Synaptic interactions between stellate cells and parvalbumin interneurons in layer 2 of the medial entorhinal cortex are organized at the scale of grid cell clusters
Figures
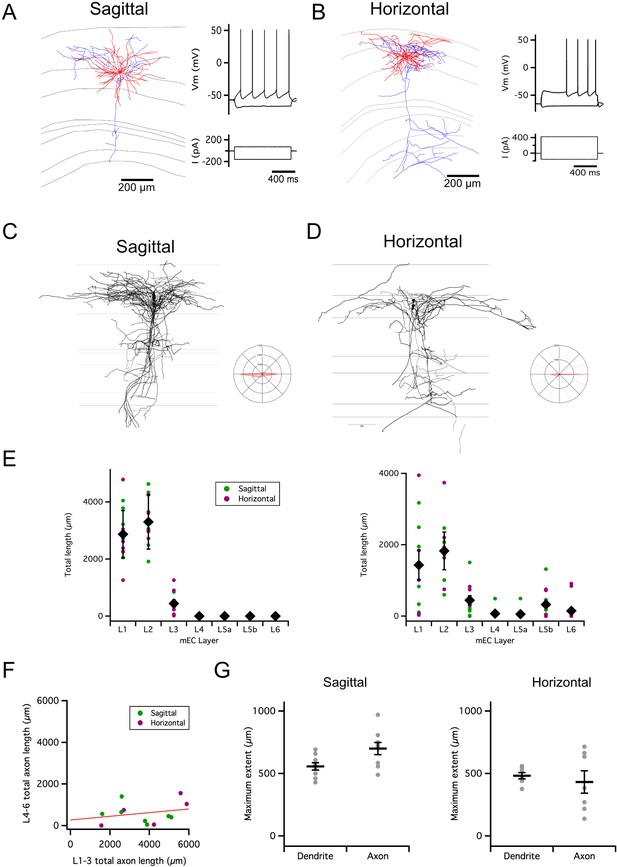
Organization of stellate cell axon collaterals.
(A–B) Examples of SCs reconstructed following patch-clamp recording from a sagittal (A) and horizontal (B) brain slice. Axons are in blue and dendrites in red. Traces to the right indicate membrane potential responses (upper) to current steps (lower) used to establish the electrophysiological profile of the recorded neurons. (C–D). Overlay of all SC axons reconstructed from sagittal (n=8) (C) and horizontal (n=5) (D) slices with soma position registered relative to the L1/2 border (left). Angle plots for all SC axons (see wedge plots in Figure 5 of Sürmeli et al., 2015) (right) indicate the dominance of axons that extend within layer 2. (E) Total length within each layer of dendrites (left) and axon collaterals (excluding the primary axon) (right). Colours distinguish data points from sagittal and horizontal slices, diamond symbols indicate the mean, and vertical bars indicate the standard error. The distribution of axons and dendrites depended on layer (p=1.72 x 10–11, F=12.8, df = 6), but did not differ between axons and dendrites (compartment: p=0.33, F=0.94, df = 1; layer x compartment: p=0.35, F=1.13, df = 6) and was independent of the slice orientation (plane: p=0.71, F=0.14, df = 1; plane x layer: p=0.62, F=0.74, df = 6; plane x layer x compartment: p=0.29, F=1.24, df = 6; two-way repeated measures ANOVA). (F) Total axon length in superficial (L1-3) layers did not predict length in deep (L4-6) layers (linear model: p=0.30, adjusted r2=0.014, F=1.17, df = 1, 11). (G) Maximum extent of L1-3 axons was greater than dendrites along the dorsoventral axis (sagittal sections; paired t-test: p=0.03, t=–2.7, df = 8), but similar along the mediolateral axis (horizontal sections; paired t-test: p=0.59, t=0.57, df = 6). Horizontal bars indicate the mean and vertical bars indicate the standard error.
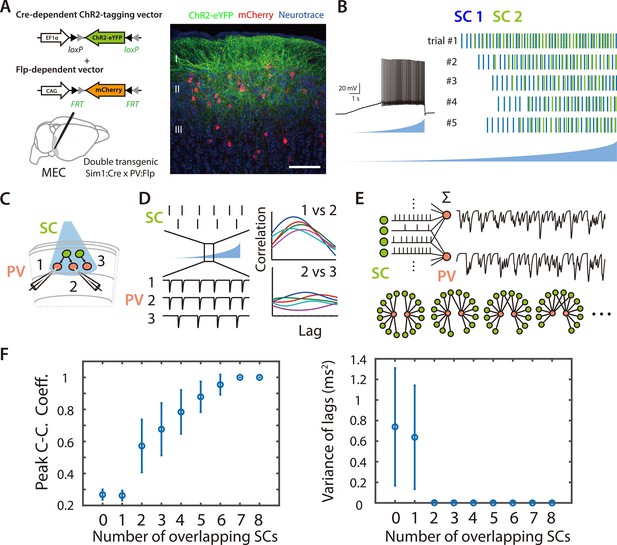
Asynchronous widefield activation strategy for investigating coordination between SCs and PV+ INs.
(A) Experimental strategy (left). To enable optical activation of SCs and targeted recording from PV interneurons, AAVs that express ChR2-eYFP conditionally on the presence of Cre and mCherry conditionally on the presence of Flp were injected into Sim1:Cre x PV:Flp double transgenic mice. Confocal imaging of fixed brain sections (right) reveals labeled SCs (green) and PV+ interneurons (red). Scale bar = 100 μm. (B) Example of spiking (left) and spike rasters (right) from two simultaneously recorded SCs (right panel) in response to ramp-modulated optical activation (blue). Note that spike firing by the two SCs is asynchronous. (C) Schematic of the simulated recording configuration used for evaluation of input from SCs to PV+ interneurons. (D) Schematic of spike rasters during ramp-modulated optical activation of SCs (upper left) and corresponding synaptic currents from the 3 PV interneurons in (lower left) for the circuit configuration in (C). Cross-correlation of EPSCs (right) recorded from pairs of PV cells receiving inputs from the same neuron (1 vs 2) are expect to show large and invariant peaks, whereas for PV+ interneurons receiving input from different SCs (2 vs 3) their peaks should be small and have variable latencies. (E) Schematic of the procedure for convolving EPSC waveforms with experimentally recorded spike times of ChR2-expressing SCs responding to the ramp-modulated light stimulus (upper). The proportion of overlapping SCs in each simulation is varied while the total SC input to each PV+ interneuron remains constant (lower). (F) Peak cross-correlation coefficient (left panel) and variance of the cross-correlation lag (right panel) as a function of number of overlapping SCs in each simulation. Mean and standard deviation for each group were calculated from 100 simulations.
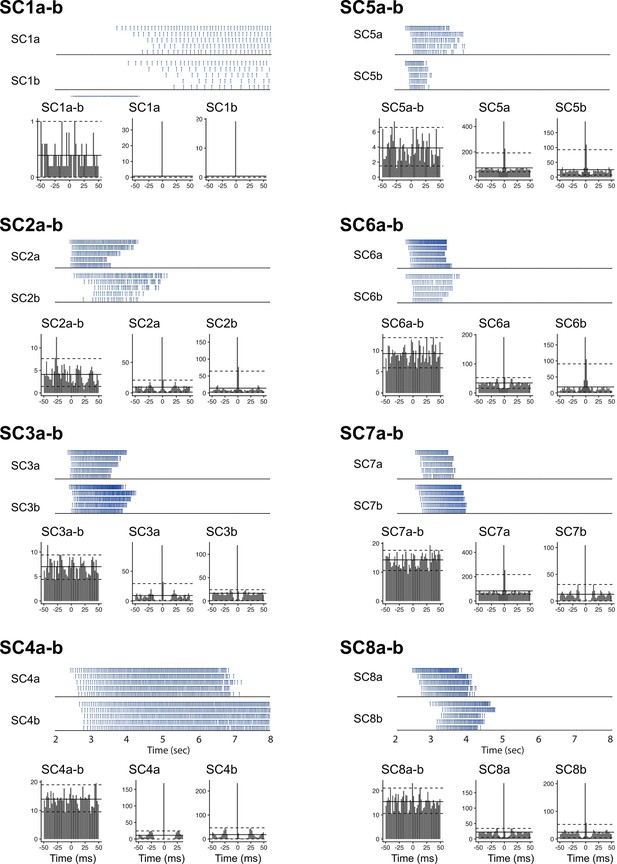
Optical ramp activation drives asynchronous activation of SCs.
Rasters of spike times (upper) from simultaneously recorded pairs of ChR2-expressing SCs in response to a ramp-modulated light stimulus, with corresponding cross-correlograms (lower left) and auto-correlograms (lower center and right). Pairs are numbered as SC1 to SC8, and neurons in each pair labeled as a and b. Spike times are used for simulations in Figure 2 and Figure 2—figure supplement 2. In the cross-correlograms and autocorrelograms, solid horizontal lines indicated the mean and dashed lines the 95% intervals of the data. To evaluate potential synchronicity we compare populations of cross-correlograms calculated from each set of sweeps, with cross-correlograms generated by shuffling the sweeps. Across all SC pairs there was no significant effect of time bin (for all pairs p>0.3, ANOVA), or interaction between time bin and the effect of shuffling the data (p>0.7, except for SC4a-b, where p=0.024). By contrast, similar analyses of autocorrelograms revealed highly significant effects of time bin (for all pairs p<1 x 10–3) and interactions between time bin and the effect of shuffling the data (p<1 x 10–12). The y-xis for all correlograms is the mean count.
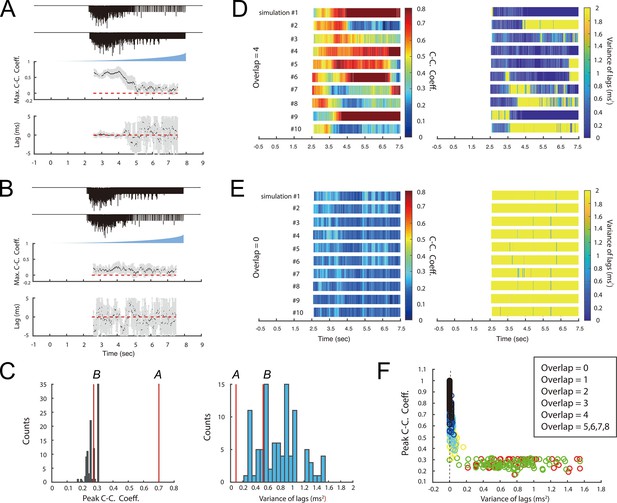
Simulation of SC inputs to PV+ interneurons.
(A, B) Simulation of EPSCs in PV+ interneurons (see Figure 2E) when 4 of 8 inputs from SCs to each PV+ interneuron also project to the other PV+ interneuron (A), and when all 8 inputs are independent (B). Simulated synaptic currents (upper) for each cell, maximum cross-correlation coefficients (middle) and cross-correlation lags (lower) as a function of time since light onset. The lower plots show means (black dots) and standard deviations (grey bars). The dashed red line corresponds to values of zero. (C) Peak correlation coefficients (left) and variance of their lags (right) from A and B (red lines) compared with null distributions generated by correlating data from pairs of basket cells recorded in different experiments. (D–E) Heat maps of mean across 10 simulations of the maximum cross-correlation coefficient within a sliding 250ms window (left panel) and the corresponding variance of lags (right panel) as a function of time since light onset for the simulated EPSCs in PV+ interneurons receiving presynaptic inputs consisting of 4 overlapping SCs (E) or no overlapping SCs (F). (F) Peak cross correlation coefficient plotted as a function of lag variance for simulations with varying degrees of overlap (colours) in the SC input to pairs of PV+ interneurons.
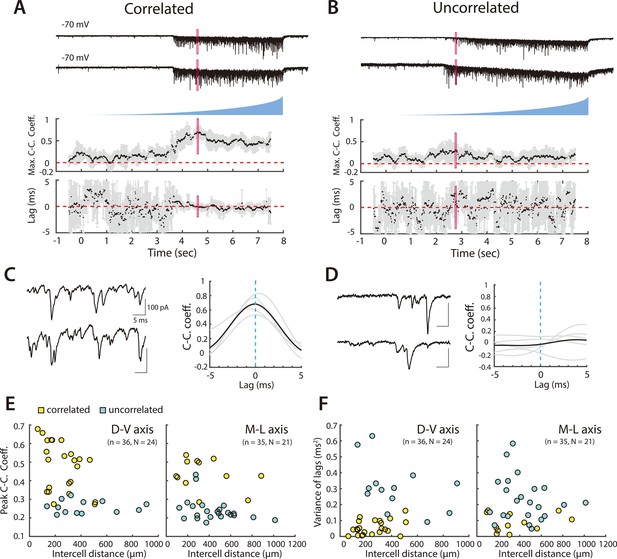
Organization of correlated input from SCs to PV+ interneurons.
(A, B) Examples of simultaneously recorded EPSCs (upper) from pairs of PV+ interneurons and corresponding measures of the maximal correlation coefficient and correlation lag variance in response to asynchronous activation of SCs. In (A) the correlation coefficient increases and the lag variance decreases with optical activation of SCs, indicating that the interneuron pair receives input from a shared SC population, whereas in (B) both parameters are independent of SC activation, indicating that each interneuron receives input from distinct SC populations. Maximum cross-correlation coefficients and cross-correlation lag variance are plotted as means for each window (black dots) and corresponding standard deviations (grey bars). Red rectangles indicate regions shown on an expanded timebase in (C, D). (C, D) Current traces (left) for the analysis window in which the mean peak cross-correlation coefficient was greatest (indicated by the red rectangles in panels A and B), and corresponding mean (bold) and individual (grey) cross-correlograms from five consecutive trials (right). (E, F) Peak cross-correlation coefficient (E) and variance of the corresponding lag (F) as a function of cell body separation along the dorsoventral axis (left panel) and mediolateral axis (right panel). Pairs indicated by yellow points were identified as receiving correlated inputs. Peak cross-correlation coefficients were correlated with intercell distance along the dorsoventral (adjusted R2=0.19, p=0.0046, F1,34 = 9.2) and mediolateral axes (adjusted R2=0.09, p=0.045, F1,33 = 4.3), whereas variance of the lags did not show a detectable dependence on distance (horizontal: adjusted R2=0.006, p=0.27, F1,34 = 1.2; vertical: adjusted R2=–0.03, p=1.0, F1,33 = 0.0).
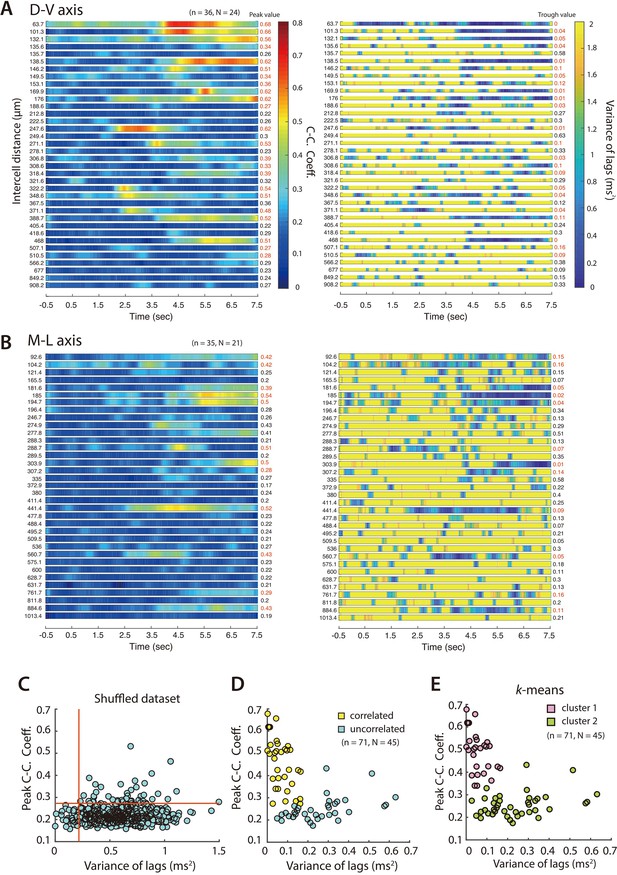
Distribution of PV+ INs receiving correlated input from SCs.
(A, B) Heat maps of mean maximum cross-correlation coefficient as a function of time since light onset for the simultaneously recorded PV+ cells (left) and averaged variance of lags as a function of time (right), for cells separated along the dorsal-ventral axis (A) and the medial-lateral axis (B). Plots are ordered by ascending intercell distances, which is indicated to the left of each heatmap. Peak values of the maximum cross-correlation coefficient and trough values of variance of lags are indicated to the right. Numbers in red indicate cell pairs assessed as receiving correlated input. (C) Peak cross-correlation coefficients as a function of variance of the corresponding lags for the shuffled dataset. (D) Peak cross-correlation coefficients as a function of variance of the corresponding lags for all the simultaneously recorded PV cells. Pairs of cells meeting the criteria for receiving coordinated input (peak cross-correlation coefficients >0.35 and trough values of variance of lags <0.2) are indicated in yellow. (E) The same data as (D) separated into two clusters using a k-means analysis.
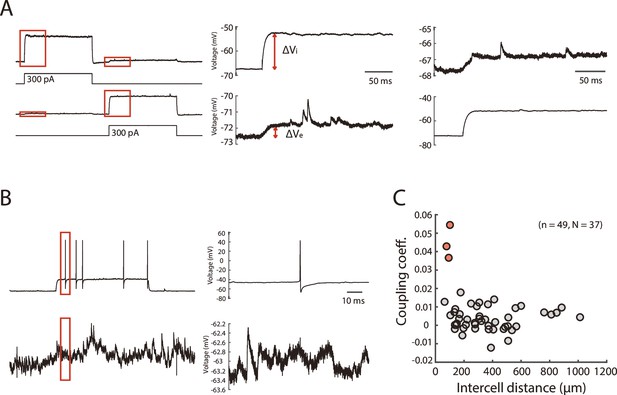
Testing for electrical and chemical synaptic connections between PV+ INs.
(A) Example of electrical connection between simultaneously recorded PV interneurons. Step current injection into either cell generates a large step change in membrane potential change in the injected cell and a smaller step change in membrane potential in the other cell. The coupling coefficient is determined by dividing ΔVe (the membrane potential change in the non-injected cell) by ΔVi (the membrane potential change in the injected cell) and averaging the values obtained from bidirectional testing. Traces to the right show regions in red boxes on the left on an expanded scale. (B) Example of testing chemical synaptic connections between simultaneously recorded PV interneurons. Action potentials were triggered in one neuron by injecting current steps and the membrane potential of the second was examined for the presence of synaptic potentials. The absence of detectable responses was unlikely to be a result of a small driving force for GABA-A responses as the postsynaptic membrane potential (mean –72.3±4.7 mV, range –82.7 to –57.25 mV) was in general well separated (mean difference –13.0±4.7 mV, range –23.4–2.0 mV) from the reversal potential for inhibitory currents (–59.3 mV). (C) Electrical coupling coefficients as a function of intercell distance for all the simultaneously recorded PV+ interneurons. Three pairs that have prominent electrical coupling (coupling coefficient >0.03) are indicated in red.
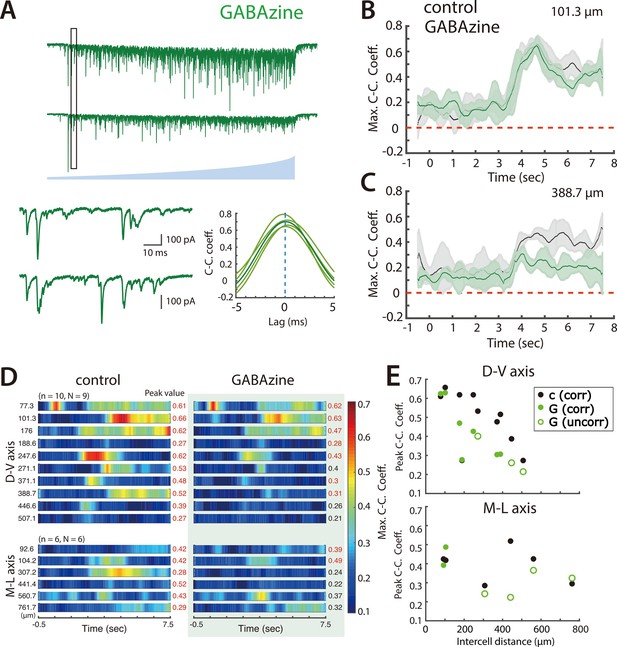
Synaptic inhibition differentially contributes to coordination by SCs of nearby and distant PV+ interneurons.
(A) Simultaneously voltage-clamp recording, in the presence of GABAzine to block fast inhibition, of the responses of a pair of PV+ interneurons (upper traces) to ramping light (blue) activation of ChR2 expressing SCs. The somata of the recorded neurons were approximately 101 µm apart. The black rectangles indicate the time frame of the expanded traces and corresponding cross-correlograms (lower). (B–C) Maximum cross-correlation coefficients as a function of time since light onset in control (grey) and GABAzine (green) conditions for the cell pair shown in panel A (B) and for a cell pair with cell bodies separated by approximately 389 μm (C). (D) Heat maps of mean maximum cross-correlation coefficient as a function of time since light onset for the simultaneously recorded PV+ interneurons in the control condition (left panel) and in the presence of GABAzine (right panel). Distances separating pairs of recorded cells are indicated to the left and peak values of the maximum cross-correlation coefficient to the right. (E) The changes in peak cross-correlation coefficient between control (c) and GABAzine (G) conditions plotted as a function of distance between cells in each pair (corr = classified as correlated input, uncorr = classified as uncorrelated input).
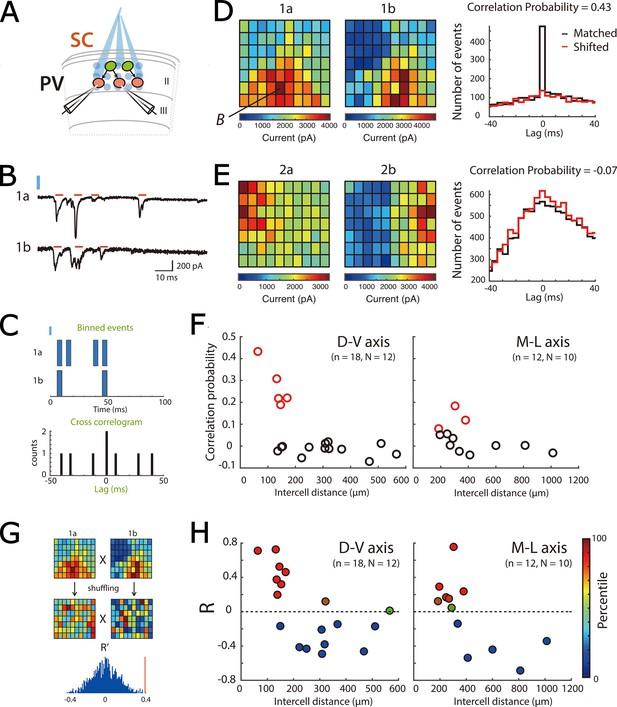
Focal activation of SCs confirms local direct connectivity to PV+ interneurons and reveals spatial organization.
(A) Schematic of experimental set up. Simultaneous whole-cell voltage-clamp recordings were made from pairs of PV+ inerneurons. ChR2-expressing SCs were activated by focal laser stimulation. (B) Examples of light-evoked current responses from a pair of simultaneously recorded PV cells. Red bars indicate detected events, blue bar indicate time of laser activation. (C) Schematic of analysis of spatial correlation of active input locations. (D–E) Heat maps (left) for each of two simultaneously recorded neurons (a and b) show total current amplitude at each stimulation location (x and y axes identify locations within the stimulation grid, example traces from the location marked B are shown in panel B). Histograms (right) show correlograms for matched (black) and shifted (red) events. A high correlation probability (D) is indicative of neurons receiving common inputs, whereas a low correlation probability (E) is indicative of neurons receiving distinct inputs. (F) Correlation probability as a function of intercell distance along the dorsal-ventral axis (left panel; adjusted R2=0.33, p=0.0072, F1,16 = 9.5) and the medial-lateral axis (right panel; adjusted R2=0.13, p=0.14, F1,10 = 2.6). Cell pairs with correlations unlikely to have arisen by chance are indicated in red. (G) Schematic of the strategy for comparing the spatial organization of inputs from SCs to pairs of PV+ INs. Pearson’s coefficient (R) was calculated following correlation of the heat maps for each cell, and for 100 pairs of shuffled heatmaps. In this example, the R from the original data (red vertical line) is above the 95th percentile of the shuffled data. (H) Pearson’s coefficient (R) following correlation of spatial maps as a function of intercell distance along the dorsal-ventral axis (left panel; adjusted R2=0.31, p=0.0092, F1,16 = 8.8) and the medial-lateral axis (right panel; adjusted R2=0.39, p=0.017, F1,10 = 8.16). Data are color-coded according to their percentile in the shuffled data.
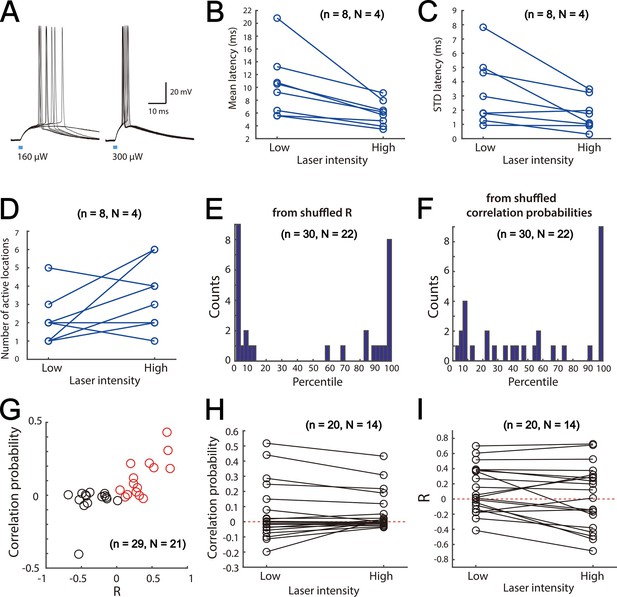
Focal activation of SCs.
(A) Examples of membrane potential responses of a ChR2-expressing SC to brief focal laser stimulation at low (left) and high (right) stimulation intensities. Each plot shows 5 overlaid responses. Note that at the higher stimulus intensity the spike latency is shorter and less variable. (B–C) Mean (B) and standard deviation (C) of the response latencies for all SCs tested at high and low stimulus intensities. (B) Mean latency low v.s. high: p=0.0078 (Signrank test, n=8 cells). (C) Std latency low v.s. high: p=0.0234 (Signrank test, n=8 cells). (D) Mean number of stimulus locations at which action potentials are triggered by high and low intensity stimulation. Number of active locations low v.s. high: p=0.1094 (Signrank test, n=8 cells) (E) Histogram of percentiles of the experimentally determined R compared with the shuffled data for all cell pairs (D-V and M-L axes). (F) Histogram of percentiles of the experimentally determined correlation probability compared with the shuffled data for all cell pairs (D-V and M-L axes). (G) Correlation probability as a function of R. Positively correlated cell pairs are indicated by red circles. (H) Comparison of correlation probability under low and high stimulating laser intensity. (I) Comparison of R under low and high stimulating laser intensity.
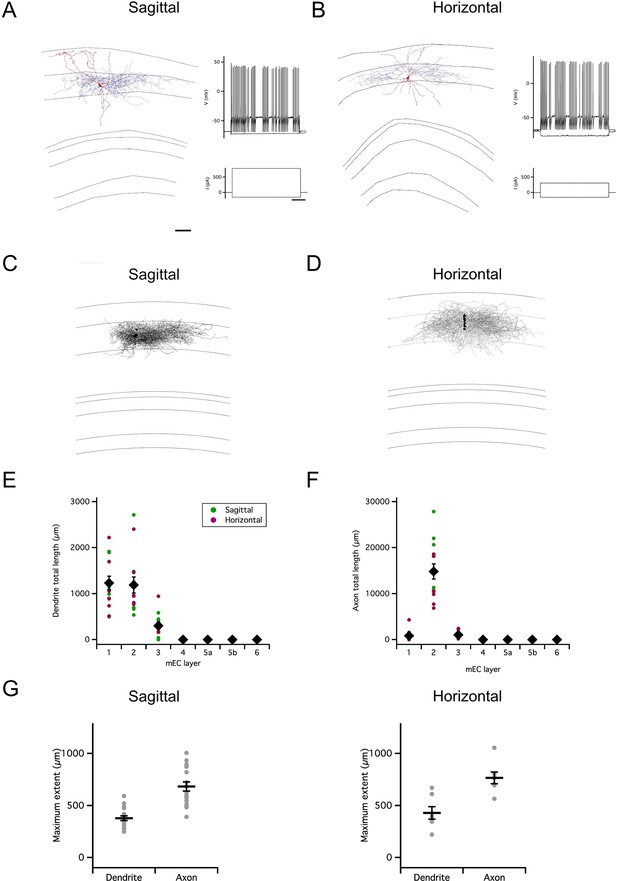
Organization of PV+ interneuron axons.
(A–B) Examples of reconstructed axonal (blue) and dendritic (red) morphology of PV+ interneurons in sagittal (A) and horizontal slices (B) (scale bar 200 µm). Axons are in blue and dendrites in red. Traces to the right indicate membrane potential responses (upper) to current steps (lower) used to establish the electrophysiological profile of the recorded neurons (scale bar 200ms). (C–D) Overlay of all PV interneurons reconstructed from sagittal (C) and horizontal (D) slices with soma position registered relative to the L1/2 border (left). Each reconstruction was aligned to the border between L1 and L2. (E–F) Total length of PV+ IN dendrites (E) and axons (F) in each layer of the MEC. Data is from the reconstructions in (C–D). Colours distinguish data points from sagittal and horizontal slices, diamond symbols indicate the mean, and vertical bars indicate the standard error. The distribution of lengths depended on layer (layer: p=<2 x 10–16, F=45, df = 6), differed between axons and dendrites (compartment: p=4.2 x 10–8; F=33.33, df = 1; compartment x layer:<2 x 10–16, F=37.21, d=6) and between slice orientations (plane: p=7.6 x 10–5, F=16.54, df = 1; plane x layer: p=2.7 x 10–12, F=13.54, df = 6; plane * layer * compartment: p=6.6 x 10–12, F=13.15, df = 6; repeated measures ANOVA). (G) Maximum extent of dendrites and axons along the dorsoventral (sagittal, n=17) and mediolateral (horizontal, n=7) axes of the MEC. The maximal axonal extent was greater than that for dendrites in the sagittal and the horizontal planes (sagittal: p=3.15 x 10–6, t=–7.0, df = 16; horizontal: p=0.00089, t - 6.1, df = 6; paired t-test). Horizontal bars indicate the mean and vertical bars indicate the standard error.
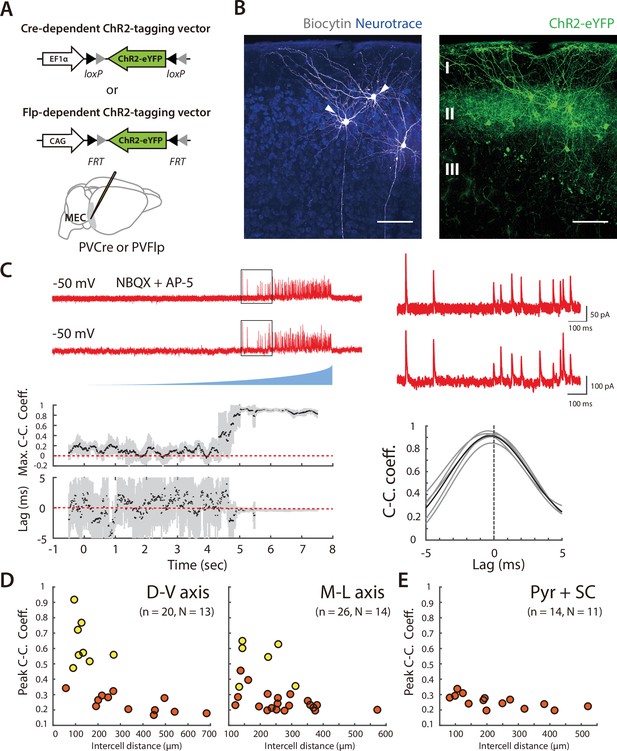
Organization of correlated inputs from PV+ interneurons to SCs.
(A) Targeting of ChR2 to PV+ INs used Cre- or FLP-dependent AAVs injected into the MEC of PVCre or PVFlp mice. (B) Whole-cell recordings were targeted to pairs of principal cells in layer 2 of the MEC (left, arrows) within the region of viral infection (right). Scale bar = 100 μm. (C) Example traces of simultaneously recorded IPSCs from the pair of SCs shown in (B) in response to ramp-modulated light stimulation. (D) Peak cross-correlation coefficient as a function of intercell distance along the dorsal-ventral axis (left panel; adjusted R2=0.41, p=0.0014, F1,18 = 14.3) and medial-lateral axis (right panel; adjusted R2=0.17, = 0.02, F1,24 = 6.0) for simultaneously recorded SC pairs. Pairs of cells meeting the criteria (peak cross-correlation coefficients >0.35 and trough values of variance of lags <0.2) are indicated in yellow, else in orange. (E) Peak cross-correlation coefficient as a function of intercell distance for all the simultaneously recorded SC and pyramidal cell pairs (D-V and M-L axes).
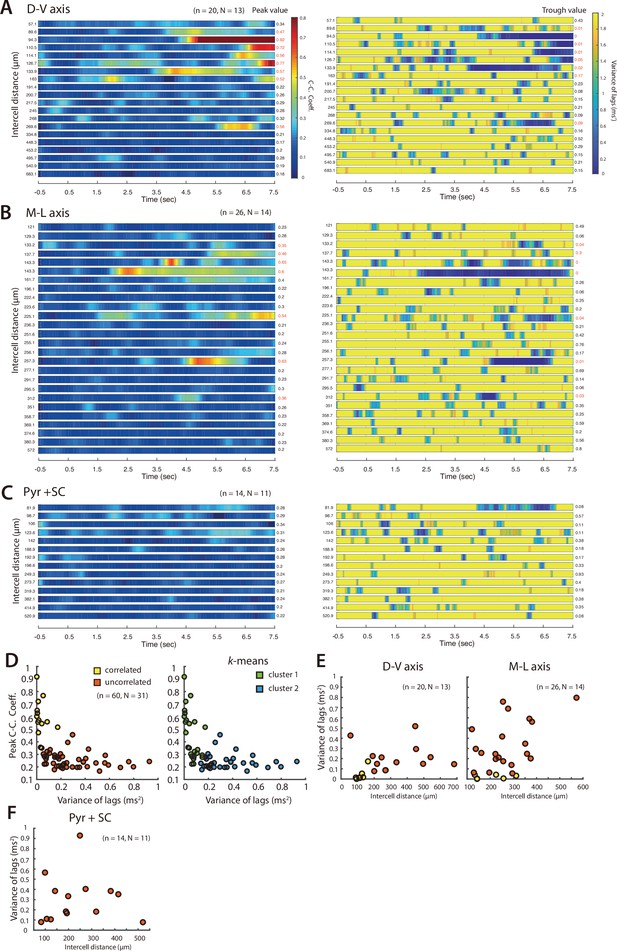
Distribution of SCs receiving correlated input from PV+ INs.
(A–C) Left panel: heat maps of averaged maximum cross-correlation coefficient as a function of time since light onset for the simultaneously recorded SCs along the dorsal-ventral axis (A), the medial-lateral axis (B) and for the simultaneously recorded SC and pyramidal cell pairs (C). Right panel: heat maps of averaged variance of lags as a function of time. Significant peak values of maximum cross-correlation coefficient and trough values of variance of lags are labeled in red. (D) Peak cross-correlation coefficients as a function of variance of lags for all the simultaneously recorded principal cells. Pairs of cells meeting the criteria (peak cross-correlation coefficients >0.35 and trough values of variance of lags <0.2) are indicated in yellow, other cells are in orange. (E) Variance of lags as a function of intercell distance of the simultaneously recorded SCs along the dorsal-ventral axis (left panel) and medial-lateral axis (right panel). (F) Variance of lags as a function of intercell distance of all the simultaneously recorded SC and pyramidal cell pairs (D-V and M-L axes).
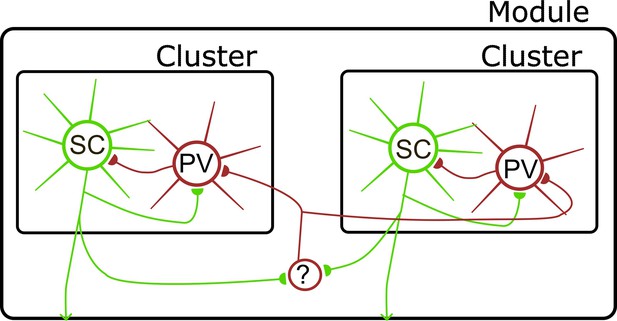
Model for organization of circuits in L2 of the MEC.
Direct interactions between SCs and PV+ INs define local clusters (see also Couey et al., 2013; Dhillon and Jones, 2000; Fuchs et al., 2016; Pastoll et al., 2012a; Winterer et al., 2017). Interactions between clusters are mediated via inhibitory interneurons (?), which could include somatostatin positive interneuron in layer 2 (Fernandez et al., 2022) or interneurons in layer 1 (Shi et al., 2023; Vandrey et al., 2022). Circles here represent populations of neurons, with connections between each population being incomplete rather than all to all.