UGGT1-mediated reglucosylation of N-glycan competes with ER-associated degradation of unstable and misfolded glycoproteins
Figures
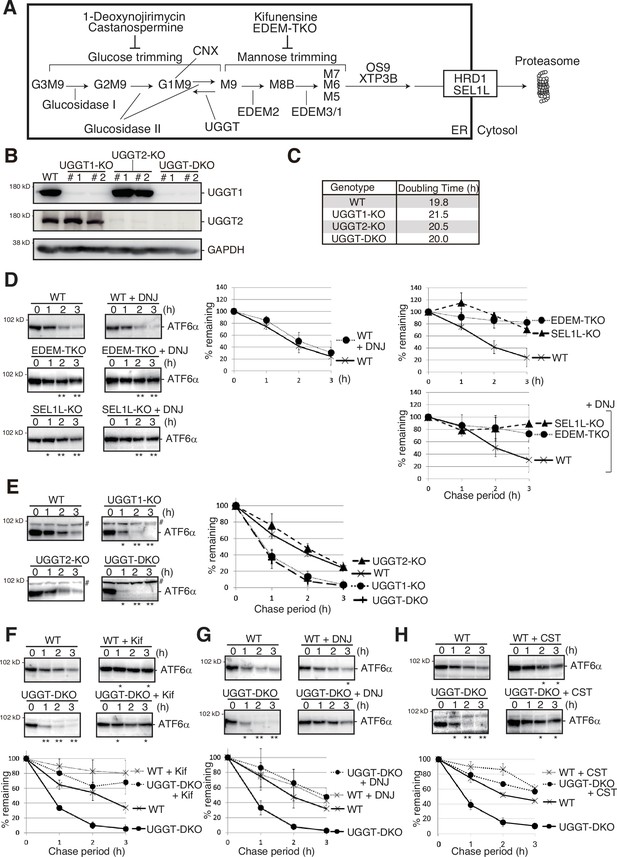
Effect of UGGT1/2-KO on degradation of ATF6α.
(A) Schematic presentation of N-glycan processing and glycoprotein ER-associated degradation (gpERAD). (B) Immunoblotting to determine endogenous protein expression of UGGT1 and UGGT2 in UGGT1-KO, UGGT2-KO, and UGGT-DKO HCT116 cells using anti-UGGT1, anti-UGGT2, and anti-Glyceraldehyde-3-phosphate dehydrogenase (GAPDH) antibodies. (C) Doubling time of wild-type (WT), UGGT1-KO, UGGT2-KO, and UGGT-DKO HCT116 cells (n=3). (D) Cycloheximide chase (50 μg/ml) and subsequent immunoblotting experiments to determine the degradation rate of endogenous ATF6α in WT, EDEM-TKO and SEL1L-KO HCT116 cells treated with or without 0.5 mM 1-deoxynojirimycin (DNJ) treatment. DNJ was added 2 hr before the addition of CHX. Endogenous ATF6α was detected by immunoblotting using anti-ATF6α antibody. The means from three independent experiments with standard deviations (error bars) are plotted against the chase period (n=3). p-value: *<0.05, **<0.01. (E) Cycloheximide chase (50 μg/ml) and subsequent immunoblotting experiments to determine the degradation rate of endogenous ATF6α in WT, UGGT1-KO, UGGT2-KO, and UGGT-DKO HCT116 cells (n=3), as in (D) # denotes a non-specific band. p-value: *<0.05, **<0.01. (F) Cycloheximide chase (50 μg/ml) and subsequent immunoblotting experiments to determine the degradation rate of endogenous ATF6α in WT and UGGT-DKO HCT116 cells treated with or without 10 μg/ml kifunensine (Kif) (n=3), as in (D). Kif was added 1 hr before the addition of CHX. p-value: *<0.05, **<0.01. (G) Cycloheximide chase (50 μg/ml) and subsequent immunoblotting experiments to determine the degradation rate of endogenous ATF6α in WT and UGGT-DKO HCT116 cells treated with or without 0.5 mM DNJ (n=3), as in (D). DNJ was added 2 hr before the addition of cycloheximide (CHX). p-value: *<0.05, **<0.01. (H) Cycloheximide chase (50 μg/ml) and subsequent immunoblotting experiments to determine the degradation rate of endogenous ATF6α in WT and UGGT-DKO HCT116 cells treated with or without 1 mM castanospermine (CST) (n=3), as in (D). CST was added 2 hr before the addition of CHX. p-value: *<0.05, **<0.01.
-
Figure 1—source data 1
Original files for western blot analysis are displayed in Figure 1B and D–H.
- https://cdn.elifesciences.org/articles/93117/elife-93117-fig1-data1-v1.zip
-
Figure 1—source data 2
PDF file containing original membranes of western blots for Figure 1B and D–H.
- https://cdn.elifesciences.org/articles/93117/elife-93117-fig1-data2-v1.zip
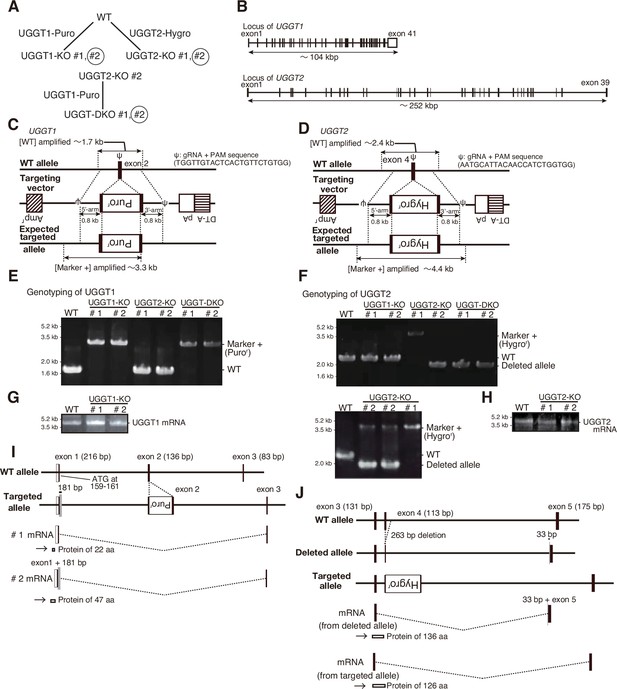
Generation of UGGT1/2-KO HCT116 cells.
(A) Strategy for obtaining UGGT1-KO, UGGT2-KO, and UGGT-DKO cells from WT HCT116 cells. UGGT1-KO #2, UGGT2-KO #2, and UGGT-DKO #2 were mainly used in this report. (B) Schematic presentation of the UGGT1 and UGGT2 loci. (C) Strategy of the CRISPR/Cas9-mediated targeting of exon 2 of the UGGT1 gene. (D) Strategy of the CRISPR/Cas9-mediated targeting of exon 4 of the UGGT2 gene. (E) Genomic PCR to confirm recombination of the UGGT1 locus. (F) Genomic PCR to confirm recombination of the UGGT2 locus. (G) RT-PCR to amplify cDNA corresponding to full-length UGGT1 mRNA from wild-type (WT) and two independent UGGT1-KO cell lines (#1 and #2). (H) RT-PCR to amplify cDNA corresponding to full-length UGGT2 mRNA from WT and two independent UGGT2-KO cell lines (#1 and #2). (I) Schematic presentation of UGGT1 mRNA expressed in two independent UGGT1-KO cell lines (#1 and #2) and their translational products, based on the determined sequence of the amplified cDNA in (G). (J) Schematic presentation of UGGT2 mRNA expressed from the deleted and targeted alleles in UGGT2-KO cell lines and their translational products, based on the determined sequence of the amplified cDNA in (H).
-
Figure 1—figure supplement 1—source data 1
Original files for agarose gel analysis are displayed in Figure 1—figure supplement 1E–H.
- https://cdn.elifesciences.org/articles/93117/elife-93117-fig1-figsupp1-data1-v1.zip
-
Figure 1—figure supplement 1—source data 2
PDF file containing original agarose gels for Figure 1—figure supplement 1E–H.
- https://cdn.elifesciences.org/articles/93117/elife-93117-fig1-figsupp1-data2-v1.pdf
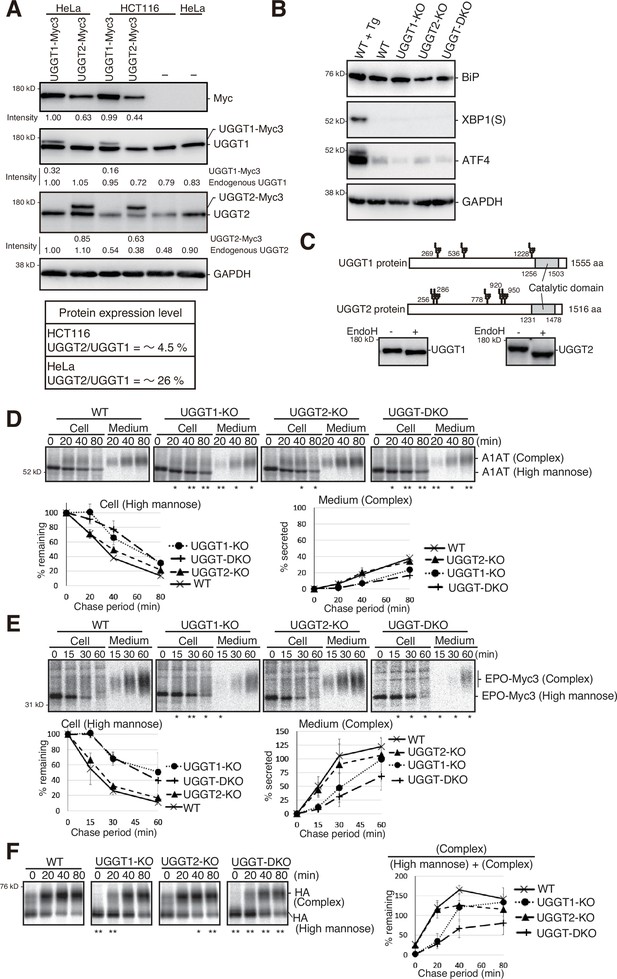
Characterization of UGGT1/2-KO HCT116 cells.
(A) Immunoblotting of cell lysates prepared from wild-type (WT) HCT116 and HeLa cells transiently expressing UGGT1-Myc3 or UGGT2-Myc3 by transfection using anti-Myc, anti-UGGT1, anti-UGGT2 and anti-GAPDH antibodies. The intensity of the lower band at the left in each panel is set to 1.00, and relative intensities are shown below. Anti-Myc antibody was used to estimate the ratio of the amounts of UGGT1-Myc3 and UGGT2-Myc3. Anti-UGGT1 antibody was used to estimate the amounts of endogenous UGGT1 and UGGT1-Myc3. Anti-UGGT2 antibody was used to estimate the amounts of endogenous UGGT2 and UGGT2-Myc3. These values were used to calculate the relative amounts of endogenous UGGT1 and UGGT2. Approximate expression level of UGGT2 relative to UGGT1 in HCT116 and HeLa cells is shown as % in the bottom panel. (B) Expression levels of BiP, XBP1(S), the spliced form of XBP1, ATF4, and GAPDH determined by immunoblotting of cell lysates, which were prepared from WT, WT treated with 300 nM Tg for 6 hr, UGGT1-KO, UGGT2-KO, and UGGT-DKO cells. (C) Schematic presentation of UGGT1 and UGGT2 proteins with potential N-glycosylation sites and catalytic domain indicated. Cell lysates were prepared from WT HCT116 cells, treated or not treated with endoglycosidase H (EndoH), and analyzed by immunoblotting using anti-UGGT1 and anti-UGGT2 antibodies. (D, E) Secretion of A1AT (D) and eythropoietin (EPO)-Myc3 (E) by WT, UGGT1-KO, UGGT2-KO, and UGGT-DKO HCT116 cells. Cells were transfected with plasmid to express A1AT or EPO-Myc3. Twenty-four hours later, cells were pulse-labeled with 35S-methionine and cysteine for 20 min and then chased for the indicated periods, followed by immunoprecipitation of A1AT or EPO-Myc3 from cells and medium using anti-A1AT or anti-Myc antibody, respectively, and subsequent subjection to reducing SDS-PAGE and autoradiography (n=3). The amount of A1AT or EPO-Myc3 in WT cells at 0 min was set as 100%. The means from three independent experiments with standard deviations (error bars) were plotted against the chase period. (F) Maturation of hemagglutinin (HA) in WT, UGGT1-KO, UGGT2-KO, and UGGT-DKO HCT116 cells. Pulse-chase experiments were conducted using various cells transfected with plasmid to express hemagglutinin (HA), followed by immunoprecipitation of HA from cells using anti-HA antibody (n=3), as in (D). The immunoprecipitates were digested with EndoH prior to subjection to reducing SDS-PAGE. The amount of HA with high-mannose type N-glycan at time 0 hr in WT cells was set as 100%.
-
Figure 1—figure supplement 2—source data 1
Original files for western blot and pulse-chase analysis are displayed in Figure 1—figure supplement 2A–F.
- https://cdn.elifesciences.org/articles/93117/elife-93117-fig1-figsupp2-data1-v1.zip
-
Figure 1—figure supplement 2—source data 2
PDF file containing original membranes of western blots and gels of pulse-chase analysis for Figure 1—figure supplement 2A–F.
- https://cdn.elifesciences.org/articles/93117/elife-93117-fig1-figsupp2-data2-v1.zip
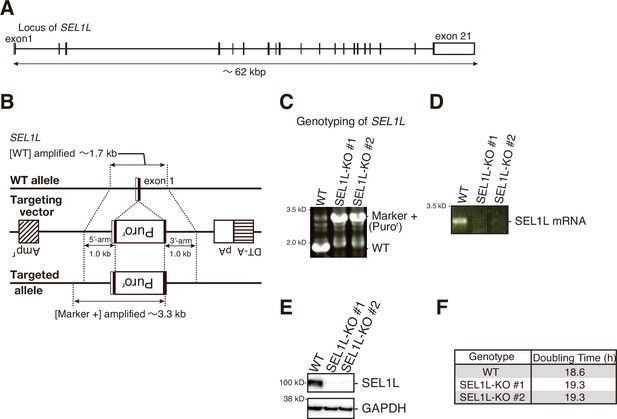
Generation of SEL1L-KO HCT116 cells.
(A) Schematic presentation of the SEL1L locus. (B) Strategy of the Transcription activator-like effector nuclease (TALEN)-mediated targeting of exon 1 of the SEL1L gene. (C) Genomic PCR to confirm recombination of the SEL1L locus. (D) RT-PCR to amplify cDNA corresponding to full-length SEL1L mRNA from wild-type (WT) and two independent SEL1L-KO cell lines (#1 and #2). (E) Immunoblotting of SEL1L protein in WT and two independent SEL1L-KO cell lines (#1 and #2) using anti-SEL1L antibody. (F) Doubling time of WT and two independent SEL1L-KO cell lines (#1 and #2).
-
Figure 1—figure supplement 3—source data 1
Original files for agarose gel and western blot are displayed in Figure 1—figure supplement 3C–E.
- https://cdn.elifesciences.org/articles/93117/elife-93117-fig1-figsupp3-data1-v1.zip
-
Figure 1—figure supplement 3—source data 2
PDF file containing original agarose gel and membranes of western blot for Figure 1—figure supplement 3C–E.
- https://cdn.elifesciences.org/articles/93117/elife-93117-fig1-figsupp3-data2-v1.pdf
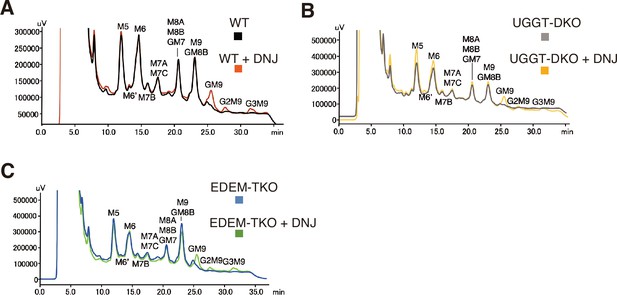
HPLC analysis of N-glycan of various types of cells untreated or treated with DNJ.
(A–C) Elution profiles of N-glycans from amide column prepared from total cellular glycoproteins of wild-type (WT) (A), UGGT1/2-double knockout (DKO) (B), and EDEM-triple knockout (TKO) (C) cells treated with or without 0.5 mM DNJ for 16 hr. Each peak was further analyzed by the ODS column to determine the exact amount of each N-glycan composition.
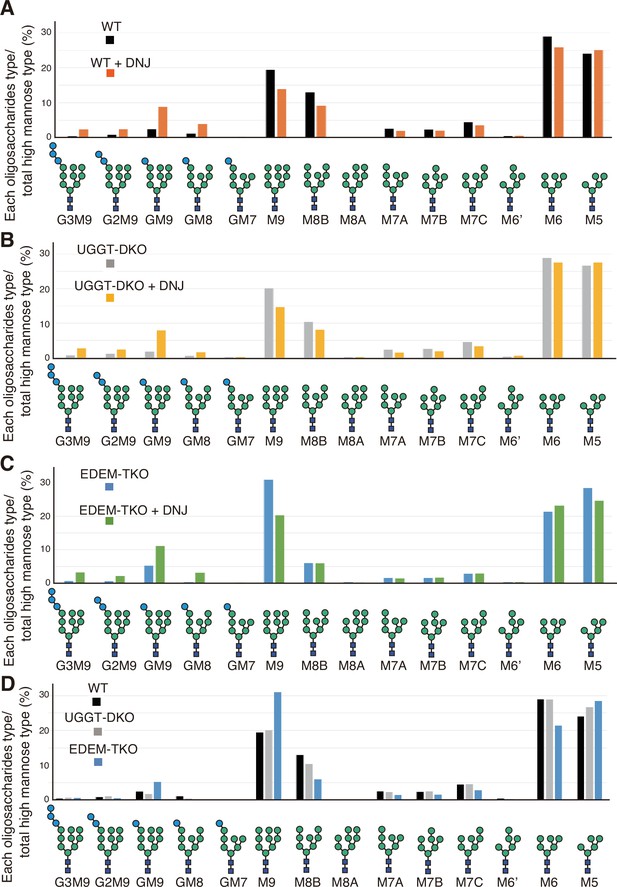
N-glycan profiling of various types of cells untreated or treated with 1-deoxynojirimycin (DNJ).
(A–D) Isomer composition of oligomannose-type N-glycans prepared from total cellular glycoproteins of wild-type (WT) cells (A), UGGT-DKO cells (B) and EDEM-TKO cells (C) treated with or without 0.5 mM DNJ for 16 hr, as well as of untreated WT, UGGT-DKO and EDEM-TKO cells (D). This experiment was performed once.
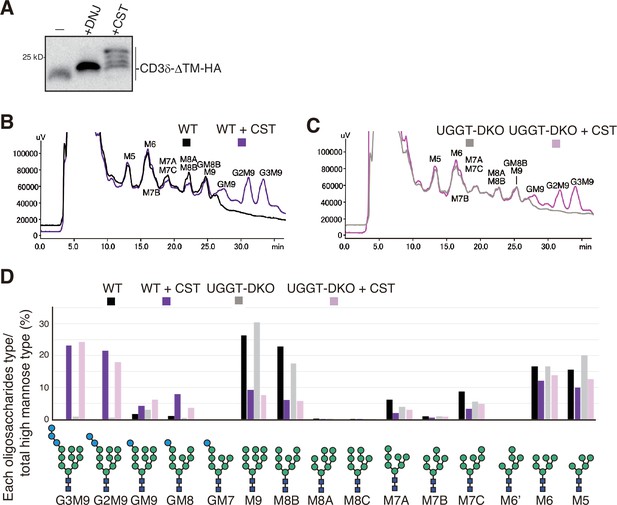
Effect of CST on N-glycan profiling of cells.
(A). Immunoblotting to determine the effect of treatment with 0.5 mM DNJ or 1 mM CST for 6 hr on exogenously expressed CD3δ-ΔTM-HA in wild-type (WT) HCT116 cells. (B, C) Elution profiles of N-glycans from amide column prepared from total cellular glycoproteins of WT (B), and UGGT-DKO (C) cells treated with or without 1mM CST for 16 hr. Each peak was further analyzed by the ODS column to determine the exact amount of each N-glycan composition. (D) Isomer composition of oligomannose-type N-glycans prepared from total cellular glycoproteins of WT and UGGT-DKO cells untreated or treated with 1 mM CST for 16 hr. This experiment was performed once.
-
Figure 1—figure supplement 6—source data 1
A original file for western blot are displayed in Figure 1—figure supplement 6A.
- https://cdn.elifesciences.org/articles/93117/elife-93117-fig1-figsupp6-data1-v1.zip
-
Figure 1—figure supplement 6—source data 2
PDF file containing an original membrane of western blot for Figure 1—figure supplement 6A.
- https://cdn.elifesciences.org/articles/93117/elife-93117-fig1-figsupp6-data2-v1.pdf
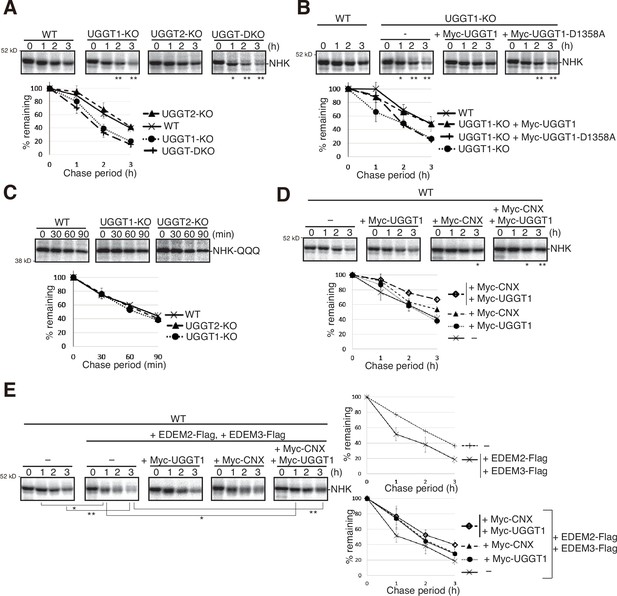
Effect of UGGT1/2-KO on degradation of soluble ER-associated degradation (ERAD) substrates.
(A) Pulse-chase and subsequent immunoprecipitation experiments using anti-α1-PI antibody to determine the degradation rate of null hong kong (NHK) in wild-type (WT), UGGT1-KO, UGGT2-KO, and UGGT-DKO HCT116 cells transfected with plasmid to express NHK (n=3). The radioactivity of each band was determined and normalized with the value at chase period 0 hr. The means from three independent experiments with standard deviations (error bars) are plotted against the chase period (n=3). p-value: *<0.05, **<0.01. (B) Rescue experiments using WT and UGGT1-KO HCT116 cells transfected with plasmid to express NHK with or without co-transfected plasmid to express Myc-UGGT1 or Myc-UGGT1-D1358A (n=3), as in (A). p-value: *<0.05, **<0.01. (C) Pulse-chase and subsequent immunoprecipitation experiments using anti-α1-PI antibody to determine the degradation rate of NHK-QQQ in WT, UGGT1-KO and UGGT2-KO HCT116 cells transfected with plasmid to express NHK-QQQ (n=3), as in (A). (D) Pulse-chase and subsequent immunoprecipitation experiments using anti-α1-PI antibody to determine the degradation rate of NHK in WT HCT116 cells transfected with plasmid to express NHK with or without co-transfected plasmid to express Myc-UGGT1 and/or Myc-CNX (n=3), as in (A). p-value: *<0.05, **<0.01. (E) Pulse-chase and subsequent immunoprecipitation experiments using anti-α1-PI antibody to determine the degradation rate of NHK in WT HCT116 cells transfected with plasmid to express NHK together with or without co-transfected plasmid to express EDEM2-Flag, EDEM3-Flag, Myc-UGGT1 and/or Myc-CNX (n=3), as in (A). p-value: *<0.05, **<0.01.
-
Figure 2—source data 1
Original files for pulse-chase analysis are displayed in Figure 2A–E.
- https://cdn.elifesciences.org/articles/93117/elife-93117-fig2-data1-v1.zip
-
Figure 2—source data 2
PDF file containing original gels of pulse-chase for Figure 2A–E.
- https://cdn.elifesciences.org/articles/93117/elife-93117-fig2-data2-v1.zip
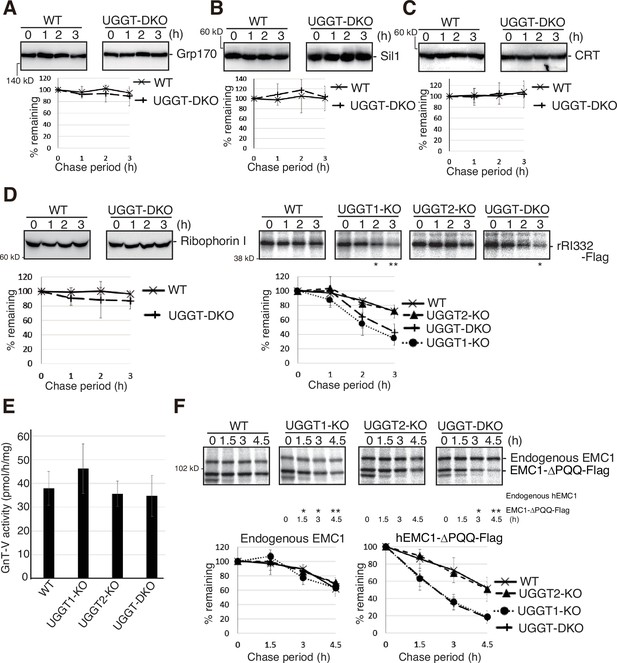
Effect of UGGT1/2-KO on functional proteins in the secretory pathway and on their unstable mutants.
(A–C) Cycloheximide chase (50 μg/ml) and subsequent immunoblotting experiments to determine the degradation rate of endogenous Grp170 (A), Sil1 (B) and CRT (C) in wild-type (WT) and UGGT-DKO HCT116 cells using the respective antibody (n=3). (D) [left] Cycloheximide chase and subsequent immunoblotting experiments to determine the degradation rate of endogenous Ribophorin I in WT and UGGT-DKO HCT116 cells using anti-Ribophorin antibody (n=3), as in Figure 1D. [right] Pulse-chase and subsequent immunoprecipitation experiments using anti-Flag antibody to determine the degradation rate of rRI332-Flag in WT, UGGT1-KO, UGGT2-KO and UGGT-DKO HCT116 cells transfected with plasmid to express rRI332-Flag (n=3), as in Figure 2A. p-value: *<0.05, **<0.01. (E) Determination of N-acetylglucosaminyltransferase-V (GnT-V) activity in cell lysates of WT, UGGT1-KO, UGGT2-KO, and UGGT-DKO HCT116 cells (n=3). (F) Pulse-chase and subsequent immunoprecipitation experiments using anti-ER membrane protein complex subunit 1 (EMC1) antibody to determine the degradation rate of endogenous EMC1 and EMC1-ΔPQQ-Flag in WT, UGGT1-KO, UGGT2-KO and UGGT-DKO HCT116 cells transfected with plasmid to express EMC1-ΔPQQ-Flag (n=3), as in Figure 2A. p-value: *<0.05, **<0.01.
-
Figure 3—source data 1
Original files for western blot and pulse-chase analysis are displayed in Figure 3A–D and F.
- https://cdn.elifesciences.org/articles/93117/elife-93117-fig3-data1-v1.zip
-
Figure 3—source data 2
PDF file containing original membranes of western blots and gels of pulse-chase analysis for Figure 3A–D and F.
- https://cdn.elifesciences.org/articles/93117/elife-93117-fig3-data2-v1.zip
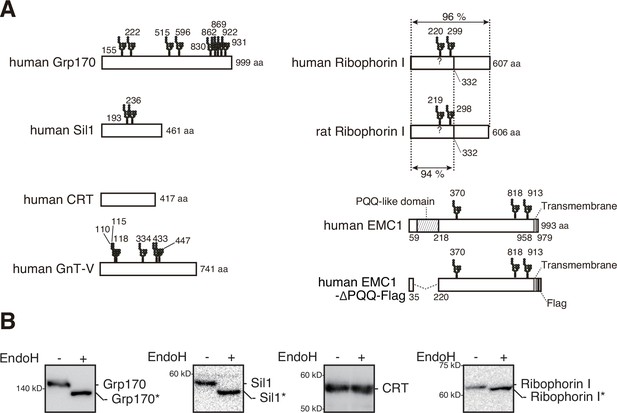
Characterization of various proteins present in the secretory pathway.
(A) Schematic structures of human Grp170, human Sil1, human CRT, human N-acetylglucosaminyltransferase-V (GnT-V), human Ribophorin I, rat Ribophorin I, human ER membrane protein complex subunit 1 (EMC1), and human EMC1-ΔPQQ-Flag with potential N-glycosylation sites indicated. It has not been confirmed whether two N-glycans are attached to human and rat Ribophorin I. Sequence identities between human and rat Ribophorin I are shown as percentages. rRI332-Flag lacks aa 333–606 of rat Ribophorin I. The positions of PQQ-like and transmembrane domains in EMC1 are indicated. (B) Immunoblotting with respective antibodies of wild-type (WT) HCT116 cell lysates treated with or without endoglycosidase H (EndoH).
-
Figure 3—figure supplement 1—source data 1
Original files for western blot are displayed in Figure 3—figure supplement 1B.
- https://cdn.elifesciences.org/articles/93117/elife-93117-fig3-figsupp1-data1-v1.zip
-
Figure 3—figure supplement 1—source data 2
PDF file containing membranes of a western blot for Figure 3—figure supplement 1B.
- https://cdn.elifesciences.org/articles/93117/elife-93117-fig3-figsupp1-data2-v1.pdf
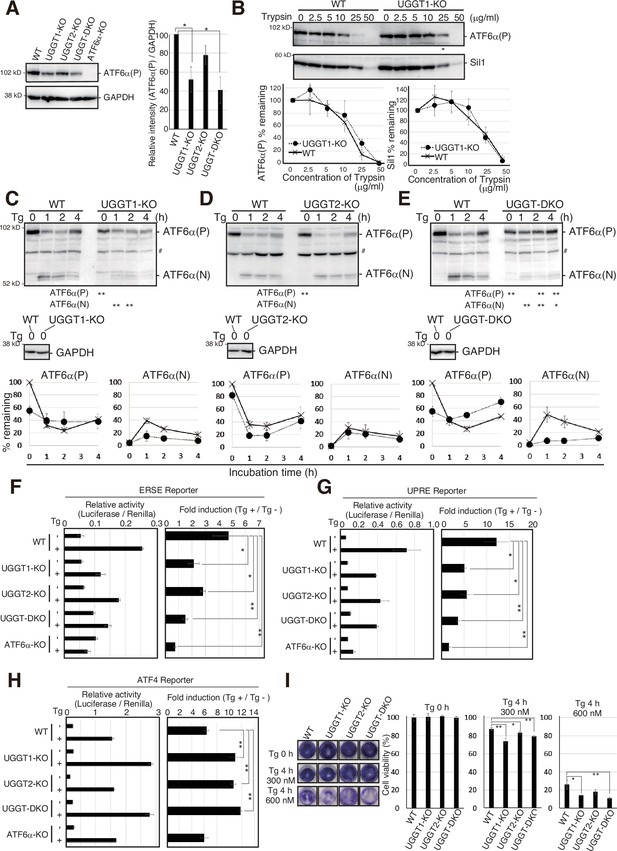
Effects of UGGT1-KO on ATF6α-mediated unfolded protein response (UPR).
(A) Immunoblotting to determine the protein level of endogenous ATF6α(P) in wild-type (WT), UGGT1-KO, UGGT2-KO, UGGT-DKO, and ATF6α-KO HCT116 cells using anti-ATF6α antibody (n=3). p-value: *<0.05. (B) The same amounts of proteins in cell lysates from WT and UGGT1-KO cells were treated with the indicated concentration of trypsin for 15 min at room temperature. Enzymatic activity of trypsin was terminated by the addition of Laemmli SDS sample buffer containing 100 mM DTT and a 10 x protease inhibitor cocktail. Immunoblotting was conducted as in Figure 1D, using anti-ATF6α and anti-Sil1 antibodies. In electrophoresis for the detection of ATF6α, loaded UGGT1-KO samples contained twice as much protein as loaded WT samples to compensate for the decrease in the level of ATF6α(P). (C–E) WT, UGGT1-KO, UGGT2-KO, and UGGT-DKO HCT116 cells were incubated in the presence of 300 nM thapsigargin (Tg) for the indicated periods. The levels of endogenous ATF6α(P), ATF6α(N), and Glyceraldehyde-3-phosphate dehydrogenase (GAPDH) were determined by immunoblotting. Intensity of the unstressed ATF6α(P) band (0 hr) was set to 100%. # indicates a non-specific band. p-value: *<0.05, **<0.01. (F–H) WT, UGGT1-KO, UGGT2-KO, UGGT-DKO, and ATF6α-KO HCT116 cells were transiently transfected with the ER stress responsive element (ERSE) (D), UPR element (UPRE) (E), or activating transcription factor 4 (ATF4) (F) reporters. Twenty-four hours after the transfection, cells were treated with 300 nM thapsigargin (Tg) for 6 hr and then harvested to determine luciferase activity (n=3). Relative activity of Luciferase to Renilla, and fold induction of Tg + relative to Tg – are shown. p-value: *<0.05, **<0.01. (I) Crystal violet assay to determine endoplasmic reticulum (ER) stress sensitivity of WT, UGGT1-KO, UGGT2-KO, and UGGT-DKO HCT116 cells. An equal number of cells of each cell line treated with 300 nM or 600 nM Tg for 4 hr were spread on 24-well plates and cultured without Tg for 5 d. Resulting HCT116 cells were stained with crystal violet and photographed, and then stained cells were solubilized with 1% SDS, and absorbance of the resulting solution was measured at 570 nm. Cell viability in ER-stressed cells was calculated as the ratio of A570 relative to that obtained with unstressed cells. p-value: *<0.05, **<0.01.
-
Figure 4—source data 1
Original files for western blot analysis are displayed in Figure 4A–E.
- https://cdn.elifesciences.org/articles/93117/elife-93117-fig4-data1-v1.zip
-
Figure 4—source data 2
PDF file containing original membranes of western blots for Figure 4A–E.
- https://cdn.elifesciences.org/articles/93117/elife-93117-fig4-data2-v1.zip
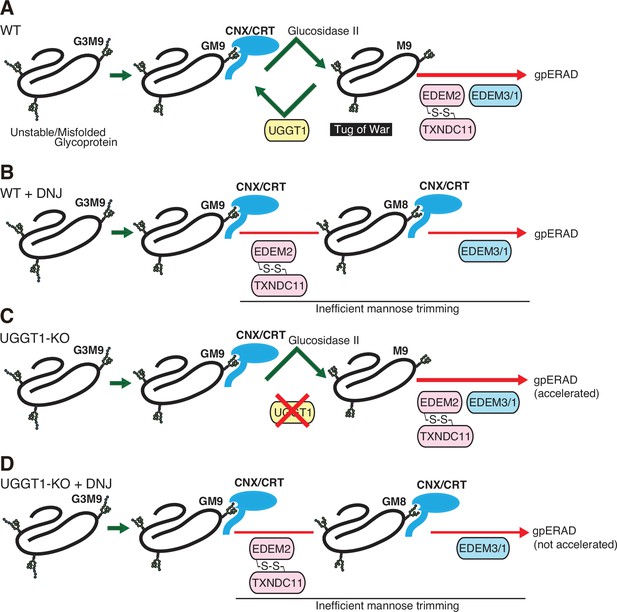
Proposed fate of glycoproteins in the endoplasmic reticulum (ER).
(A) In wild-type (WT) cells, glucosidase II produces glycoproteins with M9, which are either reglucosylated by UGGT1 and then subjected to the calnexin (CNX)/calreticulin (CRT) cycle, or degraded via EDEMs-mediated mannose trimming. Thus, the fate of glycoproteins is determined by a tug-of-war between structure formation by UGGT1 and degradation by EDEMs. (B) In WT cells treated with 1-deoxynojirimycin (DNJ), glycoproteins are not subjected to the CNX/CRT cycle and are degraded by EDEMs-mediated glycoprotein ER-associated degradation (gpERAD). It is likely that mannose trimming from GM9 proceeds more slowly than that from M9, so that glycoproteins are degraded at a similar speed in (A) and (B). (C) In UGGT1-knockout (KO) cells, glycoproteins are directly subjected to premature (accelerated) degradation by EDEMs-mediated gpERAD. (D). In UGGT1-KO cells treated with DNJ, glycoproteins are degraded similarly to (B).
Tables
Reagent type (species) or resource | Designation | Source or reference | Identifiers | Additional information |
---|---|---|---|---|
Cell line | Colorectal carcinoma | ATCC | HCT116; RRID:CVCL_0291 | Authenticated |
Recombinant DNA reagent | p3xFlag-CMV-14 | Ninagawa et al., 2014 | ||
Recombinant DNA reagent | DT-A-pA/loxP/PGK-Puro-pA/loxP | Ninagawa et al., 2014 | ||
Recombinant DNA reagent | DT-A-pA/loxP/PGK-Hygro-pA/loxP | Tsuda et al., 2019 | ||
Antibody | anti-HA; Rabbit polyclonal | Recenttec | Cat#:R4-TP1411100 | WB (1:1000) |
Antibody | anti-HA; Mouse polyclonal | Recenttec | Cat#:R4-TM1422100 | WB (1:1000) |
Antibody | anti-UGGT1; Rabbit polyclonal | Sigma | Cat#:HPA015127 | WB (1:1000) |
Antibody | anti-UGGT2; Rabbit polyclonal | GeneTex | Cat#:GTX103837; RRID:AB_11164993 | WB (1:1000) |
Antibody | anti-GAPDH; Rabbit polyclonal | Trevigen | Cat#:2275-PC-100 | WB (1:1000) |
Antibody | anti-Myc; Rabbit polyclonal | MBL | Cat#:MBL562 | WB (1:1000) |
Antibody | anti-Myc; Mouse monoclonal | Wako | Cat#:011–21874 | WB (1:1000) |
Antibody | anti-ATF6; Rabbit polyclonal | Haze et al., 1999 | WB (1:1000) | |
Antibody | anti-A1AT; Rabbit polyclonal | Dako | Cat#:A0012 | WB (1:1000) |
Antibody | anti-Flag; Mouse monoclonal | Sigma | Cat#:F3165 | WB (1:1000) |
Antibody | anti-Grp170; Rabbit polyclonal | GeneTex | Cat#:GTX102255; RRID:AB_1950534 | WB (1:1000) |
Antibody | anti-Sil1; Rabbit polyclonal | GeneTex | Cat#:GTX116755; RRID:AB_10617803 | WB (1:1000) |
Antibody | anti-Ribophorin I; Rabbit monoclonal | Abcam | Cat#:ab198508 | WB (1:1000) |
Antibody | anti-CRT; Rabbit monoclonal | Enzo Life Sciences | Cat#:ADI-SPA-600; RRID:AB_10618853 | WB (1:1000) |
Additional files
-
Supplementary file 1
Primers table used in this study.
- https://cdn.elifesciences.org/articles/93117/elife-93117-supp1-v1.docx
-
MDAR checklist
- https://cdn.elifesciences.org/articles/93117/elife-93117-mdarchecklist1-v1.docx