Spinal V1 inhibitory interneuron clades differ in birthdate, projections to motoneurons, and heterogeneity
Figures
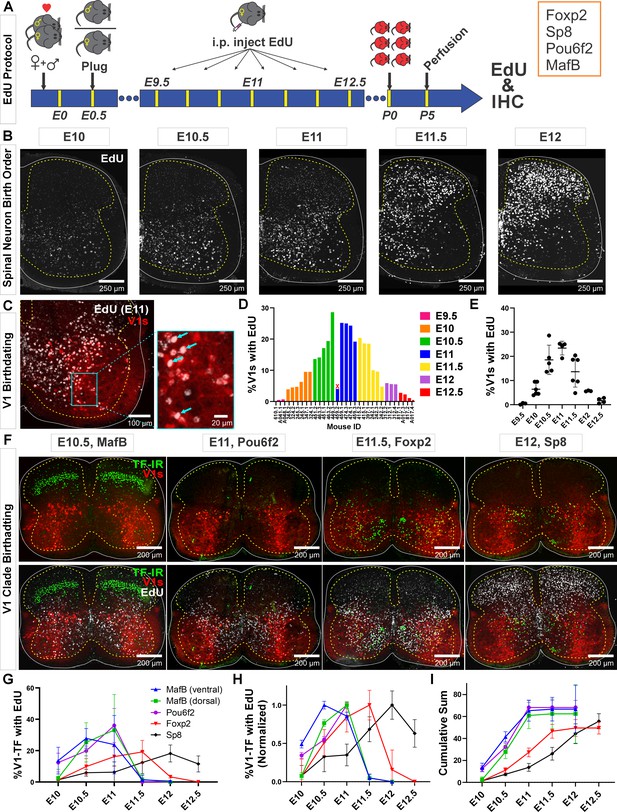
Neurogenesis order of V1 clades assayed by 5-ethynyl-2'-deoxyuridine (EdU) birthdating.
(A) Experimental design. Timed pregnant En1cre,Ai9 R26lsl-tdT females were EdU-injected at one of seven time points between E9.5 and E12.5, and the spinal cords harvested at P5. Tissue sections were processed for EdU (Click-iT) and immunostained for representative transcription factors (TFs) of major V1 clades. MafB was used to identify the MafA-Renshaw cell clade by location (‘ventral MafB-V1 cells’). (B) EdU labeling at different embryonic times. Spinal neurons are born in a ventrolateral to dorsomedial sequence. (C) Example of E11 EdU labeling in an En1-tdT spinal cord. EdU integrated in the DNA at the time of injection is diluted with subsequent cell divisions. To ensure we sampled V1 cells that incorporated EdU during S-phase after their final division, we only counted V1 cells with nuclei filled by EdU Click-iT reaction (arrows). Partially labeled nuclei (speckles) in the image were not counted. (D) Percentages of V1 cells labeled with ‘strong’ EdU in each mouse. The x-axis indicates individual animals (‘<litter number>.<animal number>’). The percentage of EdU-labeled V1 interneurons at each time point was consistent, although we also noted variability between litters and among animals within a litter. One animal (459.2) showed the wrong EdU pattern for its injection age and was discarded (indicated by an X). (E) EdU birthdating reveals a peak in V1 neurogenesis around E11 (error bars = SD; each dot represents one animal). At the time points flanking the peak there is a larger amount of variability, suggesting a fast-changing pace in V1 neurogenesis. (F) Representative images of TF antibody staining combined with EdU labeling to determine birthdates of defined V1 clades. The time points represented were selected according to the maximal or near-maximal generation of V1 interneurons in each clade. (G–I) V1-clade neurogenesis quantification. Graphs represent the average ± SEM calculated from n=3.9 ± 0.3 mice per TF/date (not all TFs were tested in all mice). For each mouse average, we analyzed four ventral horns in Lumbar 4 or 5 segments (further details of sample structure are explained in the results section). (G) Percentage of V1s expressing each clade-specific TF labeled with EdU at each embryonic time point. Ventral MafB-V1s (Renshaw cells) and Pou6f2-V1s are mostly born before E11 (dorsal MafB-V1s are a subgroup of Pou6f2-V1s). Foxp2-V1 and Sp8-V1 interneurons have wider windows of neurogenesis, but most are generated after E11. (H) Data normalized to the maximum percentage of V1s born in each group showing peak generation in each clade. Ventral MafB-V1s, Pou6f2-V1s, Foxp2-V1s, and Sp8-V1s have progressively later times of peak generation. (I) Cum-sum graphs of V1-clades neurogenesis. Between 50% and 68% of all neurons in each V1 clade are labeled across all ages. By E11 nearly all neurons in early clades are generated, while fewer than half of neurons in late clades are.
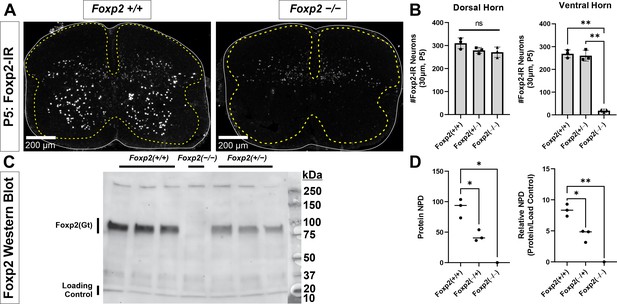
Foxp2 antibody characterization.
(A) P5 spinal cords from wild-type (WT) (Foxp2+/+) and Foxp2 knockout mice (KO: Foxp2flpo/flpo) immunostained with Santa Cruz goat polyclonal anti-Foxp2 antibody (N-16, Lot#G2911). All nuclear immunostaining in the ventral horn and lateral dorsal horn are absent in KO tissues, however some weak immunoreactivity remains in the dorsal horn. This suggests a weak cross-reaction with another epitope present only in dorsal horn cells. (B) Quantification of immunoreactive cell nuclei in the dorsal and ventral horns. For these analyses, a line was drawn horizontally from the top of the central canal to separate dorsal and ventral horns. In 30-µm-thick sections from P5 tissue, the number of Foxp2-IR cells in the dorsal horn is similar in WT (Foxp2+/+), Foxp2 hets (Foxp2flpo/+), and Foxp2 KOs (Foxp2flpo/flpo). Each dot represents one spinal cord serial section from three littermates each with a different genotype. A one-way ANOVA detected no significant differences (F(2,6) = 3.100, p=0.1190). This is likely because the number of weakly labeled spots greatly outnumbers the strongly labeled nuclei that disappear from the image. Thus, the comparison does not have enough power for the expected, relatively small difference in number (power for α=0.05:0.275 which is smaller than desired power of 0.800). We did not pursue this further because our focus is on ventral horn Foxp2 neurons. In the ventral horn there are mostly strongly labeled nuclei and, correspondingly, all disappear from the spinal cord with only a few exceptions of weak labeling. A one-way ANOVA detected significant differences (F(2,6) = 3.100, p<0.0001) and this comparison was adequately powered (power for α=0.05:1.000). Post hoc pairwise comparisons (Bonferroni-corrected t-tests) showed that WT (Foxp2+/+) and Foxp2 hets (Foxp2flpo/+) were significantly different to Foxp2 KOs (Foxp2flpo/flpo) (WT vs KO: p<0.0001 t(6)=17.41; het vs KO: p<0.0001 t(6)=16.83) but not in between them (WT vs het: p>0.9999 t(6)=0.5797) suggesting that heterozygosis did not affect the number of ventral horn interneurons detected using immunolabeling. In summary, our antibody detects Foxp2 expression in the ventral horn with high sensitivity and specificity. (C) Antibody specificity confirmed via western blot. Spinal cords from P5 mice from a single litter were extracted and immediately homogenized in a nuclear extraction solution containing protease inhibitors (NE-PER Nuclear and Cytoplasmic Extraction [Thermo Fisher]; cOmplete Protease Inhibitor Cocktail [Roche]). The nuclear fraction was used for the protein assay and subsequent western blot. Blots were stained with antibodies against Foxp2 (N-16, Santa Cruz) and, as a loading control, against acetyl-Histone H3 (Rabbit, EMD Millipore, 06-599). A single Foxp2 band is revealed at the expected theoretical 80 kDa molecular weight. This band disappears in the Foxp2 KO lane (Foxp2flpo/flpo). (D) Western blot quantification. Protein content was estimated measuring the normalized pixel density (NPD = pixel density in ROI encompassing stained bands – pixel density of same ROI placed just above the band) in the Foxp2 band and relative to the NPD of the respective loading controls in each lane. Foxp2 protein was undetectable in the Foxp2 KO lane (Foxp2flpo/flpo). In WT animals we detected almost double the amount of Foxp2 protein compared to hets (Foxp2flpo/+), and this difference was significant (two-tailed t-test t(4) = 5.004, p=0.0075). This suggests that Foxp2 gene expression is halved in hets, but this does not affect detectability of Foxp2 ventral horn interneurons which were similar in number in hets vs WTs (see B). In this graph each dot is one animal/lane and the average ± SD indicated. Raw images of the blots and corresponding labeling are found in Figure 1—figure supplement 1—source data 1 and Figure 1—figure supplement 1—source data 2.
-
Figure 1—figure supplement 1—source data 1
Raw images of gel corresponding to Figure 1—figure supplement 1C.
- https://cdn.elifesciences.org/articles/95172/elife-95172-fig1-figsupp1-data1-v1.zip
-
Figure 1—figure supplement 1—source data 2
Annotated full image of gel corresponding to Figure 1—figure supplement 1C.
- https://cdn.elifesciences.org/articles/95172/elife-95172-fig1-figsupp1-data2-v1.pdf
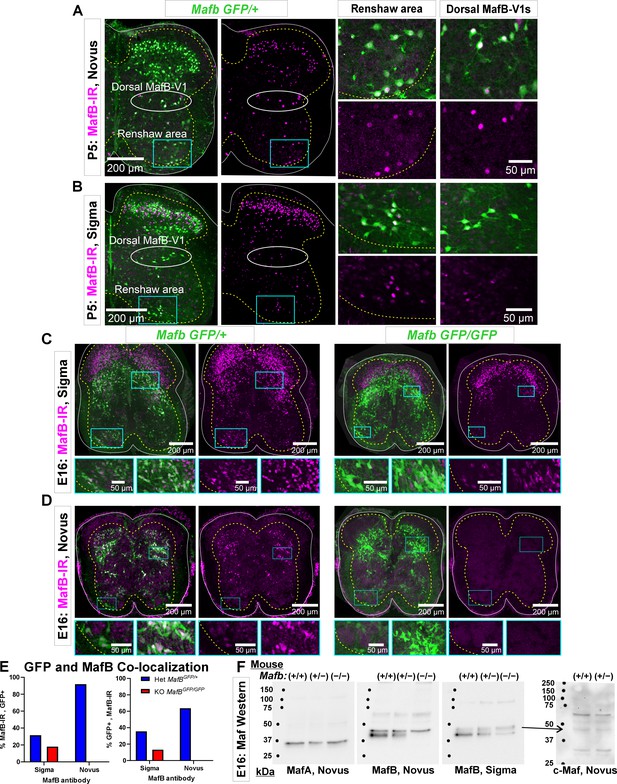
Each antibody was directed against different regions of the mouse MafB protein: aa18–167 for the Sigma antibody (Lot#A31532) and aa100–150 for the Novus antibody (lot#1).
These target sequences are shared with mouse MafA and c-Maf, but the overlap is larger with the Sigma antibody immunogen. A BLAST sequence search found 98%, 53%, and 64% significant alignment between the MafB sequence used for creation of the Sigma antibody with, respectively, aa18–140 in mouse MafB, aa18–143 in mouse MafA, and aa19–118 in mouse c-Maf. The sequence used in the Novus antibody detected no alignment with proteins other than mouse MafB. (A) P5 spinal cord from a MafbGFP/+ mouse immunostained with MafB-Novus antibodies. GFP (green) reports mafb gene expression (see Figure 1—figure supplement 3). Immunohistochemistry detects MafB protein (Cy3, magenta). Higher magnifications of the indicated areas, including the dorsal and ventral (Renshaw) MafB-V1 groups, are shown to the right. (B) P5 spinal cord from a MafbGFP/+ mouse immunostained with MafB-Sigma antibodies. The MafB-Sigma antibody detects many more dorsal horn neurons than the MafB-Novus antibody. Many interneurons at this location are known to express c-Maf. In the ventral horn MafB-immunoreactivity is qualitatively similar for both antibodies. This includes both the regions occupied by V1 Renshaw cells and by dorsal-MafB-V1s (Pou6f2-V1 clade). (C) Immunoreactivity against MafB-Sigma antibodies in het (MafbGFP/+) and KO (MafbGFP/GFP) tissue from E16 MafB-GFP reporter mice (the MafB KO is lethal at P0 because it cannot breathe on its own). Low-magnification images of MafB-GFP and MafB-immunoreactivity combined (left panel) or MafB-immunoreactivity alone (right panel) in the het (left pair) and the KO mouse (right pair). The boxed ventral and dorsal horn areas are magnified below. GFP in MafB-GFP mice reports activity of the mafb promoter, but the knocked-in GFP inactivates the Mafb allele. When both alleles carry GFP (KO, MafbGFP/GFP), GFP reports cells with gene expression from the Mafb locus although no Mafb mRNA or protein is produced. In the het mouse one allele produces Mafb mRNA: in this tissue, there is a high degree of overlap between GFP and protein immunoreactivity in the ventral and deep dorsal horns. However, there is more MafB-Sigma immunoreactivity than GFP in superficial laminae. In the KO, ventral horn immunoreactivity is greatly diminished, but lingering weak immunoreactivity remains in many neurons, including Renshaw cells. This could represent cross-reaction with MafA in the tissue. Most MafB-Sigma immunoreactivity in superficial laminae remains in the KOs suggesting that these cells strongly express a cross-reacting target and frequently do not express Mafb (GFP negative). This is most likely c-Maf which is highly expressed in laminae I to III neurons. (D) As in C, for MafB-Novus antibodies. Unlike MafB-Sigma, there is little MafB-immunoreactivity in the het animal outside GFP+ cells reporting MafB expression; this includes superficial laminae cells. All immunoreactivity disappears in the KO animal. (E) Ventral horn co-localization of MafB-GFP and MafB-immunoreactivity obtained with Sigma and Novus antibodies. Graphs show the percentage of immunoreactive cells that are GFP+ (left) and the percentage of GFP+ cells that co-localize the indicated MafB antibody immunoreactivity (right). In one spinal cord ventral horn section from a het mouse (blue bars), we sampled 441 cells with MafB-Sigma immunoreactivity, but the same section had fewer MafB-GFP cells (n=392) such that only a small number of cells co-localized both markers (n=139). In total, 31.5% of MafB-Sigma immunoreactive cells expressed GFP and 35.5% of GFP+ cells had MafB-Sigma immunoreactivity. Conversely, in a serial section immunolabeled with the Novus antibody we detected fewer MafB-Novus immunoreactive cells (n=149) than MafB-GFP cells (n=215) and the large majority were GFP+ (92.0%, n=137). There was almost no MafB-Novus immunoreactivity outside GFP+ cells. Thus, the MafB-Novus antibody is more restricted to GFP+ cells than the MafB-Sigma antibody. In addition, 63.7% of GFP+ cells express MafB-Novus immunoreactivity and while putative dorsal-MafB V1 cells express strong MafB-Novus immunoreactivity this is weak in Renshaw cells. In one MafB KO section (red bars) we detected 217 MafB-Sigma immunoreactive cells and 296 GFP+ cells with 39 cells co-localizing both markers. This corresponded to 17.9% of MafB-immunoreactive cells expressing GFP and 13.2% of the GFP+ cells expressing MafB-Sigma immunoreactivity. The MafB-Novus antibody showed no MafB-immunoreactivity in KO tissue sections with similar numbers of GFP+ cells (n=241). (F) Western blots of MafA (Novus), MafB-Novus, and MafB-Sigma on nuclear extracts from one WT (Mafb+/+), one Mafb heterozygous (het, MafbGFP/+), and one Mafb knockout (KO, MafbGFP/GFP), all E16 littermates. The same western blot was stripped and re-probed three times with the antibodies in the following order: MafB-Novus, MafA-Novus, and finally MafB-Sigma. The MafA antibody shows a single band just below the 37 kDa marker, aligning with a predicted molecular weight of 37.6 kDa. The band is of similar size in all three lanes suggesting no compensatory change in MafA expression in Mafb hets and KOs. Neither of the MafB antibodies detected the MafA band in western blots, suggesting that any possible cross-reaction in tissue is due to IgG species detecting secondary or tertiary protein structures. MafB antibodies generated a double band, with the upper band being weaker than the lower band for MafB-Sigma compared to MafB-Novus. The immunoreactivity of the lower band to MafB-Sigma diminished in hets compared to WTs and diminished further in the KO. This band completely disappeared in the KO probed with MafB-Novus antibodies. This suggest that this band corresponds to MafB and occurs at approximately 40 kDa, slightly over the predicted 35.8 kDa molecular weight. Both antibodies detected a higher molecular weight band that does not change with gene dose. This could correspond to c-Maf with a larger predicted molecular weight, 38.5 kDa. Therefore, we performed a new western blot using a c-Maf antibody from Novus, and we found a correspondence between the upper band detected by both MafB antibodies with one of the bands in the c-Maf western blots. This suggests that in western blots both MafB antibodies cross-reacted with c-Maf. Raw images of the blots and corresponding labeling are found in Figure 1—figure supplement 2—source data 1, Figure 1—figure supplement 2—source data 2, and Figure 1—figure supplement 2—source data 3.
Characterization of MafB (Novus, NB600-266) and MafB (Sigma, HPA00563) antibody immunoreactivities in the spinal cord.
-
Figure 1—figure supplement 2—source data 1
Raw images of triple probed gel corresponding to Figure 1—figure supplement 2F.
- https://cdn.elifesciences.org/articles/95172/elife-95172-fig1-figsupp2-data1-v1.zip
-
Figure 1—figure supplement 2—source data 2
Annotated images of triple probed gel corresponding to Figure 1—figure supplement 2F.
- https://cdn.elifesciences.org/articles/95172/elife-95172-fig1-figsupp2-data2-v1.pdf
-
Figure 1—figure supplement 2—source data 3
Annotated full image of c-Maf probed gel corresponding to Figure 1—figure supplement 2F.
- https://cdn.elifesciences.org/articles/95172/elife-95172-fig1-figsupp2-data3-v1.pdf
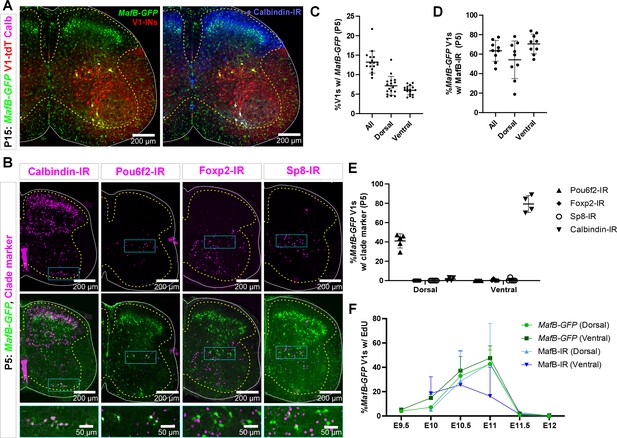
MafB-V1s visualized in a MafB-GFP mouse model.
(A) MafB-GFP+ cells in mature (P15) mice. Most MafB-GFP+ cells in lamina VII belong to the V1 lineage (tdTomato). They are found in the most dorsal and ventral regions of the distribution of V1s. Those that are also calbindin-IR fall in the distinctive ventral region occupied by Renshaw cells. In addition, there are many dorsal horn non-V1 MafB-GFP+ neurons. The small cells throughout the white and gray matter are microglia. (B) Expression of transcription factors (TFs) and calbindin in MafB-GFP+ cells in neonatal (P5) mice. Top row, immunoreactivity for calbindin (Renshaw cells) and clade-specific TFs. Bottom row, superimposition with MafB-GFP (V1-tdTomato is not shown for simplicity). Compared to P15, a few more ventral horn neurons express MafB-GFP at P5, including some motoneurons, but MafB labeling is weaker in these cells. Within MafB-GFP+ V1 neurons, the two groups located at the most dorsal and most ventral regions correspond to the V1 neurons that retain MafB-GFP at P15 (see A). These groups are indicated with rectangles in the figure. The ventral group expresses calbindin. The dorsal group expresses Pou6f2 at this age. Little-to-no Foxp2-IR or Sp8-IR is found in either dorsal or ventral groups of MafB-GFP+ V1s. (C) Quantitation of MafB-V1 neurons in P5 mice. Around 13% of all V1s express MafB-GFP+, and the percentages located in the Renshaw cell area (‘ventral’) or dorsal lamina VII (‘dorsal’) are evenly split (n=17 mice, 4 ventral horns each; bars show SD). (D) More than half of the MafB-GFP+ V1 cells have detectable levels of MafB (Sigma) immunoreactivity at P5, in both the ventral and dorsal groups (n=9 mice, 4 ventral horns each; error bars show SD). (E) V1-clade marker expression in dorsal and ventral P5 MafB-GFP V1 neurons. Dorsal MafB-GFP-V1s express Pou6f2, but do not express calbindin, Sp8, or Foxp2. Ventral MafB-GFP-V1s express calbindin (Renshaw cells) and do not express Pou6f2, Foxp2, or Sp8 (n=4–5 mice, 4 ventral horns each; error bars show SD). (F) 5-Ethynyl-2'-deoxyuridine (EdU) birthdating reveals similar proportions of EdU+ neurons at P5 for dorsal MafB-GFP vs MafB-IR neurons pulse-labeled at each embryonic time point (n=2 mice per time point per condition, 4 ventral horns each; error bars show SD). The mismatch in birthdates between MafB genetic and antibody labeling at E11 in the ventral group could arise because some E11-born ventral MafB-GFP V1s quickly downregulate MafB to levels that are undetectable with antibodies.
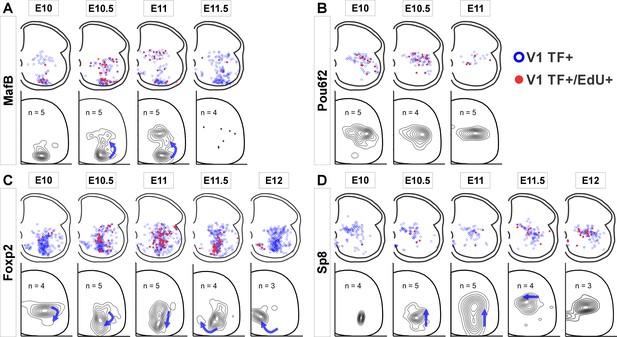
V1 interneurons born at different embryonic times settle in different locations.
Top row in each panel: blue open dots indicate all V1 interneurons positive for each transcription factor (TF) at P5, and filled red dots indicate those with strong 5-ethynyl-2'-deoxyuridine (EdU). Each plot is from one representative animal injected at each of the indicated embryonic times (four ventral horns from each animal are superimposed in the diagram). Bottom rows: cellular density profiles of V1 interneurons positive for each transcription factor (TF) and with strong EdU. Cellular density profiles represent all cells sampled in all animals EdU-injected at the indicated embryonic times; n indicates the number of animals in each plot (4 ventral horns analyzed per animal). Contour plots are derived from 2D kernel density estimates of interneuron positions; lines encompass 10% increments. Only time points with representative numbers of TF+/EdU+ cells are shown. Blue arrows highlight major directional changes in settling locations for V1s born at each time point with respect to the previous time point. (A) MafB-V1s are divided into two groups: ventral Renshaw cell MafB-V1s and dorsal MafB-Pou6f2-V1s with different birthdates. (B) Pou6f2-V1s (taken as a whole) are born between E10 and E11.5 and always settle dorsally. (C) Foxp2-V1s show variations in location according to birthdate. Cells of increasingly older birthdates settle dorsally, laterally, ventrolaterally, and then ventromedially. (D) A few Sp8-V1s are born early and are located ventrally, but the majority are born later and settle dorsally. Directional changes in settling positions according to birthdate differ among V1 clades.
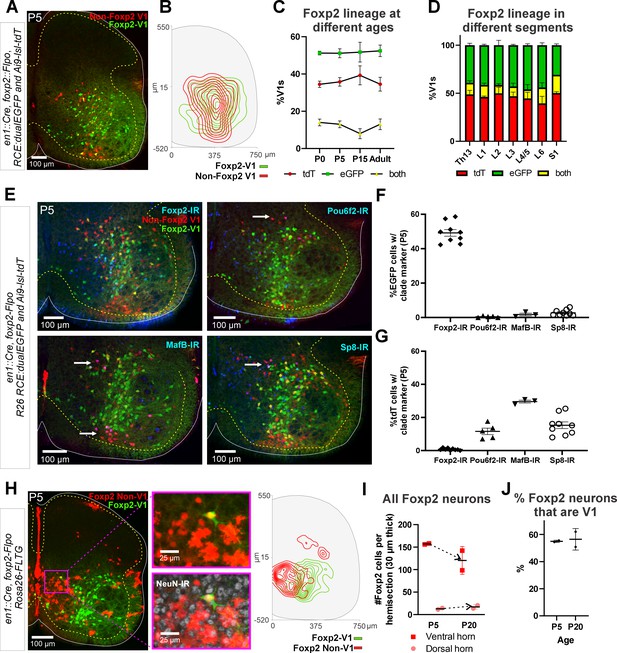
Genetic mouse models to label the Foxp2-V1 lineage.
(A) P5 mouse intersection of En1Cre/+ and Foxp2Flpo/+ with two reporter alleles (Ai9 R26 lsl-tdT and R26 RCE:dualEGFP). Foxp2-V1s express EGFP (green) and non-Foxp2-V1s tdTomato (red). A few ‘yellow cells’ correspond with Foxp2-V1 neurons that failed to remove the tdTomato Ai9 reporter. (B) Density contours demonstrate high spatial overlap between V1 neurons expressing Foxp2 (EGFP+) or not (tdT+ and EGFP-) (n=6 ventral horns). (C) Expression of lineage labels are stable throughout postnatal development, suggesting no additional Foxp2 gene upregulation in V1s after P0 (n=6 ventral horns per age in one animal, except for P5 in which 6 animals and 36 ventral horns are included; error bars show SEM). (D) Lineage labeling in P15 mice is uniform across all spinal cord segments from thoracic 13 to sacral 1 (n=6 ventral horns in each segment from 2 mice; error bars show SEM). (E) Foxp2-V1 (EGFP) and non-Foxp2 V1 (tdT) lineage labeling with antibody staining for the transcription factors defining the four major V1 clades: Foxp2, Pou6f2, MafB, and Sp8. The Foxp2-V1 lineage contains all P5 Foxp2-expressing V1s and excludes almost all those expressing the markers of other clades. (F) Percentages of Foxp2-V1s (EGFP) expressing the clade markers at P5. Around half of the Foxp2-V1s maintain expression of the Foxp2 protein at P5, and a minimal number of these cells express transcription factors that define other V1 clades. (G) Percentages of non-Foxp2 lineage V1s (tdT) expressing the different V1 clade markers. Cells outside of the Foxp2-V1 lineage do not express Foxp2 at P5, and this subset contain V1s from the three other clades (for both plots n=6.5 ± 2.6 mice, 4 ventral horns each; error bars show SEMs). (H) FLTG reporter mice reveal lineage-labeled non-V1 Foxp2 cells in the spinal cord. EGFP is expressed in Foxp2-V1s and tdTomato in non-V1 Foxp2 cells. The zoomed images of the highlighted region with and without NeuN-IR demonstrate that non-V1 Foxp2 cells include non-neuronal cell types with astrocyte morphologies. Only neurons (NeuN-IR) are included in the cell density contour plots; non-V1 Foxp2 neurons are located in the medial ventral horn and a few in the deep dorsal horn (n=2 animals at P5, 6 ventral horns in each). (I) Most Foxp2-neurons (red, non-V1s or green, V1s) are in the ventral horn and their number in 30-μm-thick L4-5 sections decreases with age as the neuropil matures and expands in size. Dorsal horn Foxp2-neurons maintain their numbers despite the growth of the spinal cord, suggesting de novo postnatal Foxp2 expression in this population. Each point is one animal analyzed through 6 ventral horns (errors bars are SD). (J) Percentage of Foxp2-lineage-labeled cells that are Foxp2-V1s remains constant throughout postnatal development (n=2 mice, 6 ventral horns each; error bars show SD).
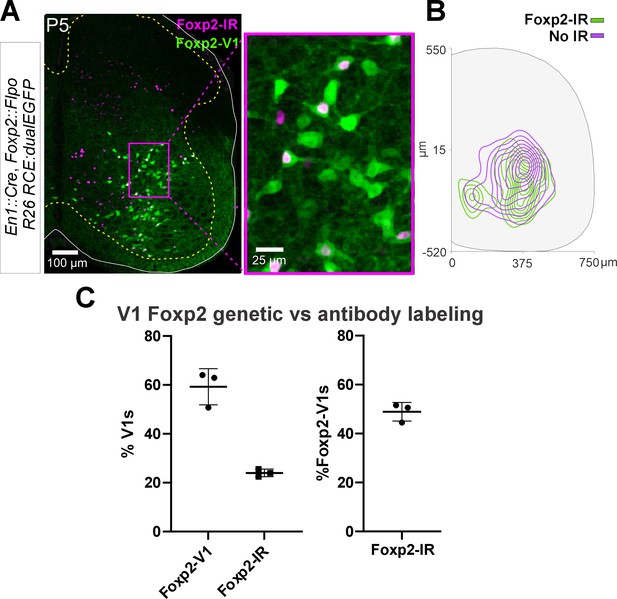
Postnatal downregulation of Foxp2 expression.
(A) Confocal image of En1Cre/+, Foxp2flpo/+, R26RCE:dualEGFP genetic labeling (green) immunostained for Foxp2 protein expression (magenta) in a P5 mouse. Low-magnification 2D projection at the left. Higher magnification of the indicated area at the right. Only a proportion of lineage label Foxp2-V1s express Foxp2 protein by P5. (B) Density contours demonstrate that lineage-labeled Foxp2-V1s with or without Foxp2 protein expression at P5 occupy similar areas in the spinal cord at P5 (n=3 mice, 3 ventral horns per animal). (C) Left, Foxp2-V1s make up roughly 60% of all V1s when measured with genetic labeling, but only about 30% of V1s maintain detectable Foxp2 expression at P5 (n is same as B; error bars show SD). Right, around half of Foxp2-V1s maintain detectable levels of Foxp2 at P5 (same data).
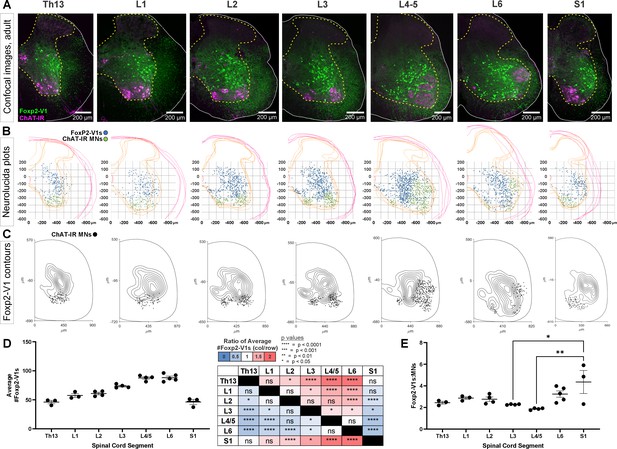
Foxp2-V1 interneurons are closely associated with shifting motor columns throughout thoracic, lumbar, and sacral levels of the spinal cord.
(A) Foxp2-V1 lineage labeling and choline acetyltransferase (ChAT) antibody staining for motoneuron identification in adult mouse spinal cords from thoracic to sacral levels. Foxp2-V1 neurons accumulate at the lateral edge of the ventral spinal cord but their locations shift when the lateral motor column (LMC) expands from L3 to L6. In addition, a distinct group of Foxp2-V1 interneurons is dispersed at ventromedial locations in lumbar segments. (B) Plots of Foxp2-V1 and ChAT-IR motoneuron cell body positions in x,y coordinates with 0,0 at the top of the central canal (n=4 ventral horns, 1 representative animal). (C) Contour plots of kernel Foxp2-V1 cell density estimations. The highest density of Foxp2-V1 neurons cluster close to LMC motoneurons from L2 to L5 (contours enclose 10% increments, closer lines indicate steeper changes in density). Motoneuron numbers progressively increase from Th13 to L5 and drop in number in L6 and S1. (D) Number of Foxp2-V1s per 50-μm-thick section (ventral horn) significantly increases in lower lumbar segments from L3 to L6 compared to S1 (dots represent individual mice; n=3–5 mice in different segments, each mouse estimate is from 6 ventral horns; bars show SEM). One-way ANOVA, post hoc Bonferroni-corrected t-tests are summarized in the right-hand table (further statistical details in Supplementary file 1a). (E) Ratios of Foxp2-V1 neurons to MNs remain constant at roughly 2.5:1 with no significant changes throughout the lumbar cord. Significance was only found for L3-L5 compared to S1 (*p<0.05; **p<0.01; post hoc Bonferroni tests). High variability in S1 is likely due to the sharp rostro-caudal decrease in motoneuron numbers in S1.
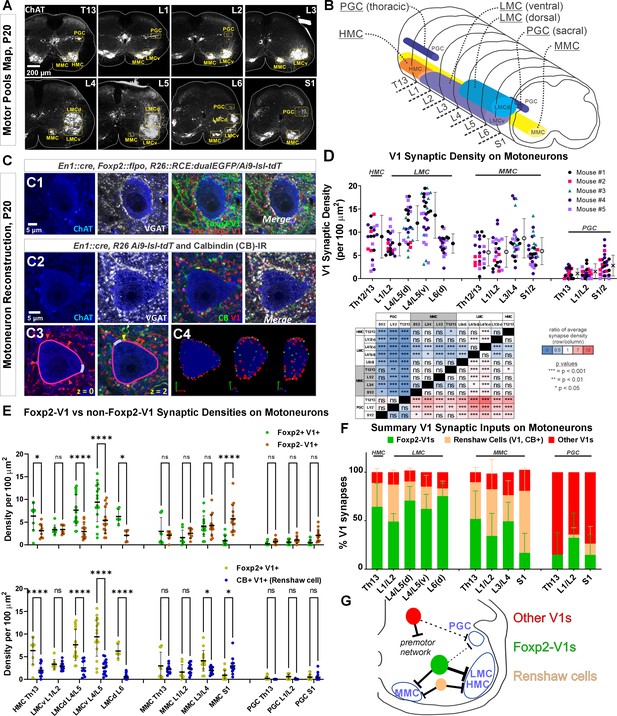
Limb and axial motoneurons are densely innervated by Foxp2-V1s and Renshaw cells.
(A) Motor column identification from lower thoracic to upper sacral spinal cord in P20 mice following labeling with choline acetyltransferase (ChAT) antibodies: PGC = preganglionic cell column; MMC = medial motor column; HMC = hypaxial motor column; LMCd/v=lateral motor column (dorsal/ventral). (B) Schematic representation of the rostro-caudal span of each motor column in the spinal segments studied. (C) Synapse quantification. Axons of Foxp2 and non-Foxp2 V1 interneurons were respectively labeled with EGFP and tdT in En1Cre, Foxp2flpo, R26 CE:dualGFP/Ai9-lsl-tdT mice. In en1Cre, Ai9 R26lsl-tdT mice we identified V1-Renshaw cell axons using calbindin antibodies. Synaptic locations were labeled with VGAT antibodies and the postsynaptic motoneurons with ChAT antibodies. Synapse densities were analyzed in a ribbon of membrane at mid-cell body level (7 optical planes, 1 µm z-step). C1, Single optical plane of an L4/5 LMCv motoneuron surrounded by genetically labeled Foxp2-V1 and non-Foxp2-V1 axons. Inhibitory synapses on ChAT-IR motoneurons are VGAT+. C2, Single optical image of an L4/5 LMCv ChAT-IR motoneuron receiving synapses from V1 Renshaw cells (genetically labeled V1 axons with calbindin-IR and VGAT). C3–4, Method for estimating synapse densities on motoneuron cell bodies using C2 as example. C3, V1-VGAT (red arrowheads) and V1-CB-VGAT synapses (yellow arrowhead) are marked (VGAT-IR is not shown for clarity), and the cell body contour annotated with regions corresponding to dendrite exits. This process was repeated in seven consecutive mid-cell body optical planes (cross-sections with well-defined nucleus and nucleolus). C4, A membrane surface slab is reconstructed in 3D (two different rotations shown). The surface area corresponding to dendrite exits is subtracted from the total surface area of the slab to calculate the available surface area on the motoneuron cell body. V1-VGAT synapses (red), V1-CB-VGAT synapses (yellow), and CB-VGAT synapses (green) are marked. A similar process was followed for calculating Foxp2-V1 synapse density. (D) Quantification of total V1-VGAT synapse densities on motoneuron cell bodies in different motor columns (n=21–30 motoneurons per motor column, n=5 animals with 4–9 motoneurons per animal per motor column). Each data point is one motoneuron color-coded by mouse origin. Average synaptic densities ± SD indicated to the right of scatter plots. A nested ANOVA found significant differences among motor column/segments (p<0.0001) with no inter-animal variability (p=0.4768). The table summarizes all post hoc pairwise comparisons for average V1 synaptic densities of each motor column and segment (Bonferroni-corrected t-tests) (further statistical details are found in Supplementary file 1b). Colors indicate increased (>1, red) or decreased ratios (<1, blue) of column motoneurons vs row motoneurons. PGC neurons receive significantly fewer V1 synapses than MMC or LMC motoneurons. The LMC (ventral and dorsal) in lower lumbar (L4/L5) had significantly more V1 contacts than MMC motoneurons or L6 dorsal LMC. (E) Comparison of synaptic densities from Foxp2-V1 and non-Foxp2-V1 neurons (top) or Renshaw cells (bottom). All motoneurons sampled in 2–3 animals for each comparison were pooled together. Densities of V1-VGAT synapses from Foxp2-V1s, non-Foxp2 V1s, or calbindin (CB)+ V1s (Renshaw cells) (n=6–17 motoneurons sampled per motor column/segments, average = 12.1 ± 2.9 SD) were compared using a two-way ANOVA for axon type vs motor column and segment. Foxp2-V1 vs non-Foxp2-V1 synapses: significant differences in density were found for type of synapse (p=0.001), motor column location (p<0.0001), and their interaction (p<0.0001). Significant differences after post hoc Bonferroni tests are indicated (*p<0.05; ****p<0.0001). In general, synapses from Foxp2-V1 axons have higher density than non-Foxp2-V1 axons on HMC and LMC columns at all spinal segments except for L1/L2 LMC. MMC motoneurons receive similar synaptic densities from both types of V1 axons, except at the sacral level in which non-Foxp2 V1 synapses predominate. PGC neurons receive very low densities of V1 axons and there are no significant differences between either type in any region. Foxp2-V1 vs CB+ V1 synapses: significant density differences were found for type of synapse (p<0.0001), motor column location (p<0.0001), and their interaction (p<0.0001). Significant differences between Foxp2-V1 and CB+ V1 synapses after post hoc Bonferroni tests are indicated (*p<0.05; ****p<0.0001). Synapses from Foxp2-V1 axons have higher density than CB+ V1 axons in HMC and LMC columns at all spinal segments except for L1/L2 LMC. MMC motoneurons receive similar synaptic densities from both types of V1 axons in upper lumbar regions, but Foxp2-V1 synapse predominate in lower lumbar. In S1 the density of CB+/V1 synapses is significantly higher. The low synaptic densities estimated in PGC neurons for Foxp2-V1s and CB+ V1s are not significantly different. Further details of statistical comparisons are in Supplementary file 1c and d. (F) Comparing the numbers of Foxp2 and CB+ (Renshaw) V1 synapses to the total number of V1 synapses, we estimated their respective percentages. From these estimates we calculated that the remainder belongs to non-Foxp2 and non-CB+ Renshaw cells. The large majority of V1 synapses on the cell bodies of LMC, HMC, and MMC motoneurons are either from Renshaw cells or Foxp2-V1s. (G) Summary diagram of major V1 clade connectivity to motoneuron cell bodies. Foxp2-V1s and Renshaw cells form the majority of inhibitory V1 contacts on LMC and HMC motoneurons, with slightly higher density from Foxp2-V1s. The MMC receives roughly equal portions of V1 contacts from Foxp2-V1s and Renshaw cells. V1s provide only sparse inhibition on preganglionic sympathetic neurons and most originate in V1 clades other than Renshaw cells and Foxp2-V1s.
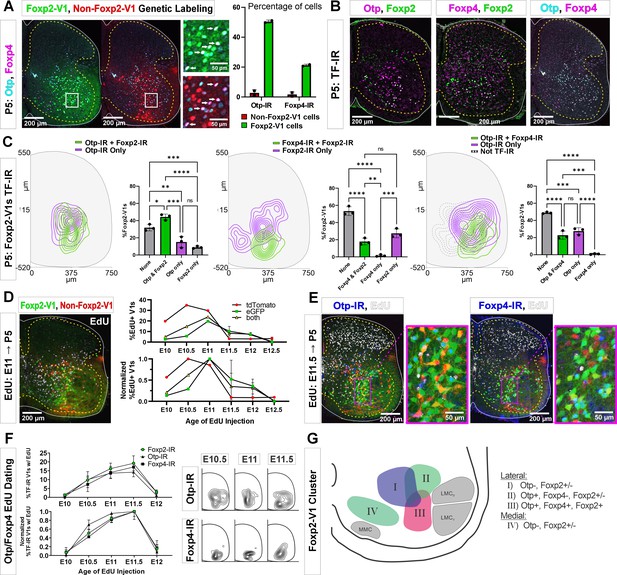
Subgroups of Foxp2-V1 interneurons defined by transcription factor (TF) expression at P5 and birthdate.
(A) Otp (blue) and Foxp4 (white) expression in lineage-labeled Foxp2-V1s (EGFP, green) and non-Foxp2-V1s (tdT, red). The boxed area is shown at higher magnification with different color combinations for clarity. It shows that Foxp4-IR cells in the Foxp2-V1 population always expressed Otp (arrows). Quantification: 49.8–51.2% of Foxp2-V1s express Otp and 20.7–21.7% Foxp4 (n=2 mice each examined in 3 ventral horns in L4/5). Very few non-Foxp2-V1 cells express either TF (Otp: 1.3–4.2% and Foxp2: 0.5–2.9%). (B) Images of P5 spinal cords containing Foxp2-V1 lineage labeling (EGFP, omitted for clarity) and double immunolabeled for Otp/Foxp2, Foxp4/Foxp2, and Otp/Foxp4. (C) Quantification of Foxp2-V1 interneurons with different combinations of TF expression at P5. For each combination, the left panels show cell distributions, and the right graphs the percentage of Foxp2-V1s with each combination (n=3 mice each analyzed in 6 ventral horns). The data was analyzed with one-way ANOVAs followed by Bonferroni-corrected pairwise comparisons (statistical details in Supplementary file 1e, f, and g. ****p<0.0001; ***p<0.001; **p<0.01; *p<0.05). There are five main groups defined by TF expression patterns: Otp-Foxp2 (44%), Otp-Foxp2-Foxp4 (23%), Otp only (15%), Foxp2 only (9%), and no TF labeling (32% by subtracting all other groups). Some groups associate with specific locations: Otp-Foxp2 cells and the smaller proportion of Otp-only cells are located laterally; Otp-Foxp4-Foxp2 cells are located lateroventrally; medial cells either contain only Foxp2 or nothing; some dorsal cells are either Foxp2 only or do not express any of these TFs. (D) Example image of 5-ethynyl-2'-deoxyuridine (EdU) birthdating in En1Cre, Foxp2flpo, R26 RCE:dualGFP/Ai9 lsl-tdT dual-color mice pulse labeled at E11. Foxp2-V1s (green and yellow cells) are born between E10 and E12, with peak birthdate around E11 and after non-Foxp2 V1s (tdTomato only). The lower graph’s data is normalized to highlight the time of peak neurogenesis for each population (n=15.0 ± 4.1 ventral horns from one mouse per time point from E10 to E11 and 2 mice per time from E11.5 to E12.5; total 9 mice; bars show SEM). There is no difference between green (EGFP only) and the smaller population of yellow cells (EGFP and tdTomato). (E) EdU labeling in the Foxp2-V1 dual-color genetic model combined with Otp and Foxp4 antibody staining. (F) V1s expressing Foxp2, Otp, and Foxp4 at P5 are mostly born between E10.5 and E11.5 with neurogenesis time courses largely overlapping. The normalized plot indicates that peak neurogenesis for all three populations occurs at E11.5, although a marginally higher number of Otp V1 cells are born earlier (n=4 ventral horns from 3.27±1.34 mice per time point; error bars show SEM). Contour plots to the right show settling locations of Otp and Foxp4-IR populations born at each time point. (G) Schematic of the L4-5 ventral horn summarizing Foxp2-V1 subgroups according to location and combinatorial expression of Otp, Foxp4, and Foxp2 at P5. LMCD: dorsal lateral motor column; LMCV: ventral lateral motor column; MMC: medial motor column.
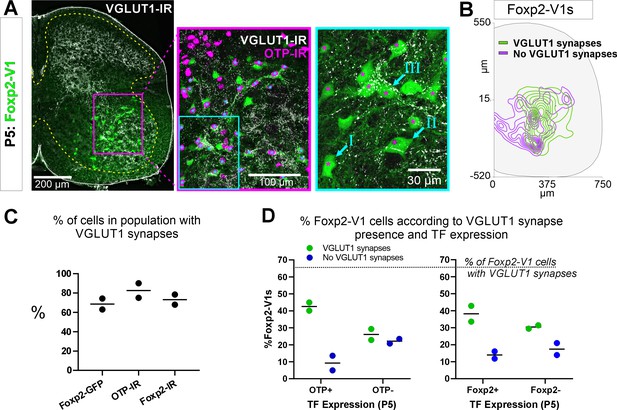
Proprioceptive (VGLUT1+) inputs preferentially target lateral Foxp2-V1 interneurons.
(A) Left, low-magnification confocal image of Foxp2-V1 lineage labeling (EGFP) combined with VGLUT1 antibody staining to identify primary proprioceptive afferent synapses. Center, the boxed area is expanded and shown with the magenta OTP-IR channel overlaying the GFP channel instead of merging with the green to maximize visual discrimination of OTP-IR Foxp2-V1s (denoted by asterisks). The overlay method used here is fully described in Materials and methods, ‘Figure composition’. Right, the center image’s inlay is shown at high magnification to demonstrate variability of VGLUT1 synapse density on Foxp2-V1 interneurons (I=absent, II = medium or low, III = high). For simplicity and rigor, we classified Foxp2-V1 interneurons as receiving or not receiving VGLUT1 synapses. Asterisks here indicate which of these Foxp2-V1s are OTP-IR based on the lower magnification images. OTP-IR Foxp2-V1s are laterally biased in their positioning and tend to have higher VGLUT1 synapse densities. (B) Distribution of Foxp2-V1 interneurons with and without VGLUT1 synapses (green and magenta, respectively). The positioning of Foxp2-V1 interneurons receiving VGLUT1 synapses is laterally biased. (C) Percentages of Foxp2-V1s (GFP+ interneurons), OTP-IR Foxp2-V1s, and Foxp2-IR Foxp2-V1s receiving VGLUT1 synapses (both proximal and/or distal). Each dot is an animal estimate from 6 or 7 ventral horns with respectively 591 and 525 Foxp2-V1 interneurons sampled. Lines indicate the averages of both animals. (D) Percentages of lineage-labeled Foxp2-V1 interneurons receiving VGLUT1 synapses and with Otp expression (left graph) or Foxp2 (right graph). Each dot represents one mouse, and the lines indicate averages. The numbers of sections and genetically labeled Foxp2-V1 interneurons sampled in each mouse are as in C. In each mouse, this includes 237 and 236 Otp-IR cells and 256 and 225 Foxp2-IR cells.
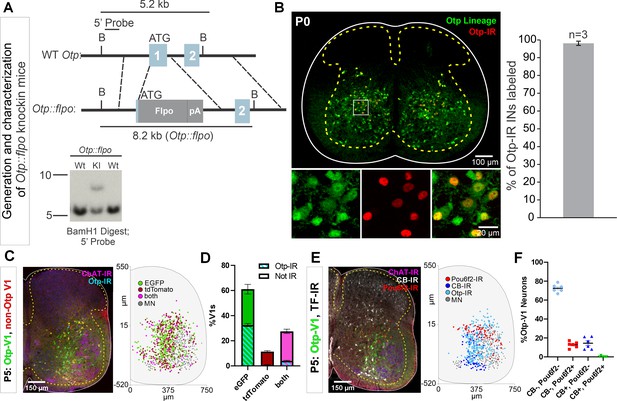
Generation of Otp-Flpo mice and intersection with En1-cre mice to target Otp-V1 cells.
(A) Targeting strategy to generate Otp::flpo mice. Flpo was inserted into the ATG in the first exon of the Otp genomic locus. Dotted lines represent approximate regions of homology in the targeting vector. Southern blot (bottom) of BamH1-digested genomic DNA with a 5’ probe external to the targeting vector identifies a 5.2 kb wild-type fragment, and an 8.2 kb knock-in fragment. Not shown: Deletion of selectable neomycin resistance gene flanked by loxP sites by crossing to Protamine-Cre mice, which recombines the floxed PGK-Neo cassette in the male germline. (B) Left, P0 lumbar spinal cord of Otp::flpo, R26RCE.fsf-GFP mice, demonstrating expression in Otp-IR interneurons. Right, 98.1 ± 1.2% (mean ± SEM, n=3 mice) of Otp-expressing cells are labeled by the reporter. (C) Intersection of Otpflpo/+, En1cre/+ using the dual-color strategy with simultaneous expression of the Ai9 tdT and RCE-DC EGFP reporters. V1 cells that express otp are labeled with EGFP (green). In these cells the Ai9-tdT reporter is effectively deleted by Flpo recombination dependent on the level of Otp expression: strong (EGFP only) and weak (EGFP and tdT). V1 cells that express tdT only (red) never express Otp. In addition, the sections were labeled with antibodies for Otp (light blue) to reveal cells that retained expression of Otp at P5 and choline acetyltransferase (ChAT) (deep blue) to localize the motor pools. Most V1s express EGFP, with tdT-only cells being a minority. Right, cell plot positions of some of the cell types identified in these sections (one mouse 6 ventral horns in L4/L5). Most cells are Otp-V1s and are shown here as green (EGFP only) and pink dots (EGFP and tdT). (D) Quantification of cells with EGFP only (green), tdT only (red), or both (pink) (n=12 ventral horns from 2 mice; error bars show SD). 88.6% of V1s (1278 V1 cells analyzed in total) expressed EGFP; 60.8% were EGFP only, 27.8% were ‘yellow’ and surprisingly only 11.4% were tdT only. As expected, P5 Otp expression detected with antibodies was absent in most tdT-labeled V1 cells (93.5% of cells) and ‘yellow’ tdT+eGFP V1 cells (85.4%), but also in a significant proportion of EGFP-labeled V1 cells (46.7%). (E) V1 cells transiently expressing Otp in embryo included cells of other clades. This was examined in En1cre/+, Otpflpo/+, R26RCE:dual-eGFP mice. Detection of Pou6f2, calbindin, and Otp in genetically labeled Otp-V1 cells at P5. Left confocal image and right cell plot (n=6 ventral horns from 1 mouse). Pou6f2-Otp-V1 cells concentrate in a dorsal band within the ventral horn. Calbindin-IR Otp1-V1 cells concentrate in the Renshaw region (ventral most region) and others are in the dorsal region of the ventral horn. Otp-V1 cells retaining Otp expression at P5 occupy all dorsoventral positions in the lateral spinal cord. (F) Pou6f2 or calbindin immunoreactivity (-IR) in Otp-V1 cells (n=397) in 6 sections (each dot) in L4/L5 from one animal. Pou6f2 was detected in 12.8% of Otp-V1 cells at P5. In addition, 14.4% of Otp-V1 cells were calbindin+ and this included many in the Renshaw cell ventral region and few others located more dorsally. One rare dorsal Otp-V1 cell contained both Pou6f2 and calbindin (included in both percentages above). By limiting the analysis to ventral Otp-V1 interneurons in the Renshaw area we estimated that 8.3% of them are Renshaw cells. In conclusion, the Otp-V1 lineage includes cells from several V1 clades. Many downregulate Otp expression before birth, thus at P5 all Otp-expressing cells are restricted to the Foxp2-V1 lineage. In addition, many medial non-V1 Foxp2 cells also express Otp. Therefore, to specifically target the lateral group of proprioceptive Otp-Foxp2-V1 cells tightly associated to the LMC, a triple genetic intersection or alternatively, postnatally timed Otp-dependent recombination is necessary. Raw images of the blots and corresponding labeling are found in Figure 7—figure supplement 1—source data 1 and Figure 7—figure supplement 1—source data 2.
-
Figure 7—figure supplement 1—source data 1
Raw image of gel corresponding to Figure 7—figure supplement 1A.
- https://cdn.elifesciences.org/articles/95172/elife-95172-fig7-figsupp1-data1-v1.pdf
-
Figure 7—figure supplement 1—source data 2
Annotated image of gel corresponding to Figure 7—figure supplement 1A.
- https://cdn.elifesciences.org/articles/95172/elife-95172-fig7-figsupp1-data2-v1.pdf
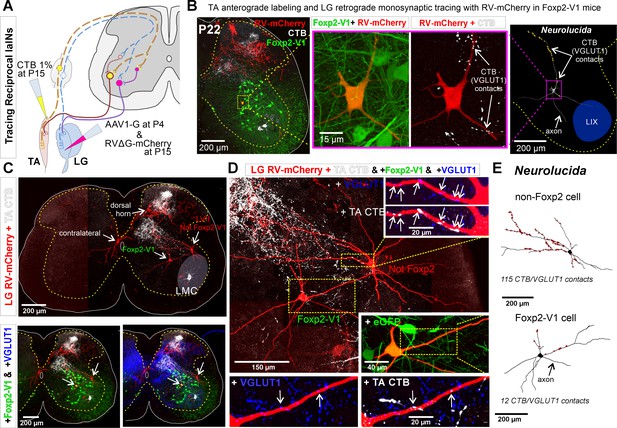
Some Foxp2-V1 interneurons are reciprocal Ia inhibitory interneurons (IaINs).
(A) Experimental design to label spinal neurons that receive inputs from tibialis anterior (TA) muscle primary afferents and connect to lateral gastrocnemius (LG) motoneurons, forming Ia reciprocal inhibitory connections from TA to LG. TA sensory afferents are labeled anterogradely with cholera toxin subunit B (CTB) followed by antibody detection of CTB and the presynaptic marker VGLUT1. Interneurons premotor to LG motoneurons are labeled by monosynaptic retrograde labeling with RVΔG-mCherry. (B) Foxp2-V1 IaIN with the most TA/VGLUT1 contacts (31) in our sample (n=5). Left, low-magnification image of Foxp2-V1 interneurons (EGFP, green), RV-mCherry labeling (red) of LG muscle afferents in the dorsal horn and interneurons presynaptic to the LG and of TA afferents anterogradely labeled with CTB (white). The Foxp2-V1 interneuron contains mCherry (yellow cell, inside box). This cell is magnified in two panels to the right, one showing Foxp2-V1 and RV-mCherry and the other RV-mCherry and CTB labeling. Arrows in the zoomed image show examples of CTB synapses (confirmed with VGLUT1) on the dendrites of this neuron. Far right image is the 3D reconstructed cell (Neurolucida) with CTB/VGLUT1 synapses indicated on its dendrites by yellow stars. The axon initial trajectory is indicated (the axon is lost at the section cut surface). The blue area highlights lamina IX. (C) Low magnification of section serial to B, showing TA-CTB afferents (white) and LG-RV-mCherry-labeled interneurons (red). Transsynaptically labeled interneurons are categorized according to position and Foxp2-V1 lineage labeling: images below show superimposed Foxp2-V1 EGFP (green) and additional VGLUT1 immunolabeling (blue). The location of the LMC is indicated. Contralateral interneurons were found in LX (as the one in this section) and in LVIII (the other two in this animal, not shown). (D) High-magnification images of two LVII LG-coupled interneurons (RV-mCherry, red) receiving synapses from TA afferents (dendrites in boxed regions are shown at high magnification demonstrating CTB-TA labeling and VGLUT1 content). The most medial interneuron belongs to the Foxp2-V1 lineage (see inset with +EGFP). Insets shown VGLUT1 synapses with (arrows) and without CTB from the TA muscle. (E) Neurolucida neuronal reconstructions showed that the Foxp2-V1 interneuron contained a medium number of TA/VGLUT1 synapses (13 contacts) in our sample of putative IaINs derived from the Foxp2-V1 lineage (n=5), while the non-Foxp2-V1 interneuron contained the largest (115) of any LVII reconstructed interneuron with mCherry, including many proximal synapses. For further examples of labeling in serial sections from this animal, see Figure 8—figure supplement 1.
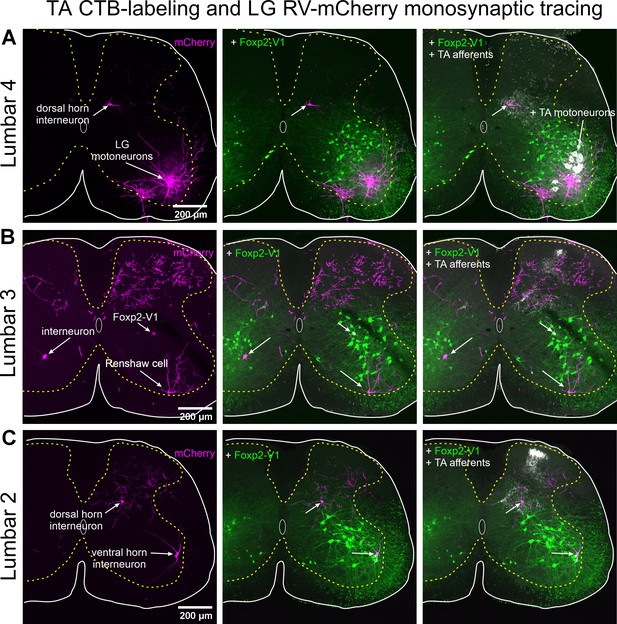
Labeling of motoneurons and interneurons, but only limited labeling of primary afferents with RVΔG-mCherry.
All confocal images are serial to those shown in Figure 8. (A) Left panel. Lumbar 4 section showing RVΔG-mCherry labeling in lateral gastrocnemius (LG) motoneurons and one dorsal horn interneuron in medial LV. Many dendrites, motor axons, and collaterals located in the Renshaw cell area are labeled. There are no mCherry-labeled primary afferents in the dorsal root, dorsal columns, or dorsal horn in this segment (the same for Lumbar 5). Middle panel. Added Foxp2-V2 lineage labeling (EGFP). The dorsal horn interneuron is far from the ventral horn region containing Foxp2-V1 interneurons. Right panel. Added cholera toxin subunit B (CTB) labeling injected in the TA. TA motoneurons and TA primary afferents are respectively retrogradely and anterogradely labeled. TA afferents concentrate in medial lamina V and contact the dorsal horn mCherry interneuron monosynaptically connected to the LG motor pool. (B) Left panel. Lumbar 3 section showing extensive RVΔG-mCherry axon labeling in the dorsal horn and three different types of ventral horn interneurons monosynaptically connected to the LG motor pool. This lumbar segment contained the denser dorsal horn axon labeling found in this animal. Some of these axons may arise from primary afferents since they can be traced to the dorsal column. These axonal arbors are far from ventral horn interneurons and have no intermingled mCherry-labeled interneurons. The three mCherry lumbar interneurons labeled in this section are located in different ventral horn positions: contralateral LVII, ipsilateral Renshaw cell area, and ipsilateral middle of LVII. Middle panel. Added Foxp2-V2 lineage labeling (EGFP). Interneurons located in the contralateral spinal cord and in the ipsilateral Renshaw cell area are not Foxp2-V1. The cell in the middle of LVII is a Foxp2-V1 interneuron. Right panel. Added CTB labeling injected in the TA. Only TA afferents are visible in this segment. They occupy medial LV as in Lumbar 4 (A). TA afferents also project to the superficial laminae in this segment. (C) Left panel. Lumbar 2 section showing RVΔG-mCherry labeling of dorsal and ventral horn interneurons. This lumbar segment shows some axon labeling in the dorsal horn. Some may arise from primary afferents. Middle panel. Added Foxp2-V2 lineage labeling (EGFP). mCherry-labeled interneurons in this section are not Foxp2-V1 interneurons. Right panel. Added CTB labeling injected in the TA. Only TA afferents are visible in this segment. Like the Lumbar 3 segment (B), TA afferents project to medial lamina V and also to superficial laminae. TA afferents contact the dorsal horn mCherry interneuron in medial lamina V.
Tables
Mouse models.
Mouse | MGI # | RRID # | Brief description | Donating laboratory | Reference |
---|---|---|---|---|---|
En1::cre | MGI:3029756 | En1-cre KI/KO | Goulding (Salk Inst.) | Sapir et al., 2004 | |
Foxp2::Flpo | MGI:6728072 | Foxp2-Flpo KI/KO | Bikoff/Jessell (Columbia U.) | Bikoff et al., 2016 | |
Otp::Flpo | MGI:6728073 | Otp-Flpo KI/KO | Bikoff/Jessell (Columbia U.) | This manuscript | |
MafB::GFP | MGI:6145008 | RRID:IMSR_RBRC02220 | MafB-GFP KI/KO | Takahashi (Tsukuba U.) | Moriguchi et al., 2006 |
Ai9-tdT | MGI:3813511 | RRID:IMSR_JAX:007909 | Rosa26-lsl-tdTom | The Jackson Laboratory | Madisen et al., 2010 |
RCE:dual-EGFP | MGI:4420759 | RRID:MMRRC_032036-JAX | Rosa26-lsl-fsf-EGFP | The Jackson Laboratory | Sousa et al., 2009 |
RC::FLTG | MGI:5617960 | RRID:IMSR_JAX:026932 | Rosa26-FLTG | The Jackson Laboratory | Plummer et al., 2015 |
RCE:FRT | MGI:4420764 | RRID:MMRRC_032038-JAX | RCE-fsf-GFP | Fishell (Harvard) | Sousa et al., 2009 |
Antibodies.
Antigen | Immunogen | Host/type | Manufacturer | Catalog # | RRID # | Dilution |
---|---|---|---|---|---|---|
OTP | Recombinant protein [DPGGHPGDLAPNSDPVEGATC] | Guinea Pig, polyclonal | Jessell Lab/ HHMI CU | CU1497 | RRID:AB_2665423 | 1:3000 |
OTP | Recombinant protein (aa1–130 from human OTP) | Rabbit, polyclonal | Thermo Fisher | PA5-89060 | RRID:AB_2805328 | 1:2000 |
Pou6f2 | Recombinant protein (Pou6f2) | Rabbit, polyclonal | Sigma | hpa008699 | RRID:AB_1079664 | 1:1000 |
Pou6f2 | Synthetic peptide amino acids 35–184 of human Pou6f2 | Guinea Pig, polyclonal | Jessell Lab/ HHMI CU | RRID:AB_2665423 | 1:500 | |
Pou6f2 | Synthetic peptide amino acids 35–184 of human Pou6f2 | Rat, monoclonal | Jessell Lab/ HHMI CU | RRID:AB_2665427 | 1:1000 | |
Sp8 | Synthetic peptide (C-terminus [C-18] from human Sp8) | Goat, polyclonal | Santa Cruz | sc-104661 | RRID:AB_2194626 | 1:2000 |
Foxp2 | Synthetic peptide (N-terminus [N-16] from human FOXP2) | Goat, polyclonal | Santa Cruz | sc-21069 | RRID:AB_2107124 | 1:2000 ICC and WB |
Foxp4 | Recombinant protein [DPGGHPGDLAPNSDPVEGATC] | Rabbit, polyclonal | Jessell Lab/ HHMI CU | CU1464 | RRID:AB_2665415 | 1:25000 |
MafA | Recombinant protein (aa300–359 [C-terminus] from mouse v-mafA) | Rabbit, polyclonal | Novus | NB400-137 | RRID:AB_10002142 | 1:500 WB |
MafB | Recombinant protein (Transcription factor MafB) | Rabbit, polyclonal | Sigma | hpa005653 | RRID:AB_1079293 | 1:1000 ICC and WB |
MafB | Recombinant protein (aa100–150 from mouse MafB) | Rabbit, polyclonal | Novus | NB600-266 | RRID:AB_2137664 | 1:250 ICC 1:500 WB |
c-Maf | Recombinant protein (aa150–200 from mouse c-Maf) | Rabbit, polyclonal | Novus | NB600-267 | RRID:AB_2137514 | 1:500 WB |
NeuroD2 | Synthetic peptide Mouse NeuroD2 aa1–100 conjugated to KLH | Rabbit, polyclonal | AbCam | Ab104430 | RRID:AB_10975628 | 1:2000 |
Prox1 | Synthetic peptide from the C-terminus of mouse Prox1 | Rabbit, polyclonal | Millipore Sigma | AB5475 | RRID:AB_177485 | 1:500 |
RFP | Recombinant protein (RFP from Discosoma sp.) | Mouse, monoclonal | Rockland | 200-301-379 | RRID:AB_2611063 | 1:1000 |
dsRed | Recombinant protein (RFP variant from Discosoma sp.) | Rabbit, polyclonal | Clontech | 632496 | RRID:AB_10013483 | 1:1000 |
GFP | Recombinant protein (GFP from Aequorea victoria) | Chicken, polyclonal | Aves | GFP-1020 | RRID:AB_10000240 | 1:1000 |
ChAT | Human placental enzyme | Goat, polyclonal | EMD Millipore | AB144P | RRID:AB_2079751 | 1:100 |
NeuN | Synthetic peptide of human RBFOX3/NeuN protein (aa20–100) | Rabbit, polyclonal | Novus | NBP1-77686 | RRID:AB_11009597 | 1:1000 |
NeuN | Isolated brain cell nuclei | Mouse clone A60 | Millipore | MAB377 | RRID:AB_2298772 | 1:100 |
Calbindin | Recombinant protein (calbindin D-28k from rat) | Rabbit, polyclonal | Swant | CB-38a | RRID:AB_10000340 | 1:1000 |
VGLUT1 | Synthetic peptide (aa456–560 from rat VGLUT1) | Guinea Pig/ polyclonal | Synaptic Systems | 135-304 | RRID:AB_887878 | 1:1000 |
VGAT | Synthetic peptide (N-terminus from rat VGAT) | Mouse/ monoclonal | Synaptic Systems | 131-011 | RRID:AB_887872 | 1:100 |
VGAT | Recombinant protein (N-terminus from rat VGAT) | Guinea Pig/ polyclonal | Synaptic Systems | 131-004 | RRID:AB_887873 | 1:200 |
CTB | Cholera toxin B subunit | Goat/ polyclonal | List Labs | #703 | RRID:AB_10013220 | 1:200 |
acetyl-Histone H3 | Linear peptide from human Histone H3 acetylated at the N-terminus | Rabbit/ polyclonal | EMD Millipore | 06-599 | RRID:AB_2115283 | 1:1000 |
Cell lines.
Cell line | Brief description/validation | Donating laboratory | Reference |
---|---|---|---|
B7GG | B7GG cells express T7 RNA polymerase, rabies virus G, and histone-tagged GFP. Their origin is BHK-21 cells. They express GFP when expressing RV-G. Cell producing virus have additionally mCherry in the cytoplasm. | Dr. Edward Callaway (Salk Institute, La Jolla, CA, USA) | Osakada and Callaway, 2013 |
HEK 293T-TVA800 cells | HEK 293T-TVA800 cells are derived from HEK 293T cells and express TVA. These cells are used to titer pseudotyped G-deleted rabies viruses. | Dr. Edward Callaway (Salk Institute, La Jolla, CA, USA) | Osakada and Callaway, 2013 |
Additional files
-
Supplementary file 1
Statistical figures for the data presented.
Contains all the statistical tables for graphs shown in figures. 1a is related to Figure 4D; 1b is related to Figure 5D; 1c is related to Figure 5E, top graph; 1d is related to Figure 5E, bottom graph; 1e is related to Figure 6C, left graph Otp/Foxp2; 1f is related to Figure 6C, center graph Foxp4/Foxp2; 1g is related to Figure 6C, right graph Otp/Foxp4.
- https://cdn.elifesciences.org/articles/95172/elife-95172-supp1-v1.docx
-
MDAR checklist
- https://cdn.elifesciences.org/articles/95172/elife-95172-mdarchecklist1-v1.pdf