Key determinants of the dual clamp/activator function of Complexin
Figures
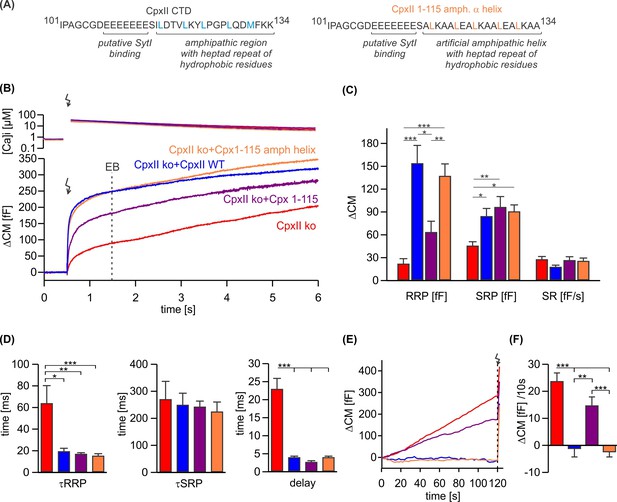
An unrelated amphipathic helix functionally substitutes for the last 19 amino acids of CpxII C-terminal domain (CTD).
(A) Primary sequence of CpxII’s CTD (left) and the mutant CpxII 1–115 amphipathic α-helix (right). (B) Mean [Ca]i levels (upper panel) and the corresponding membrane capacitance response (lower panel) from CpxII knockout (ko) cells (red n=15), and those expressing either wild-type (WT) CpxII (blue n=15), the CpxII 1–115 mutant (purple n=14), or the CpxII 1–115 amphipathic helix mutant (orange n=16). (C) Amplitudes of the readily releasable pool (RRP), the slowly releasable pool (SRP), and the rate of sustained release (SR). CpxII 1–115 amphipathic helix mutant fully supports exocytosis like the WT protein. (D) The time constants for the exocytotic burst (EB) components (τRRP and τSRP), and the exocytotic delay are rescued by the CpxII mutants like the WT protein. (E) Mean premature secretion of the tested groups at similar submicromolar [Ca]i before triggering the flash response shown in (B). (F) The rate of tonic exocytosis (determined at similar [Ca2+]i: in nM, CpxII ko: 789±120; CpxII ko+CpxII: 777±57; CpxII ko+CpxII 1–115: 739±84; CpxII ko+CpxII1-115 amph helix: 796±64) is significantly reduced with CpxII and CpxII 1–115 amph helix mutant but not with the truncated CpxII 1–115 mutant. ANOVA or Kruskal-Wallis followed by corresponding post hoc test. **p<0.01; ***p<0.001. Error bars indicate mean ± SEM.
-
Figure 1—source data 1
Excel file containing quantitative data.
- https://cdn.elifesciences.org/articles/92438/elife-92438-fig1-data1-v1.xlsx
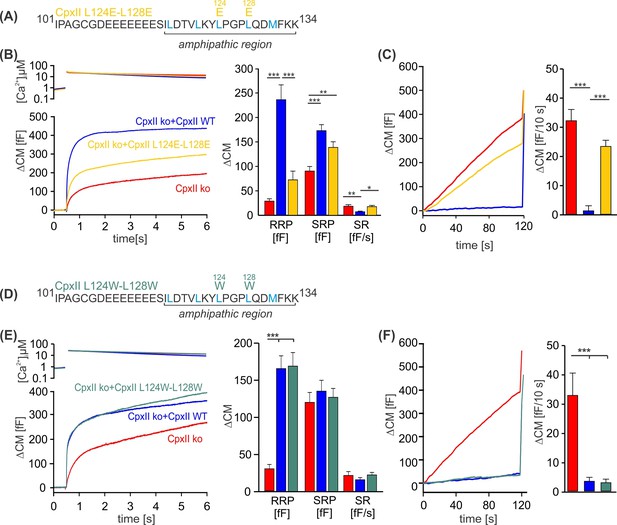
Disrupting the hydrophobic face of the amphipathic helix impairs the ability of the CpxII C-terminal domain (CTD) to prevent premature release.
(A, D) Primary sequences depicting the point mutations L124E-L128E (A) and L124W-L128W (D) within the amphipathic region of CpxII CTD. (B, E) Mean [Ca]i levels (upper panel) and the corresponding ΔCM (lower panel) from CpxII knockout (ko) cells (red n=25), and those expressing CpxII wild-type (WT) (blue n=25), or the mutants CpxII L124E-L128E (yellow n=26) in (B), and CpxII ko cells (red n=18) or those expressing the CpxII WT protein (blue, n=15) or CpxII LL-WW (dark green, n=12) in (D). Mean amplitudes of the readily releasable pool (RRP), slowly releasable pool (SRP), and sustained rate (SR) show a significant reduction in the size of the exocytotic burst (EB) by the CpxII L124E-L128E mutant, whereas CpxII L124W-L128W rescued the synchronized exocytosis like CpxII WT. (C, F) Average tonic exocytosis at similar submicromolar [Ca]i levels. Note that the mutant CpxII L124W-L128W fully clamps premature secretion like the CpxII WT protein, but the CpxII L124E-L128E mutant fails to do so. ANOVA or Kruskal-Wallis followed by corresponding post hoc test. *p<0.05; **p<0.01; ***p<0.001. Error bars indicate mean ± SEM.
-
Figure 2—source data 1
Excel file containing quantitative data.
- https://cdn.elifesciences.org/articles/92438/elife-92438-fig2-data1-v1.xlsx
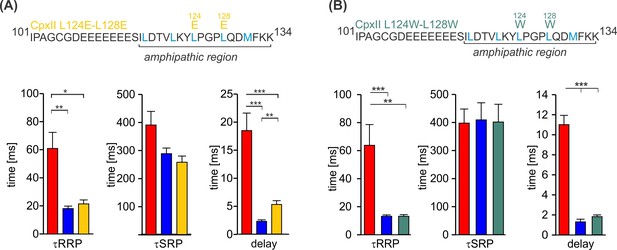
The CpxII C-terminal domain (CTD) mutants CpxII L124E-L128E and CpxII L124W-L128W rescue the kinetics of synchronized exocytosis.
(A, B) The kinetics of synchronized exocytosis as well as the delay of the stimulus-secretion coupling of CpxII knockout (ko) cells (red) are fully restored by the CpxII L124E-L128E (yellow) as well as the CpxII L124W-L128W mutant (green) when compared with expression of the CpxII wild-type (WT) protein (blue), consistent with the view that exocytosis timing is determined by the functionally intact N-terminal domain (NTD). ANOVA or Kruskal-Wallis followed by corresponding post hoc test. *p<0.05; ***p<0.001. Error bars indicate mean ± SEM.
-
Figure 2—figure supplement 1—source data 1
Excel file containing quantitative data.
- https://cdn.elifesciences.org/articles/92438/elife-92438-fig2-figsupp1-data1-v1.xlsx
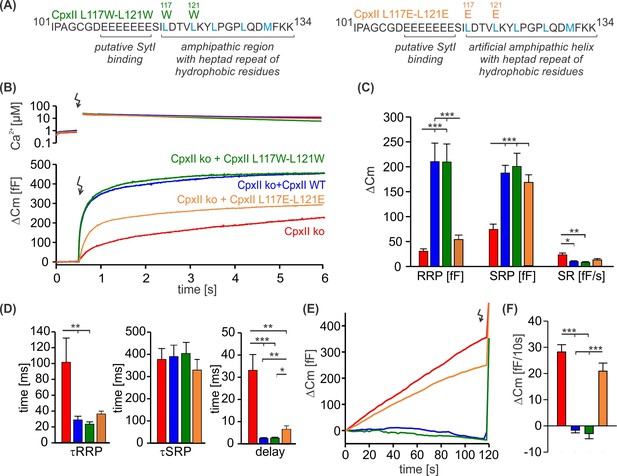
The hydrophobic face of CpxII’s amphipathic helix is essential to suppress premature release.
(A) Primary sequences showing point mutations L117W-L121W and L117E-L121E within the amphipathic α-helix of CpxII C-terminal domain (CTD). (B) Mean [Ca]i levels (upper panel) and the corresponding average increase in the membrane capacitance from CpxII knockout (ko) cells (red, n=26), and those expressing CpxII wild-type (WT) (blue, n=25), or the mutants CpxII L117E-L121E (yellow, n=25), or CpxII L117W-L121W (dark green, n=14). (C) Mean amplitudes of the readily releasable pool (RRP), slowly releasable pool (SRP), and sustained rate (SR) show a significant reduction for the CpxII L117E-L121E mutant while CpxII L117W-L121W rescues the synchronized exocytosis like the expressed CpxII WT protein. (D) All mutant variants restore exocytosis timing of CpII ko cells like the CpxII WT protein. (E, F) Average tonic exocytosis at similar submicromolar [Ca]i levels. The CpxII L117E-L121E mutant failed to rescue premature secretion phenotype of CpxII ko cells, but CpxII L117W-L121W mutant hindered it like the CpxII WT protein. ANOVA or Kruskal-Wallis followed by corresponding post hoc test. ***p<0.001. Error bars indicate mean ± SEM.
-
Figure 2—figure supplement 2—source data 1
Excel file containing quantitative data.
- https://cdn.elifesciences.org/articles/92438/elife-92438-fig2-figsupp2-data1-v1.xlsx
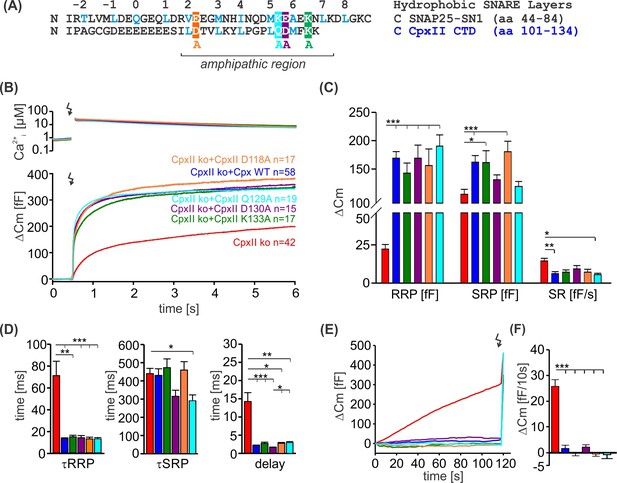
Alanine substitutions on the polar face of CpxII C-terminal domain (CTD) have no impact on its clamping ability.
(A) Primary sequences of SNAP-25 SN1 and CpxII CTD showing the positions of the alanine substitutions (subsequent panels refer to the same color coding). (B) Mean [Ca]i levels (upper panel) and the corresponding membrane capacitance increase from CpxII knockout (ko) cells (red, n=42), and those expressing either CpxII wild-type (WT) (blue, n=58), or the mutants CpxII D118A (yellow, n=17) CpxII Q129A (pink, n=19), CpxII D130A (violet, n=15), CpxII K133A (green, n=17). (C) When compared with expression of the CpxII WT protein, all CpxII mutants fully support an undiminished exocytotic burst, without changing either the readily releasable pool (RRP), slowly releasable pool (SRP), or the sustained release (SR). (D) Kinetic parameters of the synchronized exocytosis are rescued by CpxII and its mutant variants. (E, F) CpxII mutants suppress premature vesicle exocytosis at submicromolar calcium concentrations with similar efficacy as the CpxII WT protein. ANOVA or Kruskal-Wallis followed by corresponding post hoc test. **p<0.01; ***p<0.001. Error bars indicate mean ± SEM.
-
Figure 2—figure supplement 3—source data 1
Excel file containing quantitative data.
- https://cdn.elifesciences.org/articles/92438/elife-92438-fig2-figsupp3-data1-v1.xlsx
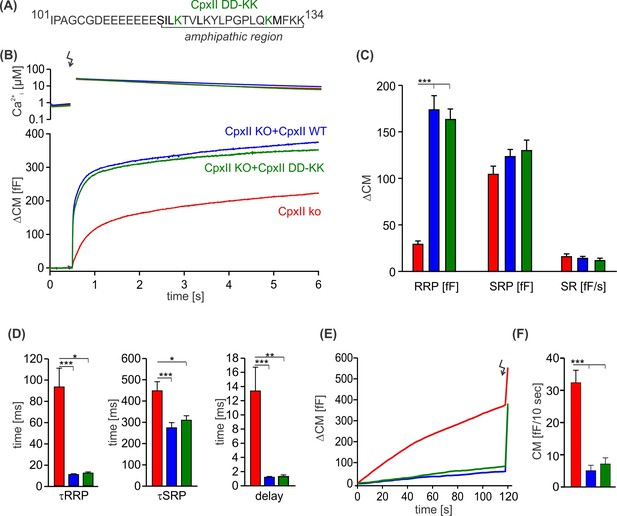
The charge reversal mutation D118K-D130K does not affect the inhibitory function of the CpxII C-terminal domain (CTD).
(A) Primary sequence of CpxII CTD showing the double point mutation D118K-D130K. (B) Mean [Ca]i levels (upper panel) and the corresponding average increase in capacitance measurements (CM) from CpxII knockout (ko) cells (red, n=27), and those expressing either CpxII wild-type (WT) (blue, n=29) or the mutant CpxII DD-KK (green, n=14). (C) The D118K-D130K mutant fully rescued all component of the exocytotic burst and the sustained rate (SR), like the expression of the CpxII WT protein. (D–F) Kinetic parameters of the synchronized exocytosis (D) and the suppression of premature vesicle exocytosis (E, F) were unaffected by the CpxII mutant. ANOVA or Kruskal-Wallis followed by corresponding post hoc test. *p<0.05; ***p<0.001. Error bars indicate mean ± SEM.
-
Figure 2—figure supplement 4—source data 1
Excel file containing quantitative data.
- https://cdn.elifesciences.org/articles/92438/elife-92438-fig2-figsupp4-data1-v1.xlsx
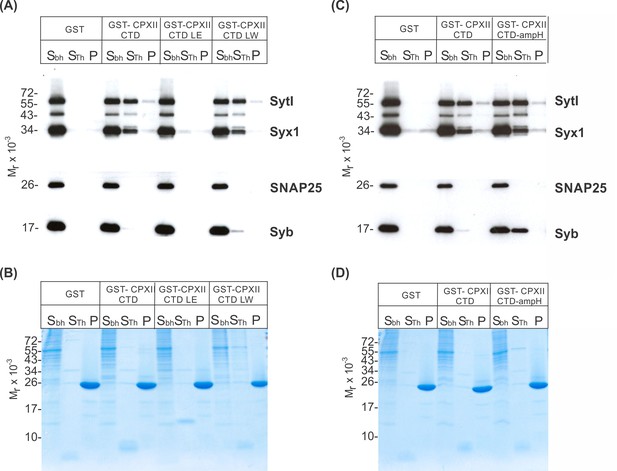
CpxII-C-terminal domain (CTD) and its mutant variants differentially interact with SNAREs and SytI.
(A, C) The GST-CpxII CTD fusion protein co-precipitates SytI and Syx1a from detergent extract of mouse brain. Substitution of leucine residues with glutamate residues (CpxII-CTD L124E-L128E, LE) abolishes any binding to CpxII CTD (A). Substitution with tryptophan residues (CpxII-CTD L124W-L128W, LW), instead, or replacement of the entire CpxII CTD with an unrelated amphipathic helix (CpxII-CTD-ampH, C) is tolerated. Samples were eluted from the column by thrombin cleavage. Equal volumes of supernatant (Sbh), the thrombin-eluted (STh), and the non-eluted fraction (P) were analyzed by SDS-PAGE (12% gel) and western blotting with antibodies against the indicated antigens. No binding to GST alone could be detected. (B, D) Corresponding Coomassie gels documenting the integrity of the GST fusion proteins, remaining in the non-eluted fraction (P) after thrombin cleavage.
-
Figure 3—source data 1
Western blot and corresponding SDS-PAGE analysis of CpxII-interacting proteins in Figure 3A and B.
- https://cdn.elifesciences.org/articles/92438/elife-92438-fig3-data1-v1.jpg
-
Figure 3—source data 2
Western blot and corresponding SDS-PAGE analysis of CpxII-interacting proteins in Figure 3C and D.
- https://cdn.elifesciences.org/articles/92438/elife-92438-fig3-data2-v1.jpg
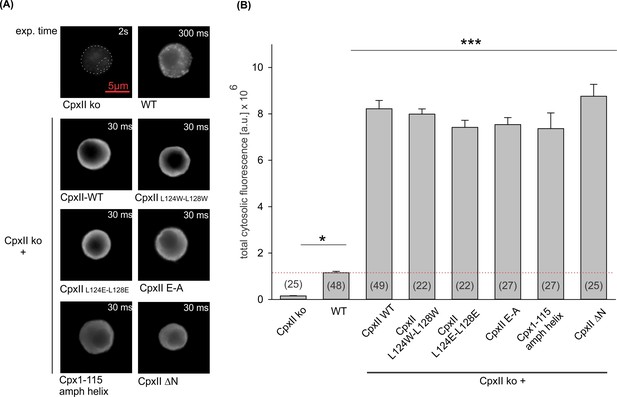
Expression analysis of CpxII and its mutants reveals similar levels of protein expression.
(A) Exemplary images of CpxII knockout (ko), wild-type (WT),or CpxII ko cells expressing CpxII WT or its mutant variants. Exposure times were adjusted to avoid the saturation of the sensor (CpxII ko 2 s, WT 300 ms, CpxII ko overexpressing CpxII or the CpxII mutants 30 ms). (B) Mean total fluorescence intensity (corrected for the exposure time) shows that CpxII WT and its mutants are expressed to similar levels in CpxII ko cells. Number of cells for each group is indicated in the corresponding bar. Kruskal-Wallis followed by Dunn’s post hoc test. ***p<0.001. Error bars indicate mean ± SEM.
-
Figure 3—figure supplement 1—source data 1
Excel file containing quantitative data of the expression analysis of CpxII and its mutant variants.
- https://cdn.elifesciences.org/articles/92438/elife-92438-fig3-figsupp1-data1-v1.xlsx
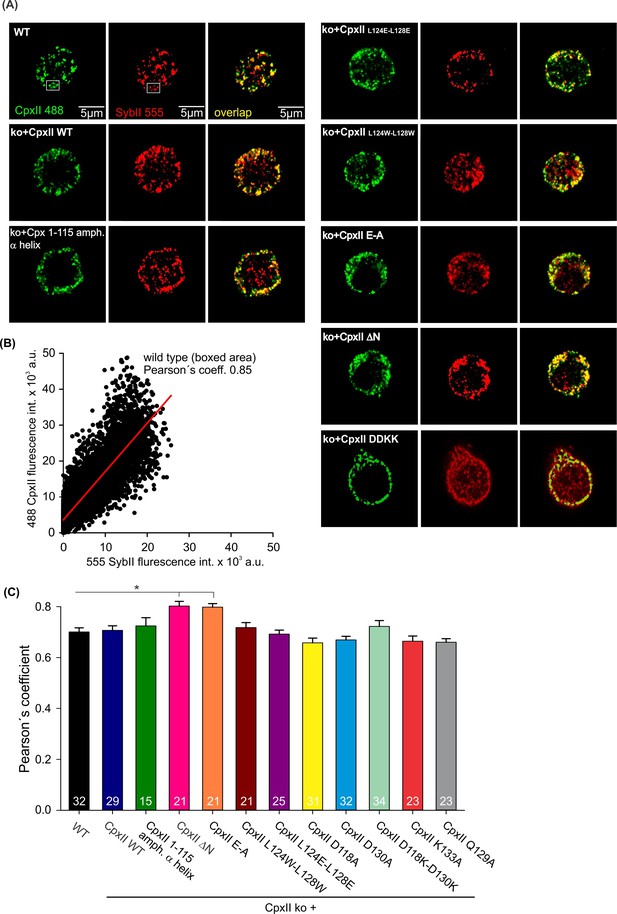
CpxII and its mutants concentrate at vesicular membranes.
(A) Exemplary immunostaining images for cells stained against CpxII or its mutant variants (green), and the vesicular marker SybII (red). The corresponding merged images (yellow) illustrate the overlap of CpxII and SybII signals (yellow). (B) Exemplary cytofluorogram for wild-type (WT) (boxed area) showing a strong correlation between SybII and CpxII stains (Pearson’s coeff.=0.85). (C) Pearson’s co-localization coefficient (threshold 6xSD of the background fluorescence) for CpxII or its mutants versus SybII shows that virally expressed CpxII variants concentrate at vesicles like the endogenous protein. Error bars indicate mean ± SEM. Kruskal-Wallis followed by Dunn’s post hoc test. *p<0.05.
-
Figure 3—figure supplement 2—source data 1
Excel file containing quantitative data of the co-localization analysis of CpxII and its mutant variants.
- https://cdn.elifesciences.org/articles/92438/elife-92438-fig3-figsupp2-data1-v1.xlsx
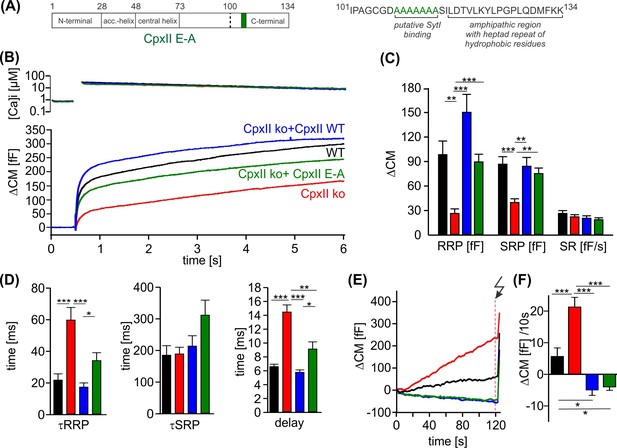
The cluster of glutamate residues in CpxII C-terminal domain (CTD) facilitates Ca2+-triggered exocytosis.
(A) Schematic representation of CpxII highlighting the position of the glutamate cluster within the CTD. Primary sequence of CpxII CTD showing the exchange of glutamate into alanine residues (CpxII E-A mutant). (B) Mean [Ca]i levels (upper panel) and capacitance measurement (CM) response from wild-type (WT) cells (black, n=18), CpxII knockout (ko) cells (red, n=19), and CpxII ko cells expressing either CpxII WT (blue, n=17) or the mutant CpxII E-A (green, n=24). (C) The mutant CpxII E-A only partially restores the fast component (readily releasable pool [RRP]) of synchronized exocytosis. (D) The CpxII E-A mutation significantly slows down the speed of the RRP and slowly releasable pool (SRP) and delays the stimulus-secretion coupling. (E) Average tonic exocytosis at similar submicromolar [Ca]i levels with the quantification in (F) showing that the mutant CpxII E-A effectively prevents premature vesicle loss at submicromolar [Ca]i levels like CpxII WT. ANOVA or Kruskal-Wallis followed by corresponding post hoc test. *p<0.05; **p<0.01; ***p<0.001. Error bars indicate mean ± SEM.
-
Figure 4—source data 1
Excel file containing quantitative data.
- https://cdn.elifesciences.org/articles/92438/elife-92438-fig4-data1-v1.xlsx
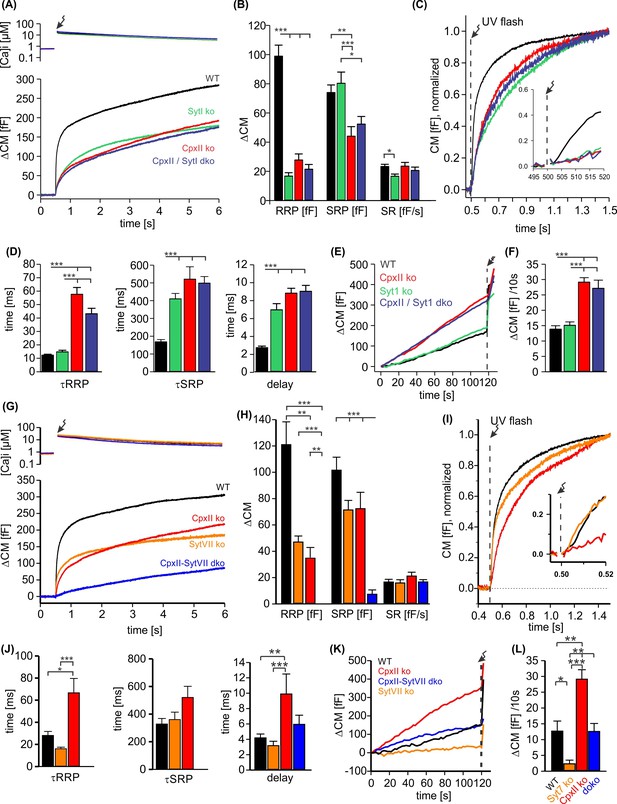
CpxII and SytI act interdependently in triggering synchronized exocytosis.
(A) Mean [Ca]i levels (upper panel) and capacitance measurement (CM) responses from wild-type (WT) cells (black, n=50), SytI knockout (ko) cells (green, n=46), CpxIIko (red, n=35), CpxII/SytI dko (dark blue, n=26). (B) Quantification of the readily releasable pool (RRP) and slowly releasable pool (SRP) size shows that SytI deletion in the absence of CpxII does not further aggravate the secretory deficit. (C) Normalized CM (as shown in A) scaled to the WT response 1 s after the flash. Inset, extended scaling of normalized CM during the first 20 ms after flash (arrow) depicting the delayed onset of secretion. (D) Kinetics of RRP and SRP exocytosis and the secretory delay. (E) Loss of SytI does not alter premature vesicle fusion. Additional loss of CpxII increases premature exocytosis to the level of CpxII ko (F). (G) Mean [Ca]i levels (upper panel) and CM responses from WT cells (black, n=17), SytVII ko cells (orange, n=20), CpxII ko (red, n=20), CpxII/SytVII dko (blue, n=24). (H) The RRP and SRP sizes are reduced by either CpxII or SytVII single ko, while the combined loss of CpxII and SytVII abolishes the EB. (I) Normalized CM (as shown in G) scaled to the WT response 1 s after the flash. Inset, extended scaling of normalized CM during the first 20 ms after flash (arrow). (J) Loss of CpxII but not of SytVII slows the time constants of RRP and SRP exocytosis (τRRP, τSRP) and prolongs the secretory delay. (K, L) SytVII deficiency diminishes the elevated premature exocytosis of CpxII ko cells (SytVII CpxII dko) and reduces it compared to WT cells. ANOVA or Kruskal-Wallis followed by corresponding post hoc test. *p<0.05; **p<0.01; ***p<0.001. Error bars indicate mean ± SEM.
-
Figure 5—source data 1
Excel file containing quantitative data.
- https://cdn.elifesciences.org/articles/92438/elife-92438-fig5-data1-v1.xlsx
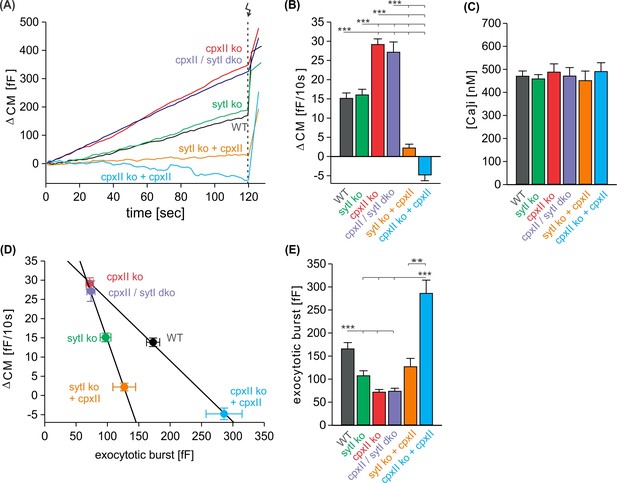
CpxII clamps premature exocytosis independently of SytI.
(A) Mean time course of premature exocytosis from CpxII knockout (ko) cells (red, n=35), CpxII/SytI dko (violet, n=26), SytI ko (light green, n=46), wild-type (WT) (black, n=49), SytIko+CpxII (dark green, n=22), CpxII ko+CpxII (blue, n=15). (B, C) Quantification of the premature exocytosis rate per 10 s at similar calcium levels shown in (C) for indicated groups. (D, E) The exocytotic burst (EB) size (readily releasable pool [RRP] + slowly releasable pool [SRP] component, E) correlates inversely with the extent of the preceding premature exocytosis upon changes of CpxII expression in the presence and in the absence of SytI (solid lines linear regressions, R2=0.99). ANOVA or Kruskal-Wallis followed by corresponding post hoc test. **p<0.01; ***p<0.001. Error bars indicate mean ± SEM.
-
Figure 5—figure supplement 1—source data 1
Excel file containing quantitative data.
- https://cdn.elifesciences.org/articles/92438/elife-92438-fig5-figsupp1-data1-v1.xlsx
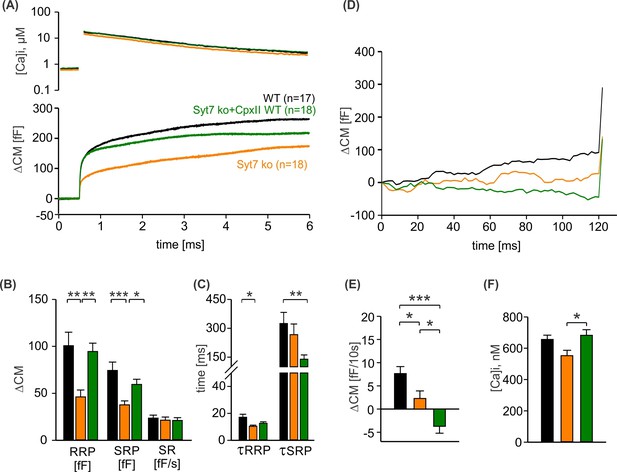
CpxII boosts exocytosis in the absence of SytVII.
(A) Mean [Ca]i levels (upper panel) and capacitance measurement (CM) responses from wild-type (WT) cells (black, n=17), SytVII knockout (ko) cells (orange, n=18), and SytVIIko cells expressing CpxII (green, n=18). (B) SytVII deletion reduces the readily releasable pool (RRP) and slowly releasable pool (SRP) size without affecting the sustained rate of secretion. (C) Kinetics of RRP and SRP exocytosis and the secretory delay. (D) Time course of premature exocytosis at submicromolar [Ca]i. (E, F) Loss of SytVII reduces premature vesicle fusion (E), which is further diminished upon CpxII expression at nearly comparable [Ca]i (F). ANOVA or Kruskal-Wallis followed by corresponding post hoc test. *p<0.05; **p<0.01; ***p<0.001. Error bars indicate mean ± SEM.
-
Figure 5—figure supplement 2—source data 1
Excel file containing quantitative data.
- https://cdn.elifesciences.org/articles/92438/elife-92438-fig5-figsupp2-data1-v1.xlsx
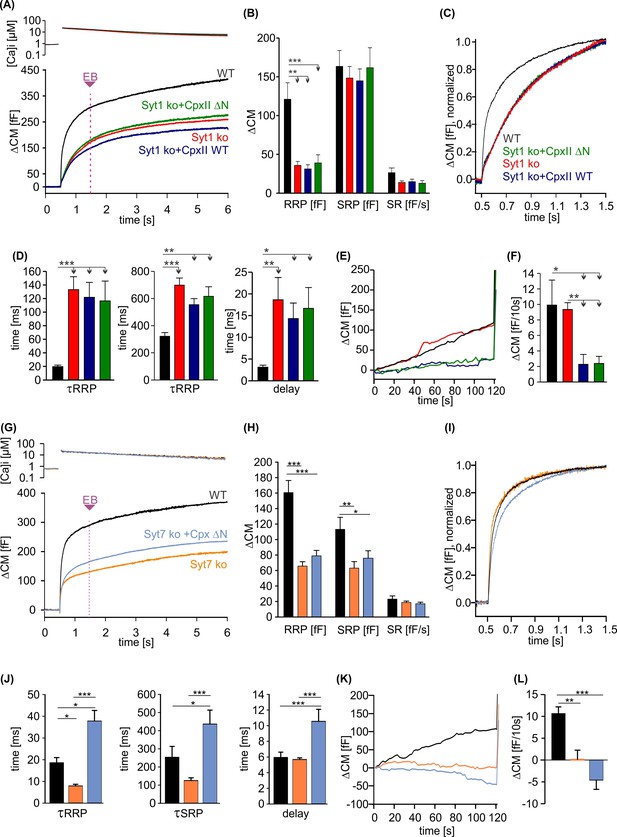
CpxII N-terminal domain (NTD) exclusively modulates the kinetics of SytI-mediated exocytosis.
(A) Mean [Ca]i levels (upper panel) and capacitance measurement (CM) response from wild-type (WT) cells (black, n=13), SytI knockout (ko) cells (red, n=19), and those overexpressing either CpxII WT (dark blue, n=16), or the mutant CpxII ΔN (green, n=20). In the absence of SytI, Cpx ΔN fails to slow down exocytosis timing as observed in SytVII cells (I). (B–D) Neither CpxII nor CpxII ΔN expression in SytI ko cells alters the size of the pools, the sustained rate (SR) rate (B) or the kinetics of synchronous exocytosis (C, normalized CM; D, time constants of readily releasable pool [RRP] and slowly releasable pool [SRP] exocytosis and secretory delay). (E, F) Both, CpxII and CpxII ΔN expression, hinders asynchronous release in the absence of SytI (F). (G) Mean [Ca]i levels (upper panel) and CM response of WT cells (black, n=13), SytVII ko cells (orange, n=17), and those expressing CpxII ΔN (light blue, n=29). (H) Both the RRP and SRP sizes are reduced in the absence of SytVII. (I) Normalized CM responses (of data shown in G) scaled to the WT response 1 s after the flash. Note that CpxII ΔN mutant slows kinetics of release in SytVII ko cells. (J) Loss of SytVII speeds up exocytosis timing, which in turn is slowed down by additional expression of CpxII ΔN. (K, L) Analysis of premature exocytosis showing reduced premature exocytosis in SytVII ko cells compared to WT. CpxII ΔN suppresses asynchronous release also in the absence of SytVII. ANOVA or Kruskal-Wallis followed by corresponding post hoc test. *p<0.05; **p<0.01; ***p<0.001. Error bars indicate mean ± SEM.
-
Figure 6—source data 1
Excel file containing quantitative data.
- https://cdn.elifesciences.org/articles/92438/elife-92438-fig6-data1-v1.xlsx
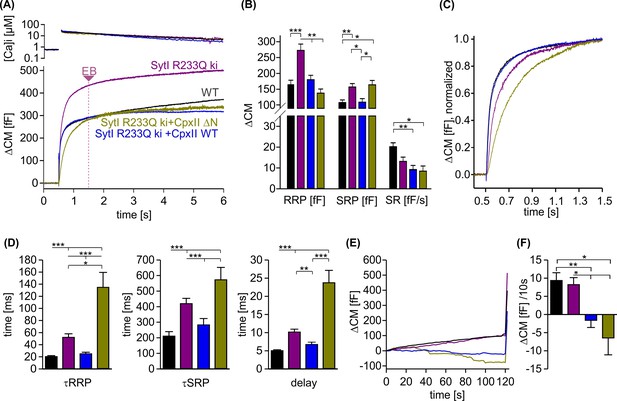
CpxII overexpression rescues the slow secretory rates of SytI R233Q knockin (ki) cells.
(A) Mean [Ca]i levels (upper panel) and capacitance measurement (CM) responses from wild-type (WT) (black, n=35), SytI R233Q ki (violet, n=39), and SytI R233Q ki cells expressing either CpxII WT (blue, n=18) or the mutant CpxII ΔN (olive green, n=11). (B) Compared to WT cells, SytI R233Q ki cells show an increased readily releasable pool (RRP) and slowly releasable pool (SRP) size, which are restored by CpxII or CpxII ΔN expression. (C) While CpxII speeds up exocytosis timing of SytI R233Q ki cells to the level of WT cells, CpxII ΔN slows it further down. Normalized CM responses (of data shown in A) scaled to the WT response 1 s after the flash. (D) CpxII and CpxII ΔN expression oppositely regulate the kinetics of exocytosis (τRRP, τSRP) and the secretory delay. (E, F) Mean premature exocytosis, determined at similar submicromolar [Ca]i (in nM, SytI R233Q ki:623±31; SytI R233Q ki+CpxII:569±28; SytI R233Q ki+CpxII ΔN:600±26; WT: 582±22), showing that CpxII WT or CpxII ΔN expression effectively hinders premature vesicle secretion. ANOVA or Kruskal-Wallis followed by corresponding post hoc test *p<0.05; **p<0.01; ***p<0.001. Error bars indicate mean ± SEM.
-
Figure 7—source data 1
Excel file containing quantitative data.
- https://cdn.elifesciences.org/articles/92438/elife-92438-fig7-data1-v1.xlsx
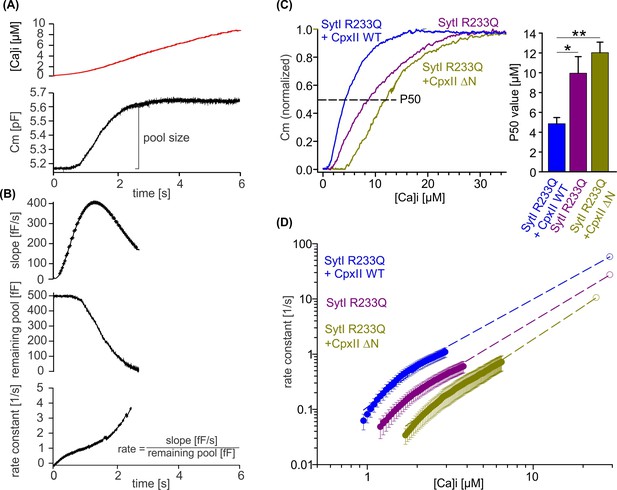
CpxII N-terminal domain (NTD) increases the Ca2+ affinity of secretion.
(A) Exemplary capacitance measurement of a chromaffin cell (lower panel) in response to a ramp-like increase in intracellular Ca2+ (top panel). (B) The slope of the ΔCM (top panel), remaining pool (middle panel), and rate of fusion (lower panel) were determined from the data shown under (A) with a temporal interval of 40 ms to match the timing of the ratiometric Ca2+ measurement. The rate was determined by dividing the slope [fF/s] by the remaining pool size [fF]. (C) Mean profile of pool depletion as the function of [Ca]i (left panel) for SytI R233Q knockin (ki) cells (violet, n=15) and those overexpressing CpxII wild-type (WT) (blue, n=14) or CpxII ΔN (olive green, n=11). Note that expression of CpxII WT lowers [Ca]i for half-maximal pool depletion (P50), whereas CpxII ΔN increases it (right panel). (D) Double-logarithmic plot of the mean exocytotic rate as the function of [Ca]i for the indicated groups. CpxII overexpression increases the rate of secretion from SytI R233Q ki cells, whereas loss of CpxII NTD further slows it down. Hill equations with similar coefficient, but different KD value, approximate the data (dashed lines). Extrapolation of the rates at low [Ca]i coincides with the readily releasable pool (RRP) kinetics (Figure 7D) determined in the flash experiment (hollow circle). ANOVA or Kruskal-Wallis followed by corresponding post hoc test. *p<0.05; ***p<0.001. Error bars indicate mean ± SEM.
-
Figure 8—source data 1
Excel file containing quantitative data.
- https://cdn.elifesciences.org/articles/92438/elife-92438-fig8-data1-v1.xlsx
Tables
Reagent type (species) or resource | Designation | Source or reference | Identifiers | Additional information |
---|---|---|---|---|
Strain, strain background (Mus musculus) | C57BL/6 | Jackson Lab strain: # 000664 | RRID:IMSR_JAX:000664 | |
Genetic reagent (Mus musculus) | Cplx 2 -/- | Reim et al., 2001 | PMID:11163241 | |
Genetic reagent (Mus musculus) | Syt 1-/- | Jackson Lab strain: # 002478 | PMID:7954835 | |
Genetic reagent (Mus musculus) | Syt 7-/- | Jackson Lab Strain: # 004950 | PMID:18308933 | |
Genetic reagent (Mus musculus) | Syt 1-/- /Syt 7-/- | This paper: Syt1: Jackson Lab Strain: # 002478 Syt7: Jackson Lab Strain: # 004950 | PMID:18308932 | |
Genetic reagent (Mus musculus) | Syt 1 R233Q ki | Fernández-Chacón et al., 2001; The Jackson laboratory B6;129P2-Syt1tm3Sud/J RRID:IMSR_JAX:006385 | PMID:11242035 | |
Antibody | Mouse monoclonal anti-Syntaxin1 | Synaptic Systems | Cat# 110 001 | Western blot: 1:1000 |
Antibody | Mouse monoclonal anti- SNAP25 | Synaptic Systems | Cat# 111 011 | Western blot: 1:1000 |
Antibody | Mouse monoclonal anti- SynaptobrevinII | Synaptic Systems | Cat# 104 211 | ICC, western blot: 1:1000 |
Antibody | Mouse monoclonal anti-Synaptotagmin I | Synaptic Systems | Cat# 105 011 | Western blot: 1:1000 |
Antibody | Mouse monoclonal anti-Synapsin 1 | Synaptic Systems | Cat# 106 001 | Western blot: 1:1000 |
Antibody | Rabbit polyclonal anti-CpxII | This paper | Materials and methods | ICC, western blot 1:5000 |
Antibody | HRP conjugated goat-anti mouse | Bio-Rad Laboratories | Cat# 170-5047 | Western blot: 1:1000 |
Antibody | HRP conjugated goat-anti rabbit | Bio-Rad Laboratories | Cat# 170-5046 | Western blot: 1:1000 |
Antibody | Alexa Fluor 555 goat anti-mouse | Invitrogen | Cat# A21422 | ICC: 1:1000 |
Antibody | Alexa Fluor 488 goat anti-rabbit | Invitrogen | Cat# A11008 | ICC: 1:1000 |
Recombinant DNA reagent (Mus musculus) | Cplx2-WT | GenBank: U35101.1 | ||
Transfected construct (Mus musculus) | pSFV-CpxII 27-134 (CpxII ΔN) IRES-EGFP | This paper | Derived from U35101.1 with indicated mutations, Semliki Forest virus expression construct, see Mutagenesis and viral constructs | |
Transfected construct (Mus musculus) | pSFV-CpxII 1-115- IRES-EGFP | This paper | Derived from U35101.1 with indicated mutations, Semliki Forest virus expression construct, see Mutagenesis and viral constructs | |
Transfected construct (Mus musculus) | pSFV-CpxII 1-115-amphepathic helix IRES-EGFP | This paper | Derived from U35101.1 with indicated mutations, Semliki Forest virus expression construct, see Mutagenesis and viral constructs | |
Transfected construct (Mus musculus) | pSFV-CpxII L124W-L128W IRES-EGFP | This paper | Derived from U35101.1 with indicated mutations, Semliki Forest virus expression construct, see Mutagenesis and viral constructs | |
Transfected construct (Mus musculus) | pSFV-CpxII L124E-L128E IRES-EGFP | This paper | Derived from U35101.1 with indicated mutations, Semliki Forest virus expression construct, see Mutagenesis and viral constructs | |
Transfected construct (Mus musculus) | pSFV-CpxII L117W-L121W IRES-EGFP | This paper | Derived from U35101.1 with indicated mutations, Semliki Forest virus expression construct, see Mutagenesis and viral constructs | |
Transfected construct (Mus musculus) | pSFV-CpxII L124E-L128E IRES-EGFP | This paper | Derived from U35101.1 with indicated mutations, Semliki Forest virus expression construct, see Mutagenesis and viral constructs | |
Transfected construct (Mus musculus) | pSFV-CpxII Q129A IRES-EGFP | This paper | Derived from U35101.1 with indicated mutations, Semliki Forest virus expression construct, see Mutagenesis and viral constructs | |
Transfected construct (Mus musculus) | pSFV-CpxII D118A IRES-EGFP | This paper | Derived from U35101.1 with indicated mutations, Semliki Forest virus expression construct, see Mutagenesis and viral constructs | |
Transfected construct (Mus musculus) | pSFV-CpxII D130A IRES-EGFP | This paper | Derived from U35101.1 with indicated mutations, Semliki Forest virus expression construct, see Mutagenesis and viral constructs | |
Transfected construct (Mus musculus) | pSFV-CpxII K133A IRES-EGFP | This paper | Derived from U35101.1 with indicated mutations, Semliki Forest virus expression construct, see Mutagenesis and viral constructs | |
Transfected construct (Mus musculus) | pSFV-CpxII D118K-D130K IRES-EGFP | This paper | Derived from U35101.1 with indicated mutations, Semliki Forest virus expression construct, see Mutagenesis and viral constructs | |
Transfected construct (Mus musculus) | pSFV-CpxII E-A IRES-EGFP | This paper | Derived from U35101.1 with indicated mutations, Semliki Forest virus expression construct, see Mutagenesis and viral constructs | |
Recombinant DNA reagent | pGEX-KG-vector | This paper | Prokaryotic expression vector, see Biochemistry | |
Peptide, recombinant protein | GST-C-terminal domain peptide | This paper | Materials and methods | |
Peptide, recombinant protein | GST-C-terminal domain peptide L124E-L128E | This paper | Materials and methods | |
Peptide, recombinant protein | GST-C-terminal domain peptide L124W-L128W | This paper | Materials and methods | |
Peptide, recombinant protein | GST-C-terminal domain peptide amphipathic helix | This paper | Materials and methods | |
Software algorithm | IgorPro | WaveMetrics Software | ||
Software algorithm | AutesP | NPI electronics | ||
Software algorithm | Zen2008 | Zeiss | ||
Software algorithm | ImageJ | National Institutes of Health |