Conformational dynamics of a nicotinic receptor neurotransmitter site
Figures
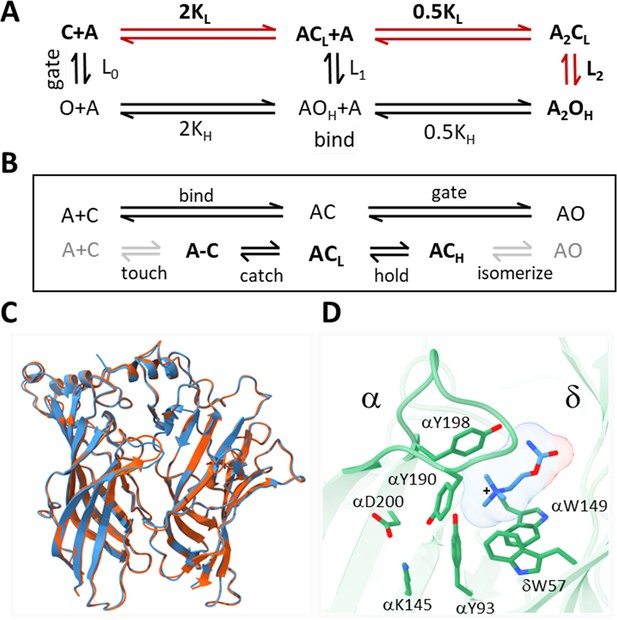
Acetylcholine receptor (AChR) activation.
(A) Traditional scheme. Vertical is ‘gating’ and horizontal is ‘binding;’ red, the main physiological pathway. The isomerization between closed-channel/low-affinity (CL) and open-channel/high-affinity (OH) conformations occurs with or without agonists (equilibrium constant Ln; n, number of bound ligands), is spontaneous (depends only on temperature), and global (on a ~μs time scale). Agonists (A) bind weakly to CL equilibrium association constant KL, free energy change ΔGL and strongly to OH (KH, ΔGH). The two orthosteric sites of adult AChRs are approximately equivalent and independent and there is no significant external energy, so by microscopic reversibility L2/L0 = (KH/KL)2 (Nayak and Auerbach, 2017). (B) Expansions of binding (top, ends with catch) and gating (bottom, starts with hold). The agonist diffuses to and contacts the target ('touch') to form an encounter complex (A–C); a local 'catch' rearrangement establishes the low affinity complex (ACL); a local ‘hold’ rearrangement establishes the high-affinity complex (ACH); the remaining protein domains rearrange (‘isomerize’) without a further change in affinity, to generate a conducting channel (AOH). Gray arrows, are steps that incur the same energy change for all agonists used in this study; black arrows, agonist-dependent free energy changes occur in catch (ΔGL) and in hold (ΔGH-ΔGL). (C) α-δ subunit extracellular domains; red, after toxin removal (6UWZ.pdb) and blue, apo (7QKO.pdb). There are no major deviations (Cα RMSD = 0.3 Å). (D) Closeup of the desensitized Torpedo α-δ subunit neurotransmitter site occupied by carbamylcholine (CCh, blue) (7QL6.pdb; Zarkadas et al., 2022). In this is H conformation, three aromatic groups in the α subunit (149-190-198) surround the agonist’s cationic center (+) together and provide most of the ACh binding energy (Purohit et al., 2014); the agonist’s tail points away from the α subunit (trans orientation).
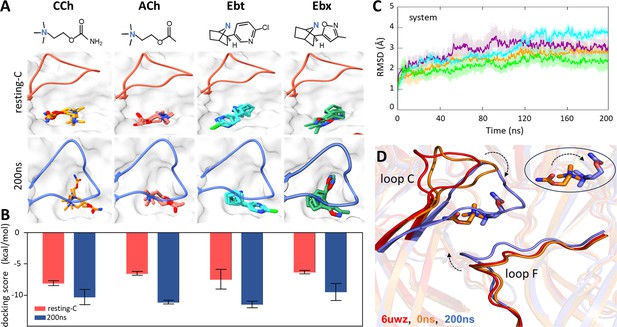
Agonist docking and loop dynamics.
(A) Top, agonists (blue, cationic center): carbamylcholine (CCh), acetylcholine (ACh), epibatidine (Ebt), and epiboxidine (Ebx). Bottom, α−δ site with docked agonists (top three poses). Resting-C, 6UVW.pdb minus toxin (red): loop C is up and agonist is cis; 200 ns, after simulation and removal of CCh (blue): loop C is down and agonist is trans. (B) Bottom, for all four agonists the docking scores (mean ± SD, n=3) were more favorable after simulation. (C) Cα root-mean-square deviation (RMSD) (mean ± SD, triplicates) are stable after ~120 ns (ACh, cyan; CCh, green; Ebt, orange; Ebx, purple). (D) Close-up of the CCh-occupied pocket. Red, resting-C; orange, equilibrated (0 ns molecular dynamics, MD); blue, after 200 ns MD. IN the simulations, loop C flops down (arrow), loop F moves in, and the agonist flips cis→trans (circled inset).
-
Figure 2—source data 1
Molecular dynamics (MD) simulation.
Average root-mean-square deviation (RMSD) values for protein, ligand, or the entire complex (protein + ligand) across individual MD simulation runs. SD, standard deviation; SEM, standard error of the mean.
- https://cdn.elifesciences.org/articles/92418/elife-92418-fig2-data1-v1.docx
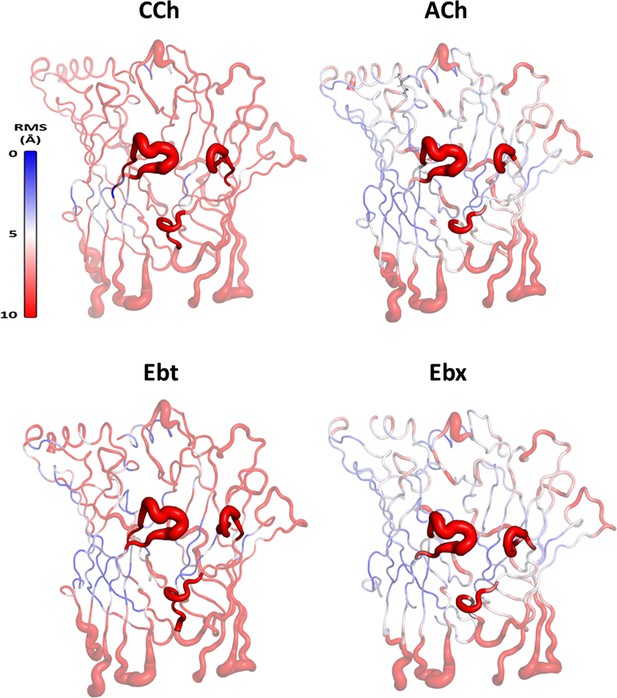
Root mean square fluctuation (RMSF) of Cα for the extracellular domain (ECD) of α-δ subunits.
RMSF for carbamylcholine (CCh), acetylcholine (ACh), epibatidine (Ebt), and epiboxidine (Ebx) (structures in Figure 4A). Greatest fluctuations are in loops C and F that surround the orthosteric pocket; red-blue is the greatest-least fluctuations.
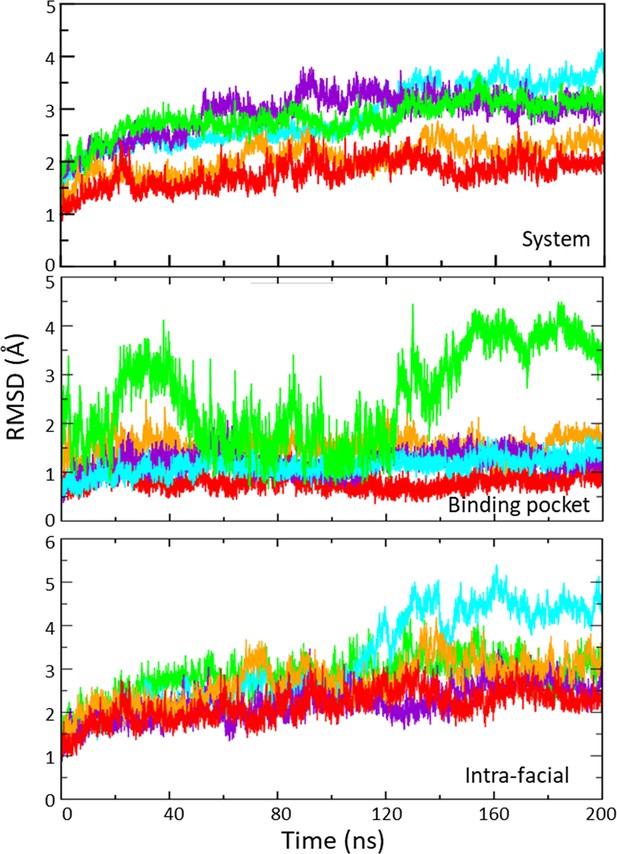
RMSD of pocket residues during molecular dynamics (MD) simulations.
The root-mean-square deviation (RMSD) of binding pocket residues relative to their initial positions over the course of 200 ns MD simulations for each agonist (carbamylcholine, CCh in red, epibatidine EBt in orange, epiboxidine EBx in purple, acetylcholine ACh in blue, and apo in green). Agonists stabilize pocket residues (middle) but intra-facial residues (near the transmembrane domain (TMD) and adjacent subunits; lower) fluctuate similarly with and without agonists.
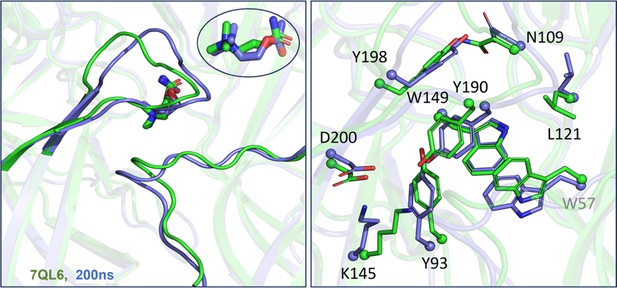
Alignment of molecular dynamics (MD) simulations (carbamylcholine, CCh).
Left, loops. An overlay of the final MD configuration with CCh (putative ACH; blue) and the cryo-EM desensitized (ADH) structure with CCh (7QL6.PDB, green) shows strong overlap in loops C (top left) and F (bottom right) (root-mean-square deviation, RMSD=0.3). Insert: the agonist (trans orientation) also overlaps. In experiments, ACH and ADH have the same CCh binding free energy. Right, side-chains. The final MD structure (blue) aligns approximately with the desensitized, high-affinity conformation of the pocket (green).
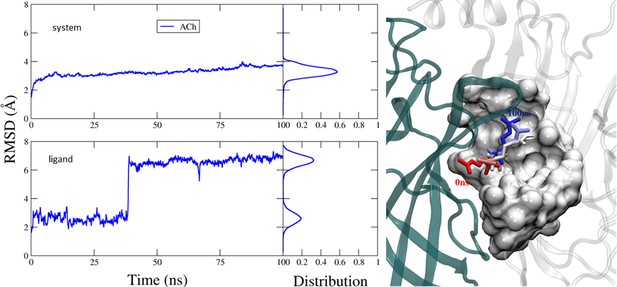
Molecular dynamics (MD) simulation of the pentameric system.
Left, root-mean-square-deviation (RMSD) of the pentamer (top) and the ligand (bottom), in complex with docked acetylcholine (ACh) (100 ns MD simulation). Side panels are distribution plots. Right, the m1-m2-m3 changes in ACh conformation in the orthosteric cavity. The agonist’s orientation flips from cis (m1, at 0 ns; red) to trans (m3, at 200 nS; blue). m2 is in between. Similar structural changes are apparent in the dimer.
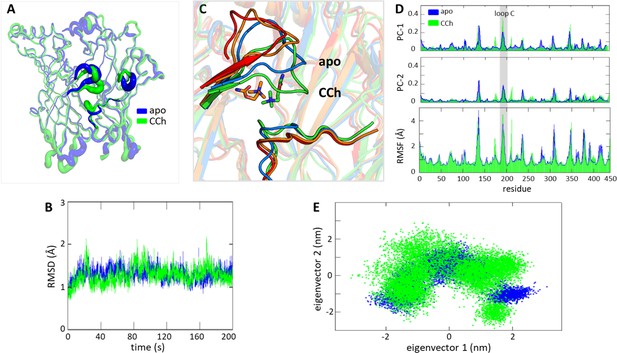
Conformational convergence between apo (blue) and with carbamylcholine (CCh) (green).
(A) Root mean square fluctuation (RMSF) of Cα extracellular domain (ECD) shows similar regions of fluctuations, loops C and F that surround the pocket. (B) Root-mean-square-deviation (RMSD) of the system Cα. (C) Closeup of the α−δ orthosteric pocket showing displacements of loop C (flop) and loop F; blue, apo; red, 6UWZ; orange CCh 0 ns; green, CCh 200 ns. (D) RMSF shows loop C movement (gray highlight) is closely aligned with key conformational shifts observed in the primary two principal components (PC1 and PC2). (E) A 2D scatter plot of the first two principal components derived from the simulation data for apo superimposed with CCh; cluster overlaps indicate that similar stable conformations were explored.
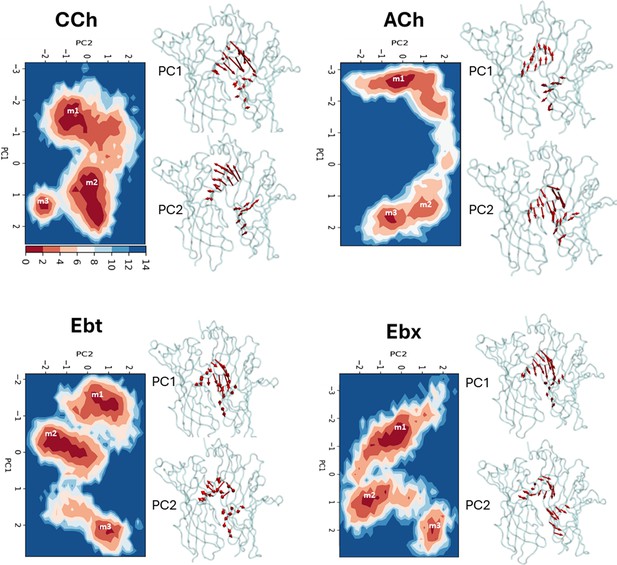
Principal component analysis (PCA).
Left, for each agonist a plot of PC-1 versus PC-2, the first two principal components that capture the maximum variance in the trajectory (Figure 3—source data 1). Colors represent free energy value in kcal/mol (scale, upper left, bottom). For all agonists, there are three energy minima (darkest red) - m1, m2, and m3 - that correspond to different conformations of the neurotransmitter site. Right, ‘porcupine’ plots indicating that the direction and magnitude of changes PC-1 versus PC-2 is in loops C and F. From energy comparisons (Figure 4, Figure 4—source data 1) and temporal sequences (Figure 3—figure supplement 2, Figure 4—source data 1) we hypothesize that m1 represent state ACL, m3 represents state ACH, and m2 is an intermediate state in the L→H, hold transition (Figure 1B).
-
Figure 3—source data 1
Cumulative contribution of principal components (PCs) to the variance in molecular dynamics (MD) Simulations.
The table presents the percentage of the total variance in the molecular motion that is accounted for by the first ten principal components in five different simulation conditions: Apo; CCh, carbamylcholine; ACh, acetylcholine; Ebt, epibatidine, and Ebx, epiboxidine. Each row corresponds to a principal component (PC1 through PC10), while the columns list the cumulative percentage of motion variance that the PCs account for in each simulation condition.
- https://cdn.elifesciences.org/articles/92418/elife-92418-fig3-data1-v1.docx
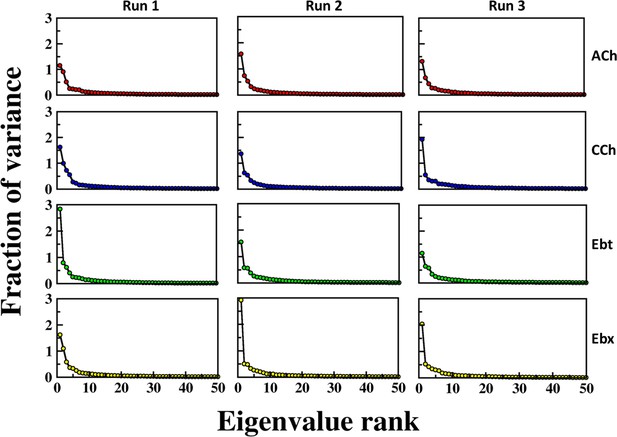
Fraction of variance explained by eigenvalue rank for different simulation runs and conditions.
The plots show the fraction of variance (y-axis) as a function of eigenvalue rank (x-axis) for principal component analysis (PCA). Columns, three independent simulation runs (RUN1, RUN2, and RUN3); rows, four different conditions or datasets, indicated by different colors (red, blue, green, yellow). Each plot demonstrates that the first few principal components capture the majority of the variance, with a rapid decrease in the fraction of variance as eigenvalue rank increases. This pattern is consistent across all runs and conditions, indicating the robustness and reproducibility of the PCA results.
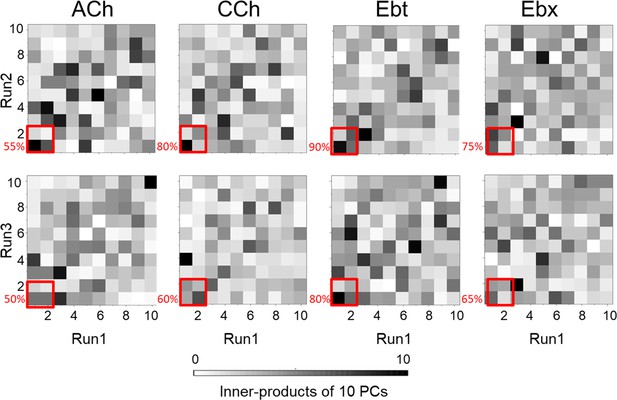
Inner product heatmaps of principal components (PCs) from independent molecular dynamics (MD) simulations.
The heatmaps display the inner product values of the top 10 PCs obtained from pairwise comparisons of three independent simulation runs for four systems - ACh, acetylcholine; CCh, carbamylcholine; Ebt, epibatidine and Ebx, epiboxidine. The color gradient from white to black indicates the magnitude of the inner product, with white representing an inner product of 0 (no correlation) and black representing an inner product of 10 (perfect correlation). The red boxes in the heatmaps correspond to PC-1 and PC-2 (percentage similarity between the runs indicated).
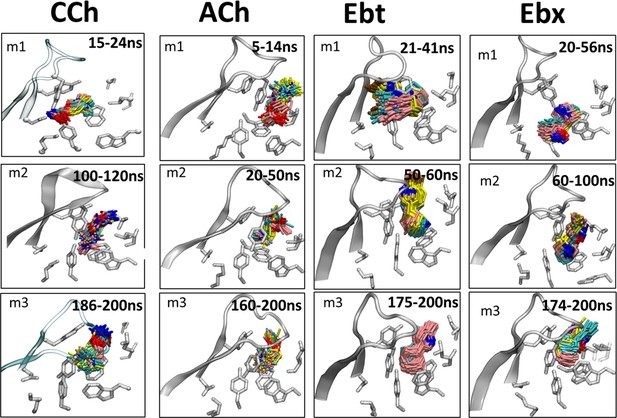
Cluster analysis of ligand poses.
Three prominent poses of the ligand for each of the three energy minima (m1, m2, m3) were identified using cluster analysis in visual molecular dynamics (VMD). The top three clusters, with root-mean-square-deviation (RMSD) of ≤1 Å each, display similar ligand orientations across the energy minima. The analysis was performed on frames extracted from the bottom of the wells apparent in the principal component analysis (PCA). The temporal order in simulations is: m1 (start)<m2 (middle) <m3 (end). The agonists are carbamylcholine (CCh), acetylcholine (ACh), epibatidine (Ebt), and epiboxidine (Ebx). Three prominent clusters are colored by heteroatom with carbons in cyan, pink, and yellow, respectively.
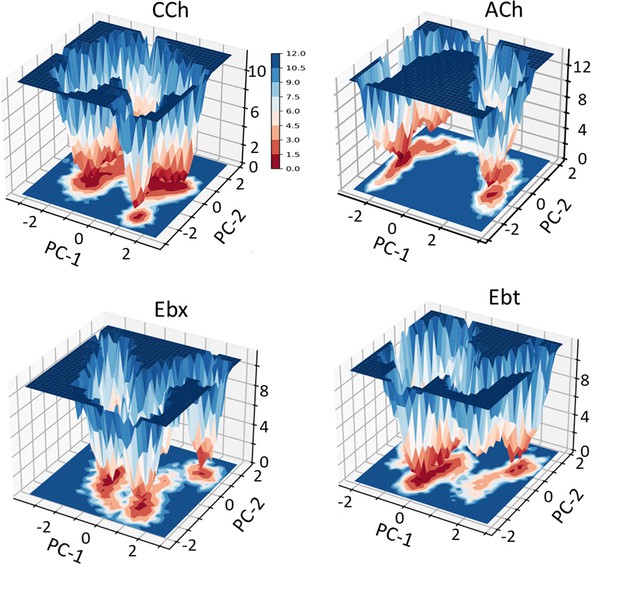
Free energy landscapes as a function of PC1 and PC2.
The color scale represents the free energy values in kcal/mol; deeper wells (red) indicate more stable and shallower wells (blue) indicate less stable. All four agonists show three stable states (m1, m2, and m3). CCh, carbamylcholine; ACh, acetylcholine; Ebt, epibatidine and Ebx, epiboxidine.
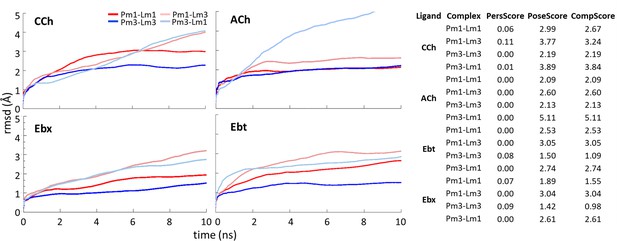
Ligand-protein Complexes during binding pose metadynamics (BPMD).
An average root-mean-square-deviation (RMSD) plot of various ligand-protein complexes over the course of 10 × 10 ns metadynamics runs using PoseScore and PersScore derived values for four different agonists. The lines represent different ligand-protein combinations: Pm1-Lm1 (protein in m1 and ligand in m1, red), Pm1-Lm3 (protein in m1 and ligand in m3, light red), Pm3-Lm3 (protein in m3 and ligand in m3, blue), and Pm3-Lm1 (protein in m3 and ligand in m1, light blue). The plots indicate that the Pm3-Lm3 complexes show the least fluctuation, suggesting higher stability, while Pm1-Lm1 and the cross-docked complexes (Pm1-Lm3 and Pm3-Lm1) exhibit lower stability. Table, BPMD assesses the stability of binding poses using PoseScore and PersScore. PoseScore measures the RMSD of the ligand’s heavy atoms relative to their initial coordinates, with lower values indicating higher stability. PersScore measures the persistence of important contacts, such as hydrogen bonds and pi-pi interactions, between the ligand and protein residues, with higher values indicating more stable complexes. The Composite Score (CompScore) combines PoseScore and PersScore (CompScore = PoseScore - 5 × PersScore) to provide an overall stability assessment, with lower CompScores indicating more stable ligand-protein complexes.
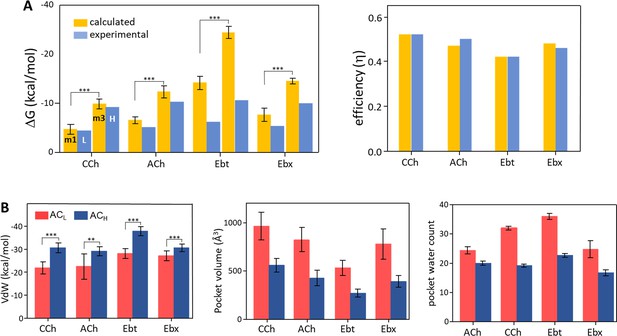
Binding free energies and pocket properties.
(A) Calculated (yellow) versus experimental (blue) binding free energies for four agonists (structures in Figure 2A, top) (Figure 4—source data 1). Poisson-Boltzmann Surface Area (PBSA) calculations were done on clusters selected from m1 and m3 minima of principal component analysis (PCA) plots (Figure 3; Figure 3—figure supplement 2). Left, absolute ΔG, and right, efficiency (1-ΔGL/ΔGH). The agreement in efficiencies supports the hypothesis that m1 represents ACL and m3 represents ACH (B) In L→H (red→blue), VdW interactions (left) increase, pocket volume (center) decreases, and the number of water molecules in the pocket (right) decreases. Overall, the pocket stabilizes, compacts, and de-wets.
-
Figure 4—source data 1
Table of calculated and experimental binding energies (kcal/mol).
Agonists, see Figure 2A. In silico (calculated) values were from frames from each principal component analysis (PCA) population (m1, m2, and m3; Figure 3) after cluster analysis of ligand orientation. In vitro (experimental) values were from electrophysiology measurements. Window, frame times for each population; n frames, number of simulation frames from three prominent clusters chosen for MM-PBSA analysis;ΔH, change in binding enthalpy; ΔS change in binding entropy (T, absolute temperature);ΔH, change in Gibbs free energy (ΔG=ΔH-TΔS); η, efficiency (1-ΔGLA/ΔGHA). SD, standard deviation; SEM, standard error of the mean. Data are shown as bar graphs in Figure 4.
- https://cdn.elifesciences.org/articles/92418/elife-92418-fig4-data1-v1.docx
-
Figure 4—source data 2
MM-Poisson-Boltzmann Surface Area (PBSA) components of the free energy calculation (see Figure 4).
EEL, electrostatic; VdW, van der Waals; EPB, electrostatic Poisson-Boltzmann; ENPOLAR, nonpolar solvation energy; ΔG gas, gas-phase free energy; ΔG solv, solvation free energy; SD, standard deviation; SEM, standard error of the mean.
- https://cdn.elifesciences.org/articles/92418/elife-92418-fig4-data2-v1.docx
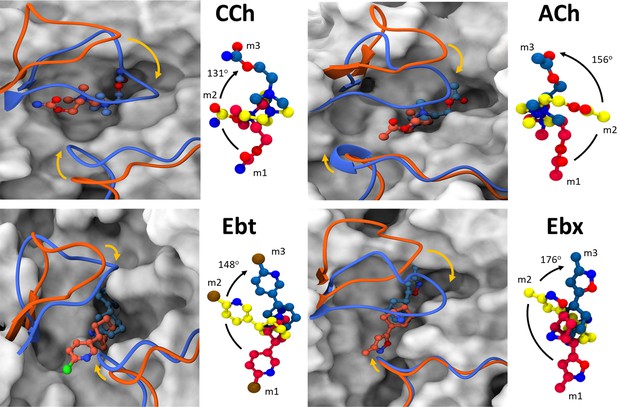
Agonist and loop movements in the hold (flip and flop).
(A) Left, superimposed cartoons of ACL (m1; orange) and ACH (m3; blue). Loop C is upper left and loop F is lower right. In L→H (orange→blue) there is a cis→trans reorientation of the agonist (flip) and a downward movement of loop C (flop, arrow). Right, agonist structure m1 (red) versus m2 (yellow) versus m3 (blue). Degree pertains to the m1→m3 pivot angle of the agonist.
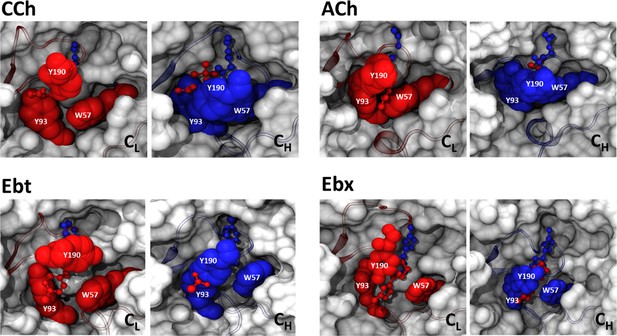
Conformational change of the pocket cavity accommodates agonist re-orientation.
Red, low-affinity conformation (ACL); blue, high-affinity conformation (ACH). In ACL the ligand is cis and aromatic residues αY190, αY93, and δW57 are spread to accommodate the agonist’s tail. In ACH the ligand is trans and αY190 is positioned to fill the αY93-δW57 gap, creating a unified surface that restrains the ligand’s tail. Key residue sidechains are shown as spheres; the agonist is ball-and-stick.
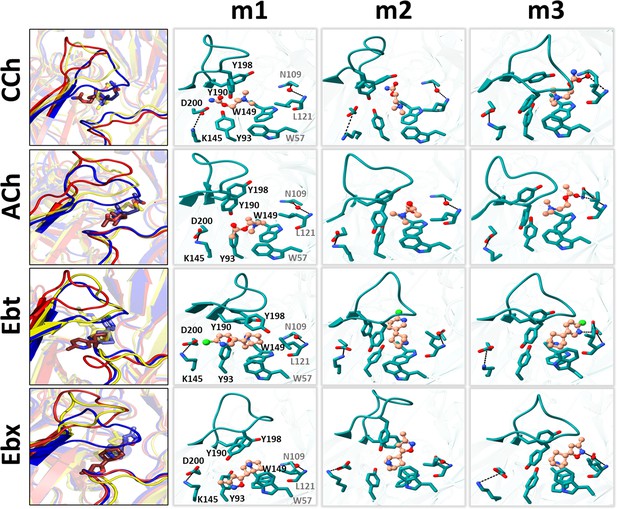
Representative snapshots in L→H (hold).
Left, rearrangements of loop C, loop F, and the ligand (red, m1; yellow, m2; blue, m3); right, residue and ligand orientations. m1 is ACL, m2 is an intermediate state, m3 is ACH. (Figure 1B). In m1, a functional group in the agonist tail interacts with αY93 (all agonists) and αD200 (only CCh, and Ebt). The position and orientation of αW149 relative to N+ of the agonist remains nearly unchanged m1→m2→m3 and serves as a fulcrum for the cis→trans flip (see Figure 5). In m2, the functional nitrogen at the agonist tail (CCh, Ebt, and Ebx) interacts with the hydroxyl group of αY198. For all ligands, αY190 repositioning and loop C flop (m1→m3) are correlated. In m3, the agonist fully flips to trans, facilitating VdW interactions, de-wetting, and the formation of water-mediated hydrogen bonds with the reactive group at its tail with δN109/δL121 backbone (loop E) via a structural water.
-
Figure 6—source data 1
Residue distances.
Distance measurements (Å) between functional groups. αK145 is to the companion polar group; N+ is to the center of the indole ring; loop C is Cα of αC192 compared apo; m1, m2, and m3 structures taken from plateau regions of the trajectories.
- https://cdn.elifesciences.org/articles/92418/elife-92418-fig6-data1-v1.docx
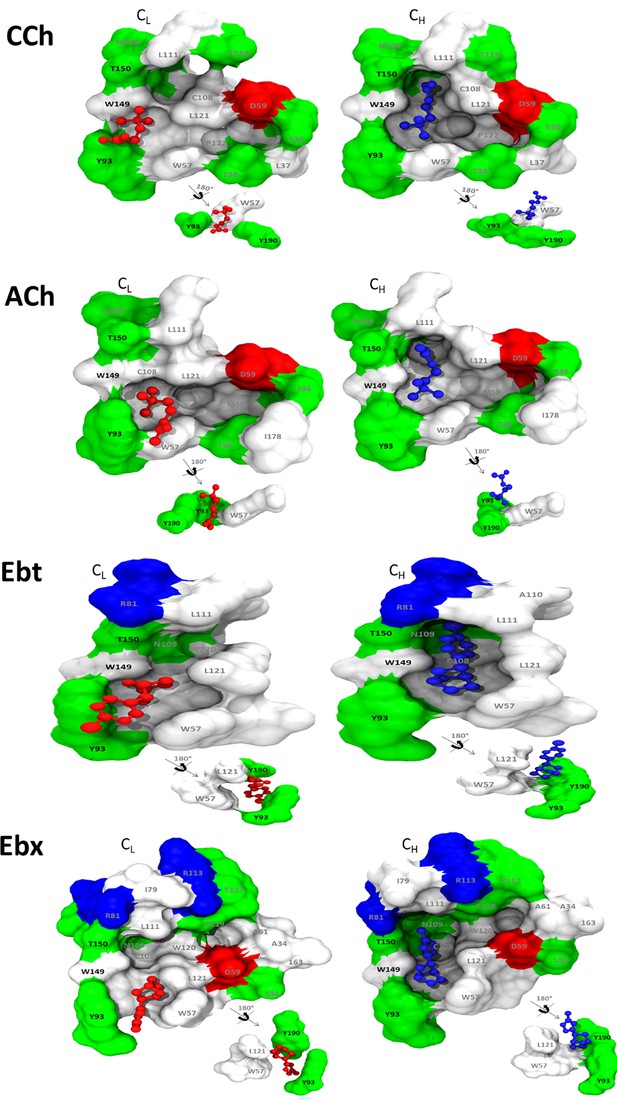
Binding cavities (α−δ subunit interface).
Left. Agonist (red) is low affinity (red: ACL, m1). Right, the agonist is high affinity (blue; ACH, m3). All agonists (see Figure 5) rotate about the cationic center (N+)-αW149 interface, so that the tail flips from being near αY93 in L (cis) to being in contact with the complementary-subunit surface in H (trans).
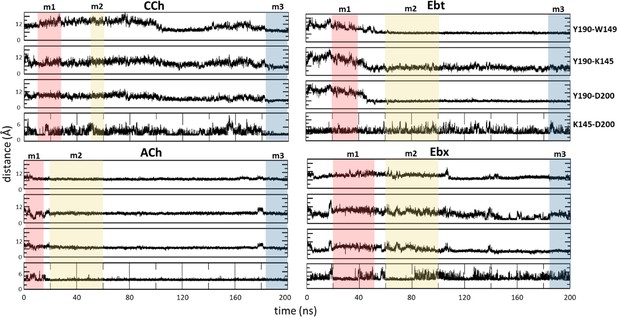
Key residue distances over the course of molecular dynamics (MD) simulation.
The distances in angstroms between specific functional groups of the hydroxyl group of αY190 to the indole of αW149, the amine of αK145, the carboxyl of αD200, and the salt-bridge distance between αK145 and αD200. The bottom energy minima for each system are highlighted: m1, red; m2, yellow; m3, blue.
Videos
Early Cis-to-Trans Transition of CCh in the AChR Binding Pocket.
Stabilization of agonist trans orientation and loop C displacement during the hold transition.
Cis-to-Trans Transition of Ebx in the AChR Binding Pocket from L-to-H.
Additional files
-
Supplementary file 1
Supplementary material.
- https://cdn.elifesciences.org/articles/92418/elife-92418-supp1-v1.docx
-
MDAR checklist
- https://cdn.elifesciences.org/articles/92418/elife-92418-mdarchecklist1-v1.pdf