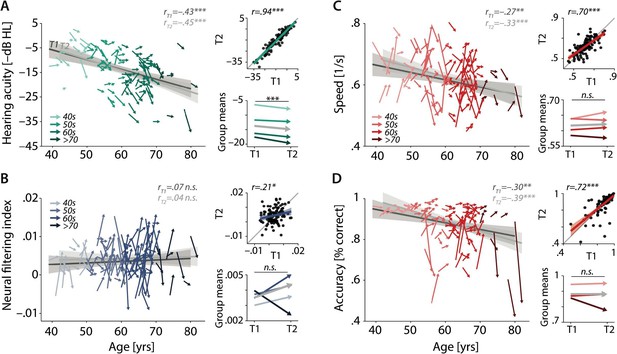
Characterizing cross-sectional and longitudinal change along the auditory sensory (A), neural (B), and behavioural (C, D) domain.
For each domain, coloured vectors (colour-coding four age groups for illustrative purposes, only) in the respective left subpanels show an individual’s change from T1 to T2 along with the cross-sectional trend plus 95% confidence interval (CI) separately for T1 (dark grey) and T2 (light grey). Top right subpanels: correlation of T1 and T2 as measure of test-retest reliability along with the 45° line (grey) and individual data points (black circles). Bottom right panels: Mean longitudinal change per age group (coloured vectors) and grand mean change (grey). Note that accuracy is expressed here as proportion correct for illustrative purposes, but was analysed logit-transformed or by applying generalized linear models.