The breath shape controls intonation of mouse vocalizations
Figures
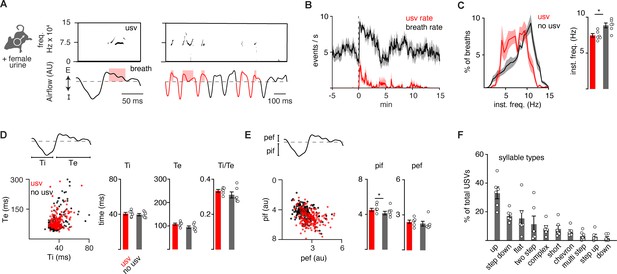
The full repertoire of vocalizations occurs within a normal appearing breath.
(A) Male mice exposed to female urine produced ultrasonic vocalizations (USVs) at about 75 kHz (top) that coincide with the expiratory airflow (E, arbitrary units) of the breath cycle (bottom). Red box indicates the length of the USV. A bout of vocalizations contains breaths with USVs (red) interspersed among sniff breaths (black). (B) Rates of breathing (black) and USV production (red). Exposure to female urine at time 0, n=6 mice. (C) Left, histogram of the instantaneous frequency of breaths with and without USVs from n=6 animals. Right, average instantaneous frequency for each mouse (mean ± SEM). Each dot is the mean from each animal. p-Value 0.03; paired t-test. (D) Scatter plot of the inspiratory (Ti) and expiratory time (Te) for USV (red) and non-USV (black) breaths from a representative animal. Right, bar graph of mean ± SEM of Ti, Te, and the ratio for n=6. p-Values 0.40, 0.18, and 0.25; paired t-test. (E) The breath peak inspiratory (pif) and expiratory (pef) airflow represented as in D. p-Values 0.01, 0.27; paired t-test. (F) Bar graph (mean ± SEM) of the percent of total USVs for each type from n=6 mice.
-
Figure 1—source data 1
Characterization of basal versus ultrasonic vocalization (USV) containing breaths.
- https://cdn.elifesciences.org/articles/93079/elife-93079-fig1-data1-v1.xlsx
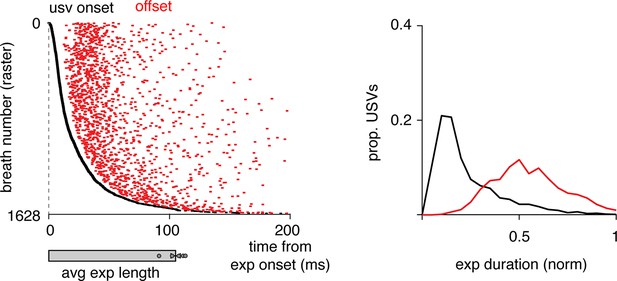
Ultrasonic vocalization (USV) onset and offset during expiration.
Left, raster plot of USV onset and offset times (ms) aligned to the beginning of expiration (onset, black and offset, red) for 1850 events. Below, the average expiratory length for n=6 animals. Right, histogram of the onset for each vocalization during a normalized expiratory duration. Note, while onset is biased to early expiration, vocalizations can begin throughout and even in late expiration.
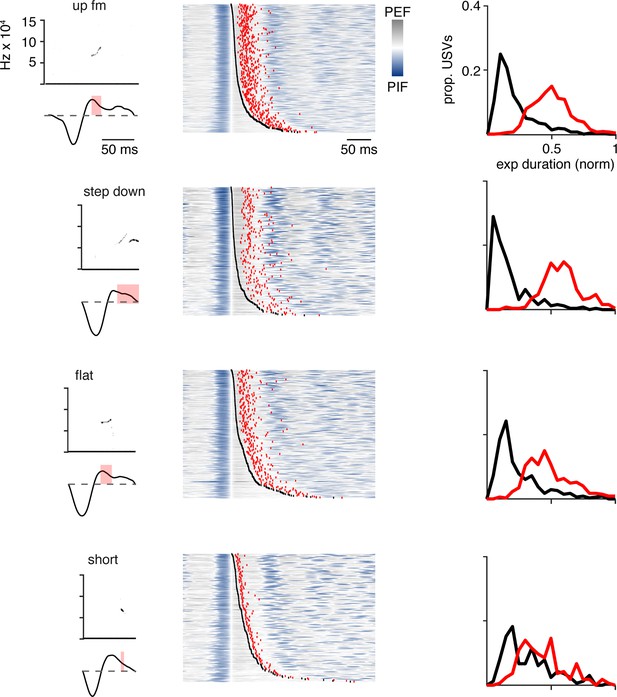
Representative example of the most common ultrasonic vocalization (USV) types and the onset and offset times during expiration.
Representative examples for each of the most common USV types and the representation of the onset and offset as Figure 1—figure supplement 1.
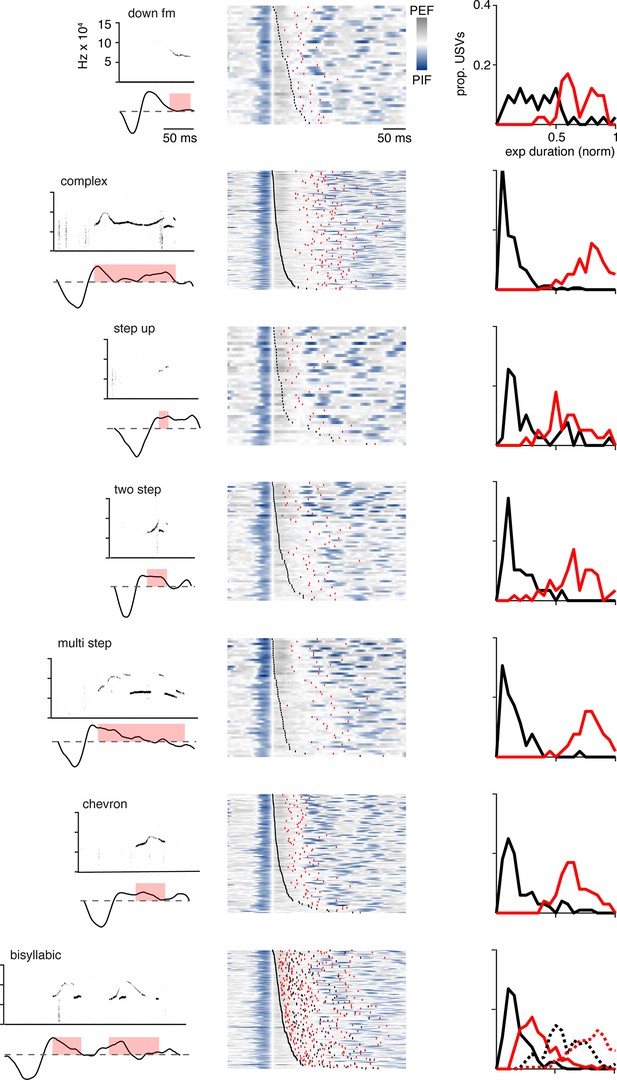
Representative example of the many ultrasonic vocalization (USV) types and the onset and offset times during expiration.
Representative examples for the remaining USV types and the representation of the onset and offset as Figure 1—figure supplement 1. Note, more complex vocalizations have onset and offset times that occur later in expiration.
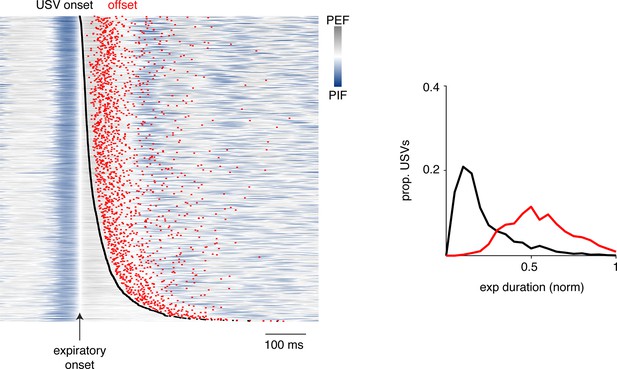
Raster plot of ultrasonic vocalization (USV) on- and offset plotted upon the breathing rhythm.
Raster plot of 1850 USVs aligned by the beginning of expiration with the sound onset and offset annotated by dots. The breath airflow is represented by the gradient from blue to gray, where inspiration is blue and expiration is gray. Note that breaths after ~1200 have late onset during expiration and delay the onset of the subsequent inspiration.
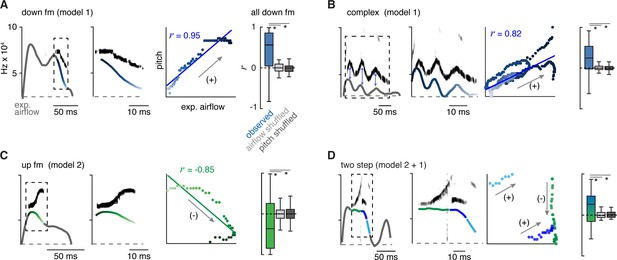
The 10 types of ultrasonic vocalizations (USVs) are produced by at least two mechanisms that modulate airflow.
(A) Left, example of the expiratory airflow and pitch for a down frequency modulated (fm) USV. Middle, magnification of airflow and sound. The scale of airflow is not displayed. The time of breath airflow from expiration onset during the USV is color coded blue to white. Scatter plot of instantaneous expiratory airflow and pitch for the single USV and the correlation (line, r). Note, the change in pitch mirrors airflow (annotated as ‘+’), consistent with the ‘breath modulation’ called model 1. Box and whisker plot of n=40 down fm correlation coefficients (r). Controls, normal USV pitch vs shuffled airflow or shuffled pitch to normal USV expiratory airflow. (B–D) Representative expiratory airflow and pitch, box and whisker plot of all r values, and onset/offset for complex (n=165), up fm (n=589), and two step (n=61) vocalizations represented as in A. The airflow for each unique USV element is uniquely color coded as green, blue, or purple. Note, the change in pitch for two components correlates and one anti-correlates. This is consistent with both mechanisms being sequentially used. Annotated as mixed blue and green box and whisker plot. *=p<0.05, one-way ANOVA with Sidak’s post hoc test.
-
Figure 2—source data 1
Observed and shuffled correlations between pitch and expiratory airflow.
- https://cdn.elifesciences.org/articles/93079/elife-93079-fig2-data1-v1.xlsx
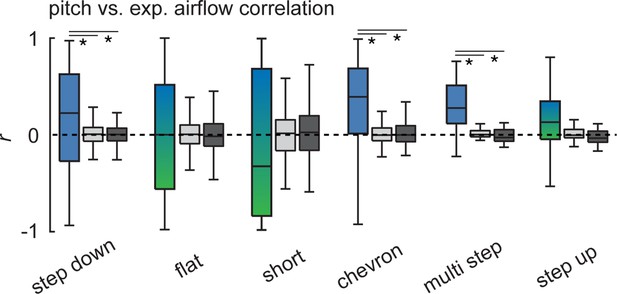
Correlation coefficient and onset/offset time for six ultrasonic vocalizations (USVs).
Box and whisker plot of correlation coefficients (r) for step down (n=293), flat (n=337), short (n=168), chevron (n=99), multi (n=58), and step up (n=40) USVs. *=p<0.05, one-way ANOVA with Sidak’s post hoc test.
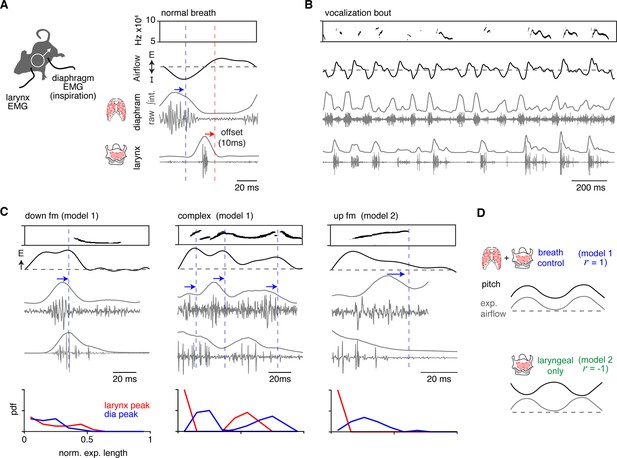
Ectopic activation of inspiratory and laryngeal muscles corresponds to changes in vocalization pitch.
(A) Activity of the diaphragm (inspiratory) and laryngeal (thyroarytenoid and cricothyroid) muscles were recorded in vivo by electromyography (EMG) simultaneously with breathing and ultrasonic vocalizations (USVs). Right, example of sound, breath airflow, and muscle activities during a basal breath. The diaphragm shows restricted activity during inspiration and laryngeal muscles are active during the post-inspiration period. Note, blue and red arrows/lines indicate the ~10 ms offset of the peak EMG activity and airflow/sound measurements. (B) Representative vocalization bout shows robust vocalizations and breathing in mice with implanted EMGs. (C) Representative sound, airflow, diaphragm, and laryngeal EMGs during the expiration of a down frequency modulated (fm) (n=23 annotated), complex (n=43 annotated), and up fm USV (n=29 annotated). Blue arrow and dashed line indicate the airflow and sound offset from the diaphragm peak muscle activity. Bottom, probability density function (PDF) for the peak of the integrated EMG activity for the diaphragm (blue) and larynx (red) during the normalized expiration. Y-axis is from 0 to 1. Note, data in A–D is from one animal with clear EMG signals that represents the findings in all three animals studied. (D) Schematics for each model. Model 1 – breath control: inspiratory and laryngeal muscles have alternating activity throughout the sound/expiration and a r>0 for pitch vs expiratory airflow. Muscle activities correspond to an increase (laryngeal) and a decrease (diaphragm) in pitch. Model 2 – laryngeal only: laryngeal but not diaphragm activity occurs during the sound and produces a r<0 for pitch vs expiratory airflow.
-
Figure 3—source data 1
Diaphragm and laryngeal electromyography (EMG) peaks normalized to expiratory length.
- https://cdn.elifesciences.org/articles/93079/elife-93079-fig3-data1-v1.xlsx
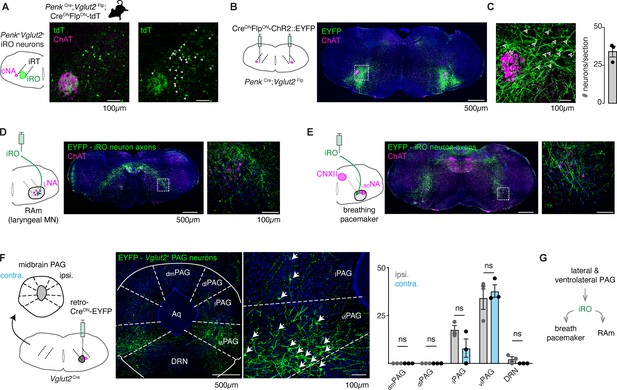
Anatomically and molecularly defined intermediate reticular oscillator (iRO) neurons form a brainstem phonatory circuit.
(A) Labeling of Penk+Slc17a6+ neurons in the iRO anatomical region in adult Penk-Cre;Slc17a6-Flp;Ai65 mice (CreONFlpON-tdTomato) (observed in n=5 mice). The iRO region is defined as medial to the compact nucleus ambiguus (cNA, ChAT+) in the ventral intermediate reticular formation (iRT). Note, the cNA is filled with tdTomato labeled axons. Cell bodies marked with arrowhead. (B) Bilateral stereotaxic injection of AAV CreONFlpON-ChR2::EYFP into the iRO anatomical region of Penk-Cre;Slc17a6-Flp adult mice. (C) Magnified boxed region in B. Arrowheads label neuron soma quantified right (n=3). (D) Axons of EYFP expressing iRO neurons from B in the retroambiguus (RAm) anatomical region where laryngeal premotor and motor neurons are located. (E) Axons of EYFP expressing iRO neurons from B in the breathing pacemaker. (F) Unilateral retrograde AAV CreON-EYP (AAVrg) stereotaxic injection into the iRO region in Slc17a6-Cre adults (n=3). Glutamatergic neurons were identified in the contralateral (contra.) and ipsilateral (ipsi.) midbrain periaqueductal gray (PAG). Anatomical regions of the PAG: dorsomedial (dm), dorsolateral (dl), lateral (l), ventrolateral (vl) nearby to the dorsal raphe nucleus (DRN) and surrounding the cerebral aqueduct (Aq). Quantification of glutamatergic PAG neurons in each region demarcated, ns = not statistically significant; two-way ANOVA with Sidka’s post hoc test. (G) Model schematic of the iRO as a central component of the brainstem phonation circuit to convert a vocalization ‘go’ cue from the PAG into a motor pattern.
-
Figure 4—source data 1
Quantification of periaqueductal gray (PAG) histology.
- https://cdn.elifesciences.org/articles/93079/elife-93079-fig4-data1-v1.xlsx
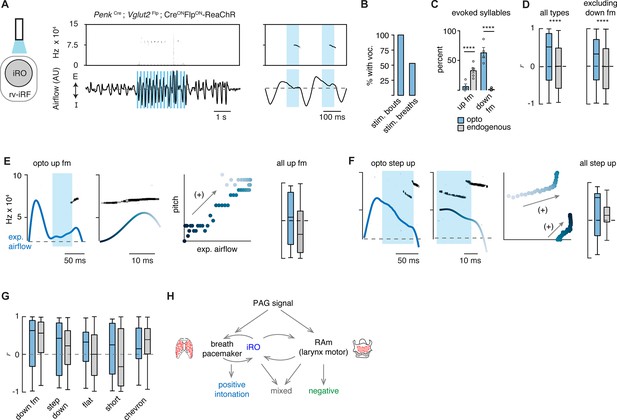
Ectopic activation of the intermediate reticular oscillator (iRO) evokes airflow correlated ultrasonic vocalization (USV) types and switches the relationship of the anti-correlated types.
(A) Optogenetic activation of the iRO region in Penk-Cre;Slc17a6-Flp;CreONFlpON-ReaChR mice evoked USVs (blue box, 5 Hz stimulation). USVs occurred during, or shortly after laser onset. (B) Percentage of stimulation bouts containing at least one USV and the percentage of breaths within the stimulation window containing a USV. (C) Percentage of optogenetically evoked (blue) or endogenously occurring (gray) syllables that are up frequency modulated (fm) of down fm. ****p-value<0.001; two-way ANOVA with Sidak’s post hoc test, p>0.05 for all other types. (D) Box and whisker plot of the correlation coefficient between breathing airflow and pitch (r) for all opto evoked (n=395) and endogenous (n=1850) USVs and all opto evoked and endogenous vocalizations without down fm (n=143 and 1810) from n=4 opto and n=6 endogenous mice. ****p-value<0.001; Mann-Whitney test. (E) Left, example of the expiratory airflow and pitch for an optogenetically evoked up fm USV. Middle, magnification of airflow and sound. Time of breath airflow during the USV is color coded blue to white. Scatter plot of instantaneous expiratory airflow and pitch for the single USV. Compare to endogenous up fm USV in Figure 2C. Right, box and whisker plot of correlation coefficients (r) for each optogenetically evoked and endogenous up fm USV (n=15 vs 589). (F) Step up USV as in E. Box and whisker plot of correlation coefficients (r) for each optogenetically evoked and endogenous step up USV (n=15 vs 40). (G) Box and whisker plots for the remaining r-values of optogenetically evoked USV types. Down fm n=242 vs 40, step down n=38 vs 293, flat n=34 vs 337, short n=27 vs 168, and chevron n=10 vs 99 from n=4 opto and 6 endogenous mice. (E–G) Two-way ANOVA with Sidak’s post hoc test for two-way comparisons was used; all p-values>0.05. (H) Schematic illustrating the two mechanisms to pattern the USV pitch. Left, the reciprocal connection between the iRO and breathing pacemaker patterns the USVs with a positive correlation between pitch and airflow. Right, the retroambiguus (RAm) control of the larynx dictates the anti-correlated USV types. Middle, the combination of these two mechanisms within a single breath create additional USV patterns.
-
Figure 5—source data 1
Comparison between ultrasonic vocalization (USV) type and intonation correlations for optogenetically evoked and endogenous USVs.
- https://cdn.elifesciences.org/articles/93079/elife-93079-fig5-data1-v1.xlsx
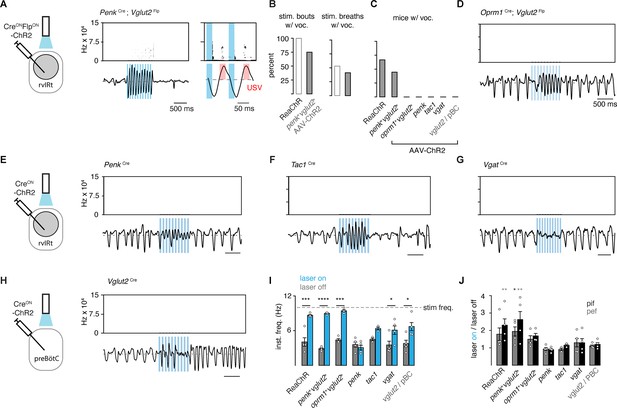
Optogenetic modulation of breathing and ultrasonic vocalizations (USVs) for different molecularly defined cell types in the intermediate reticular oscillator (iRO) anatomical region.
(A) Representative example of the change in breathing and USVs during a single light stimulation bout (blue box, 10 Hz) in Penk-Cre;Slc17a6-Flp mice stereotaxically injected with AAV CreONFlpON-Channel Rhodopsin2::YFP (ChR2) in the iRO (gray circle). Breathing rate is entrained by light and the amplitude is increased. USVs occur at the peak of expiration. rvIRt, rostral ventral intermediate reticular formation. (B) Percent of stimulation bouts and breaths within each bout that contain USVs or broad-band vocalizations in Penk-Cre;Slc17a6-Flp;ReaChR and Penk-Cre;Slc17a6-Flp virally injected mice. (C) Percent of mice with vocalizations for each tested genotype and injection site. ReaChR with iRO optic fiber implantation, n=6. iRO stereotaxic viral injection: Penk-Cre;Slc17a6-Flp, n=9; Oprm1-Cre;Slc17a6-Flp, n=4; Penk-Cre, n=4; Tac1-Cre, n=5, Slc32a1-Cre, n=4. PreBötzinger complex (PreBötC) stereotaxic viral injection: Slc17a6-Cre, n=4. (D–H) Representative examples of stimulation bouts for each genotype with rvIRt or preBötC viral injection. (I) Bar graph of average ± standard deviation and average for each animal (circle) for the instantaneous breathing frequency before and during the optogenetic laser pulse (10 Hz). *p<0.05; ***p<0.001; ****p<0.0001; two-way ANOVA with Sidak’s post hoc test. Genotypes and injection sites as in C–H. (J) Bar graph of average ± standard deviation and average for each animal (circle) for the ratio of the peak inspiratory flow (pif, black) and peak expiratory flow (pef, gray) for optogenetically stimulated breaths versus nearby unstimulated breaths for each genotype. *p<0.05; **p<0.01; two-way ANOVA with Sidak’s post hoc test. Genotypes and injection sites as in C–H.
Tables
Reagent type (species) or resource | Designation | Source or reference | Identifiers | Additional information |
---|---|---|---|---|
Genetic reagent (Mus musculus) | Slc17a6-IRES2-FlpO-D knock-in | The Jackson Laboratory | 030212 | |
Genetic reagent (M. musculus) | Penk-IRES2-Cre | The Jackson Laboratory | 025112 | |
Genetic reagent (M. musculus) | R26 LSL FSF ReaChR-mCitrine | The Jackson Laboratory | 024846 | |
Genetic reagent (M. musculus) | Tac1-IRES2-Cre-D | The Jackson Laboratory | 021877 | |
Genetic reagent (M. musculus) | Oprm1Cre:GFP KIKO | The Jackson Laboratory | 035574 | |
Genetic reagent (M. musculus) | Vgat-ires-cre knock-in | The Jackson Laboratory | 028862 | |
Genetic reagent (M. musculus) | Ai65(RCFL-tdT)-D | The Jackson Laboratory | 021875 | |
Strain, strain background (adeno-associated virus) | AAV5-hSyn-Con/Fon-hChR2(H134R)-EYFP | Addgene, Fenno et al., 2014. | 55645-AAV5 | |
Strain, strain background (adeno-associated virus) | AAV5-EF1a-DIO-hChR2(H134R)-EYFP-WPRE-HGHpA | Addgene | 20298-AAV5 | |
Strain, strain background (adeno-associated virus) | AAVrg-EF1a-DIO-hChR2(H134R)-EYFP-WPRE-HGHpA | Addgene | 20298-AAVrg | |
Antibody | Anti-GFP (chicken polyclonal) | Aves | GFP-1020 | (1:1000) |
Antibody | Anti-ChAT (goat polyclonal) | Millipore | AB144p | (1:500) |
Antibody | Anti-Chicken Alexa Fluor 488 (donkey polyclonal) | Invitrogen | A78948 | (1:500) |
Antibody | Anti-Goat Alexa Fluor 546 (donkey polyclonal) | Invitrogen | A11056 | (1:500) |
Antibody | Anti-Goat Alexa Fluor 647 (donkey polyclonal) | Invitrogen | A21447 | (1:500) |
Software, algorithm | MATLAB | Mathworks | MATLAB 2022b | |
Software, algorithm | VocalMat | Fonseca et al., 2021; Fonseca and Santana, 2022 | https://github.com/ahof1704/VocalMat | |
Software, algorithm | USVseg | Tachibana et al., 2020; rtachi-lab, 2024 | https://github.com/rtachi-lab/usvseg | |
Software, algorithm | Bespoke code | This paper, Bachmutsky et al., 2020, Wei et al., 2022 | https://github.com/YackleLab/the-breath-shape-controls-intonation-of-mouse-vocalizations copy archived at Yackle, 2024 | |
Software, algorithm | Prism 9 | GraphPad |