High-resolution awake mouse fMRI at 14 tesla
Figures
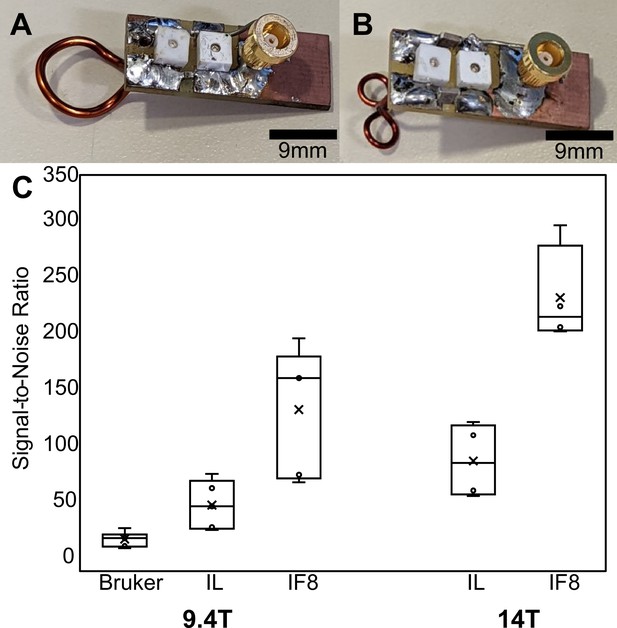
Comparison between implanted and commercial coils.
(A and B) show representative (unattached) prototype coils in the single loop and ‘figure 8’ styles, respectively. (C) Box-and-whisker plot presents the cortical-specific signal-to-noise ratio (SNR) values calculated by dividing the mean signal of the upper cortex by the standard deviation of the noise to compare between commercial Bruker phased array surface coil, single loop implant, and ‘figure 8’ style implants. Bruker → commercial phased array coil, IL → implanted single loop coil, IF8 → implanted ‘figure 8’ coil. The bar graph shows the SNR of anatomical images acquired with different radio frequency (RF) coils using the 9.4 T scanner ( = 27.2, N=6, = 57.5, N=5, = 142.5, N=5) and the 14 T scanner ( = 96.8, N=4, = 209.2, N=5).
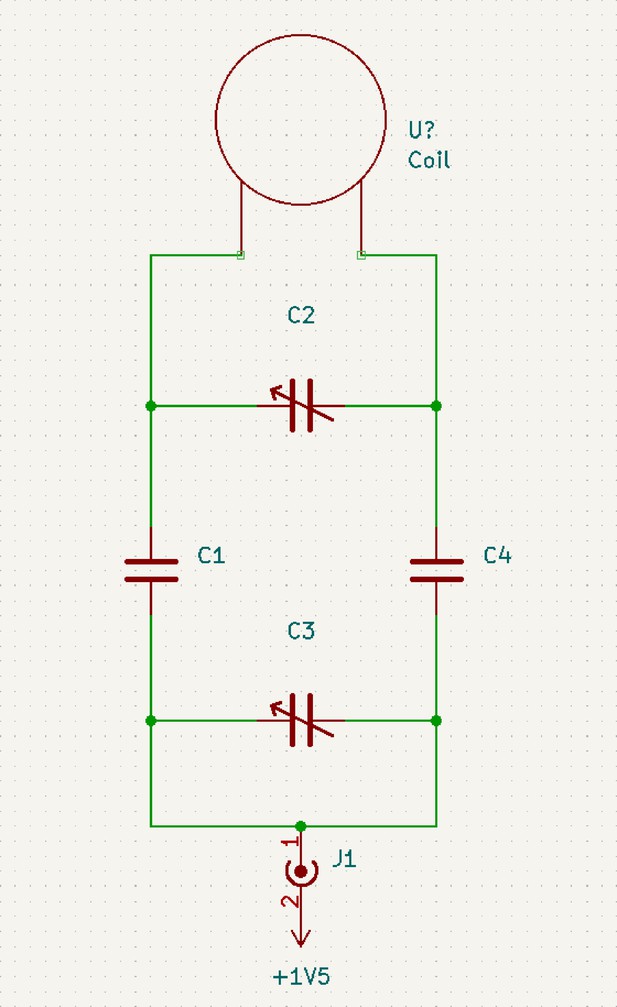
Schematic of circuit diagram of coil designed for 1H imaging at 600 MHz.
C1 and C4 are 2.2 pF capacitors. C2 is a 0.6–2.5 pF trimmer. C3 is a 5–18 pF trimmer.
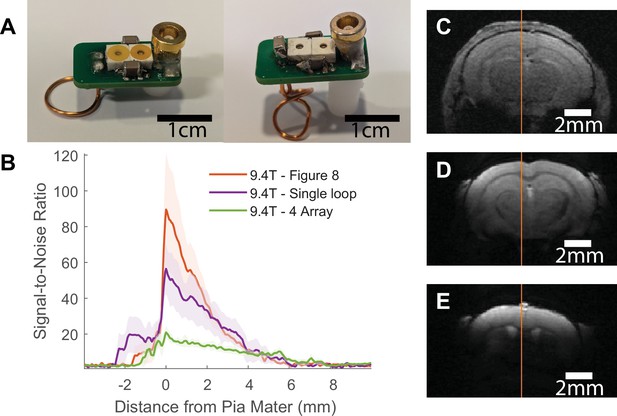
Comparison between implanted and commercial coils.
(A) shows representative (unimplanted) coils in the single loop (left) and ‘figure 8’ styles (right). Table 2 provides a parts list and cost for making these coils and Figure 1—figure supplement 1 provides a circuit diagram to assemble. (B) presents the signal-to-noise ratio (SNR) line profile values as a function of distance from pia mater for each coil tested at 9.4 T: commercial phased array surface coil (four array), implanted single loop, and implanted ‘figure 8’. SNR values were calculated by dividing the signal by the standard deviation of the noise. Shaded regions represent standard error. (C–E) show a representative fast low angle shot (FLASH) image with line profile of SNR measurements from each of the coils used to create the graph seen in (B). Clear visual improvement in SNR can be seen in figures (C–E) . C – commercial phased array. D – single loop at 9.4 T. E – ‘figure 8’ at 9.4 T (N4 array = 6, Nsingle loop = 5, Nfigure 8 = 5).
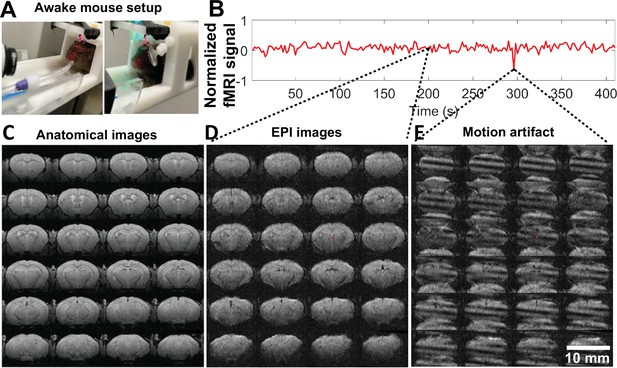
High-resolution awake mouse functional magnetic resonance imaging (fMRI) at 14 T.
(A). The awake mouse setup with head-fixed position in a custom-built cradle for visual and vibrissa stimulation. (B) The representative fMRI time course of an awake mouse based on raw image data acquired from high-resolution echo planar imaging (EPI), enabling the trace of motion-induced artifacts. (C) The anatomical MRI images (fast low angle shot [FLASH]) acquired from one representative awake mouse, showing minimal susceptibility and whole brain coverage from the implanted surface coil. (D) The raw EPI fMRI image with same spatial resolution as the anatomical FLASH image. (E) The snapshot of the distorted images due to motion of the awake mouse during scanning. Video 2 shows the video of motion-induced artifacts throughout the fMRI trial.
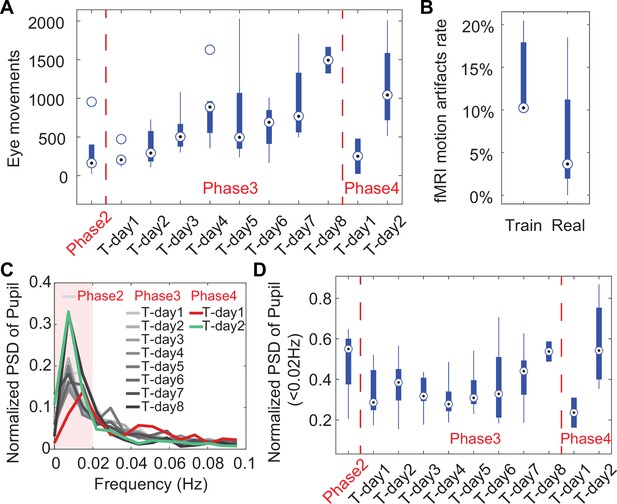
Results of acclimation training on animals.
(A) Box-and-whisker plot showing eye movements over 10 min duration of training days showing an increase in eye movements over the training duration indicating the animals are becoming more relaxed in the holder while being exposed to MRI sounds. The drop in eye movements at Phase 4 Training Day 1 is caused by putting the animals into the MRI for the final training. The following day at Phase 4 Training Day 2, the eye movements have increased back to the level before being inserted into the scanner. Phase 2 incorporated the 2 days animals were put in the holder without the mock-MRI environment. Phase 3 includes each day of training in a mock-MRI environment. Phase 4 showcases eye movements acquired inside the MRI during resting-state data acquisition. (B) Box-and-whisker plot of the ratio of detectable motion during scanning +1 or 2 days after training (train) and after 1 month of scanning (real) showing a non-significant decrease in the detectable motion indicating the effectiveness of the training. (C) Graph of the normalized power spectrum density (PSD) showing the increase of low-frequency pupil oscillations from eye in each phase. (D) Box-and-whisker plot showing the averaged normalized PSD of pupil oscillation frequencies below 0.02 Hz as maximally indicated in (C) showing late stage increases in mock-MRI and EPI-MRI phases, e.g., Phase 3 and Phase 4. Circle with black dot represents the median of each data group.
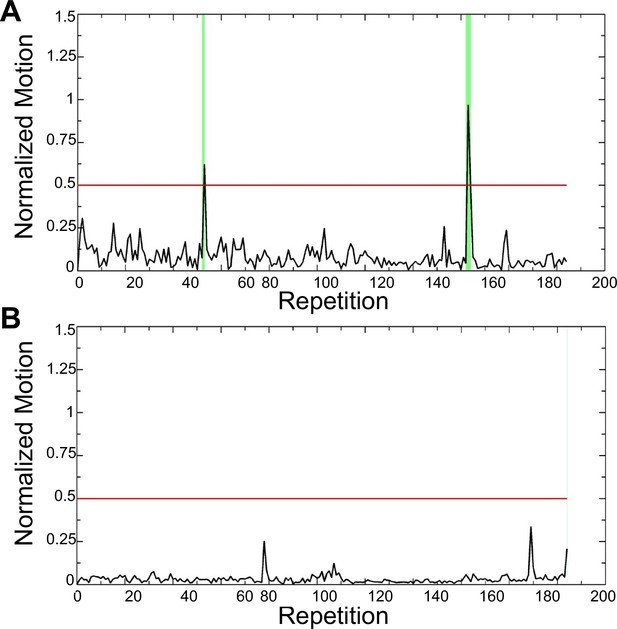
Figure showing motion data (.MOT file) of a representative mouse immediately following training (A) and after 1 month of scanning (B) indicating the amount of struggling the animal is doing during scanning.
Struggling-induced spikes are already substantially minimized following the training with only 5 volumes (2%) censored. After 1 month of scanning there was only one single repetition (repetition 204) at the end of the scan that was censored.
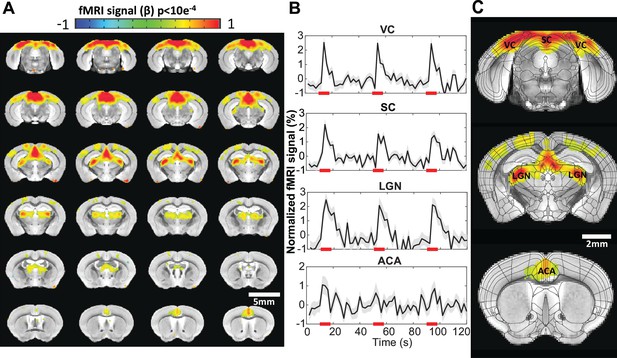
Visual stimulation-evoked high-resolution functional magnetic resonance imaging (fMRI) of awake mice.
(A) The brain-wide functional maps of awake mice show strong positive blood oxygen level-dependent (BOLD) activation in the visual cortex (VC), lateral geniculate nucleus (LGN), superior colliculus (SC), and anterior cingulate area (ACA) based on the group analysis. Highlighted brain regions are statistically generated using a one-way T-test with p<0.0001 (B) The averaged time course of the ROIs derived from the Allen Brain Atlas, demonstrating an evoked positive BOLD signal changes upon the 8 s visual stimulation (5 Hz 530 nm and 5.1 Hz 490 nm 20 ms light pluses). Each graph displays the average of 162 sets of 3 stimulation epochs. Shaded regions represent standard error. Red lines represent the 8 s stimulation duration. (C) Functional maps overlain with the brain atlas to highlight the activated brains regions: VC, SC, LGN, and ACA (N=13 (6F/7M)).
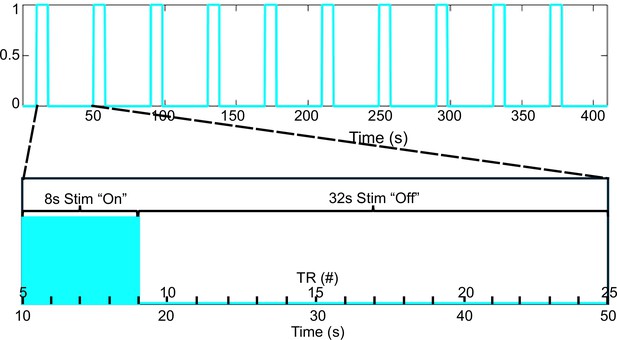
Figure showing stimulation paradigm in blue.
Each ‘TR period’ is 2 s. The stimulation is repeated 10 times over the 410 s acquisition period which includes a 10 s baseline acquisition period before the first stimulation. This paradigm results in 4 ‘TR periods’ occurring during the stim on phase and 16 ‘TR periods’ occurring during the stim off phase. Any stimulation design can be used for the ‘on’ duration. TR, repetition time.
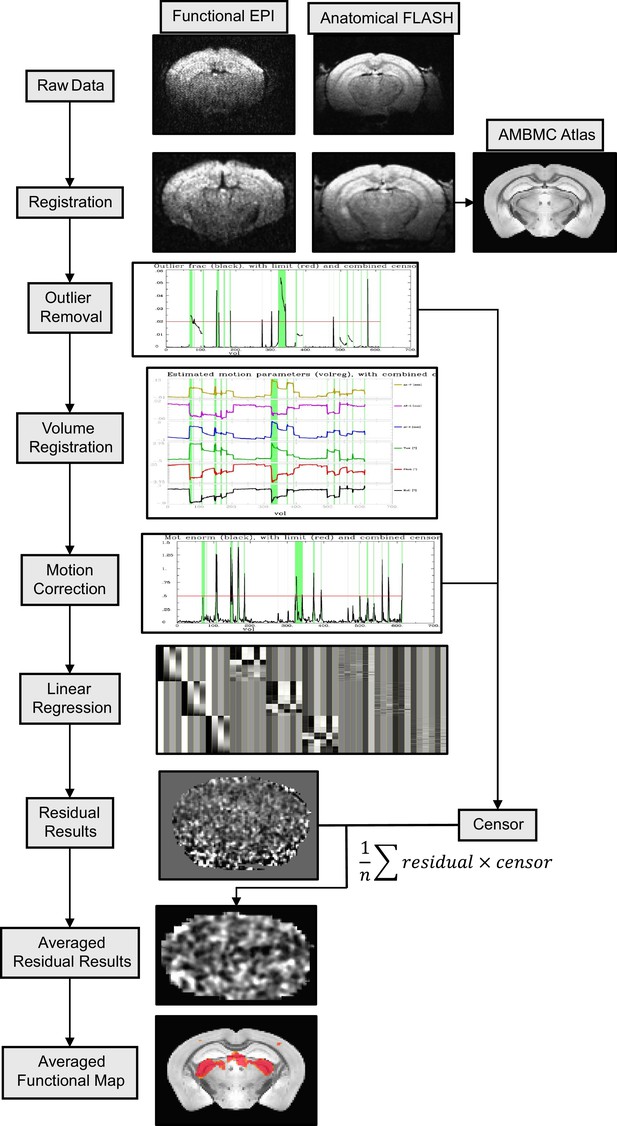
The processing pipeline of the awake mouse functional magnetic resonance imaging (fMRI) datasets.
The workflow diagram is described through the following steps: raw data registration, outlier removal, volume registration-based motion file estimation, motion removal, linear regression with the censoring function, functional map demonstration.
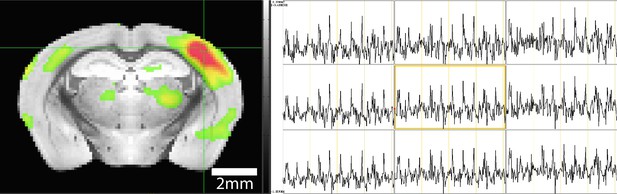
Time course from single experiment showing strong blood oxygen level-dependent (BOLD) activations during stimulation paradigm.
(Left) BOLD activation map highlighting positive activation in the barrel cortex (BC) and selection of a 3×3 voxel display of time course data (green box). (Right) Time course data taken from 3×3 voxel selection clearly showing 10 stimulations in each of the voxels shown.
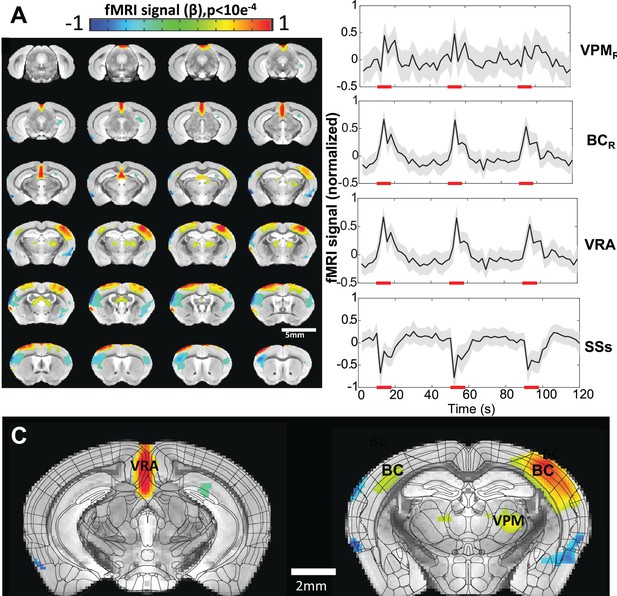
Vibrissa stimulation-evoked high-resolution functional magnetic resonance imaging (fMRI) of awake mice.
(A) The brain-wide functional maps of awake mice show the strong positive blood oxygen level-dependent (BOLD) activation in the contralateral barrel cortex (BC) and ventral posteromedial nucleus (VPM) and posterior thalamic nucleus (PO). Positive BOLD signals are also detected at the motor cortex (MC) and the ventral retrosplenial area (VRA), as well as at the ipsilateral BC and thalamic nuclei. Negative BOLD signals are detected in supplementary somatosensory areas (SSs) (including nose and mouth) as well as part of the caudoputamen. Highlighted brain regions are statistically generated using a one-way T-test with p<0.0001. (B) The averaged time course based on the brain atlas ROIs for VMP, BC, and VRA, demonstrating positive BOLD signal changes upon the 8 s air-puff vibrissa stimulation (8 Hz, 10 ms). Averaged time course of the SSs ROI shows negative BOLD signal changes. Each graph displays the average of 279 sets of 3 stimulation epochs. Shaded regions represent standard error. Red lines represent the 8 s stimulation duration. (C) The functional maps are overlain with the brain atlas to highlight the activated vibrissa thalamocortical pathway (VPM→BC) and the VRA in awake mice (N=13 (6F/7M)).
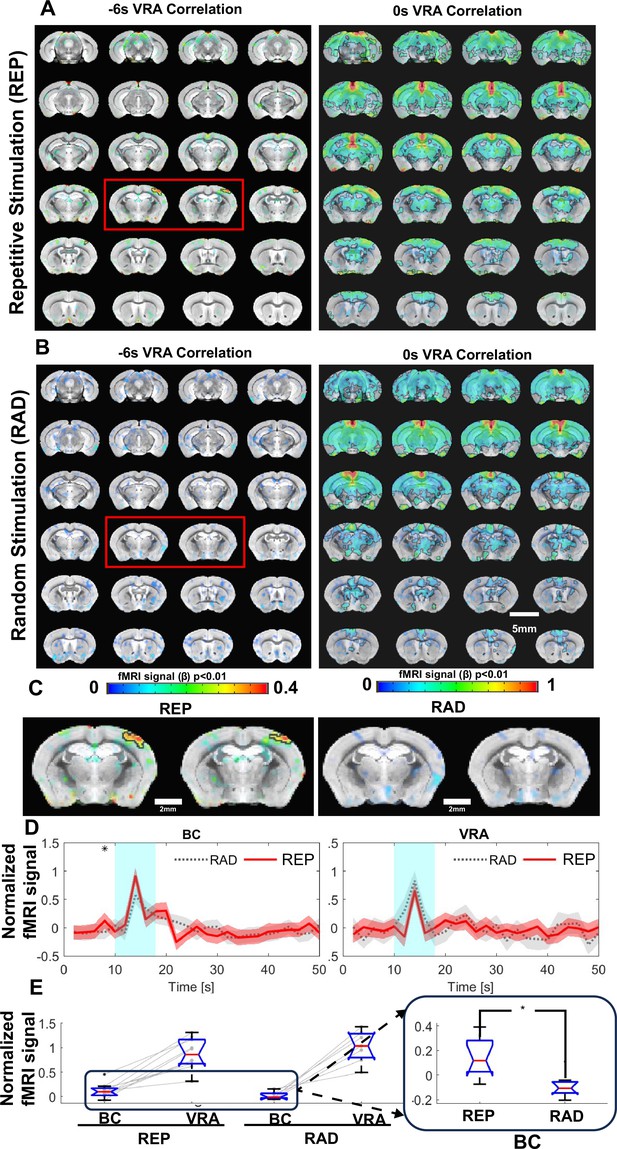
Ventral retrosplenial area (VRA)-based brain-wide correlation maps at different time shifts.
(A) The VRA-based correlation maps at –6 s and 0 s time shifts of awake mice with repetitive stimulation (REP). The strong correlation in the contralateral barrel cortex (BC) is shown in the correlation map at the –6 s time shift (red box). Highlighted brain regions are statistically generated using a one-way T-test with p<0.01. (B) The VRA-based correlation maps at –6 s and 0 s time shifts of awake mice with randomized stimulation (RAD). No correlation is detected in the contralateral BC at the –6 s time shift (red box). Highlighted brain regions are statistically generated using a one-way T-test with p<0.01 (C) The enlarged images from the –6 s time shift correlation maps of REP and RAD groups, demonstrating the strong correlation patterns located at the contralateral BC only in the REP group. (D) The averaged time course from both contralateral BC and VRA of REP and RAD groups, showing that early positive blood oxygen level-dependent (BOLD) signals detected at 2 s prior to the stimulation in contralateral BC of the REP group and no significant difference detected in VRA. Shaded regions represent standard error. ❋ shows significance from two-tail two-way T-test (p<0.05). (E) The bar graph presents the mean BOLD signals of contralateral BC at 2 s prior to stimulation time point and peak signals of VRA in REP and RAD groups. The inset is the expanded bar graph to show the significantly higher BOLD signals detected in the contralateral BC at 2 s prior to stimulation in REP group using a two-tail two-way T-test (p<0.015, REP graph displays the average of 930 stimulation epochs, RAD graph displays the average of 240 stimulation epochs). (N=9 (4F/5M)).
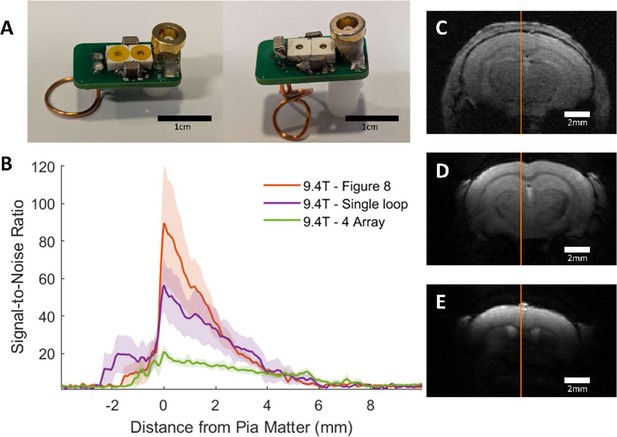
Comparison between implanted and commercial coils.
(A) shows representative coils in the single loop (left) and ‘figure 8’ styles (right). Supplementary Table 1 provides a parts list and cost for making these coils and Supplementary Figure 1 provides a circuit diagram to assemble. (B) presents the SNR line profile values as a function of distance from Pia Matter for each coil tested at 9.4T: commercial phased array surface coil (4 Array), implanted single loop coil, and implanted ‘figure 8’ coil. SNR values were calculated by dividing the signal by the standard deviation of the noise. (C-E) show a representative FLASH image with line profile of SNR measurements from each of the coils used to create the graph seen in B. Clear visual improvement in SNR can be seen in figures C-E. C – Commercial phased array. D – Single loop at 9.4T. E – Figure 8 at 9.4T. (N4 array = 6, Nsingle loop = 5, Nfigure 8 = 5)
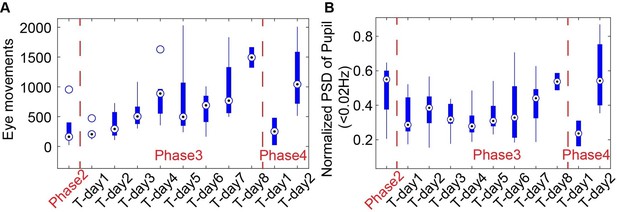
The eye movements (A) and power spectra of pupil dynamics (<0.02Hz) (B) change during different training phases.
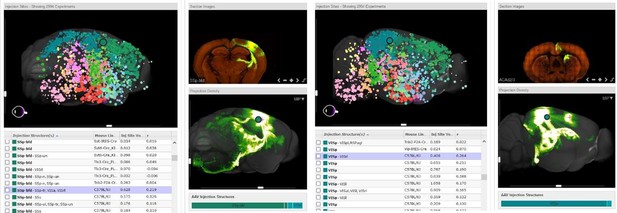
Representative images are shown indicating connections between the barrel cortex and retrosplenial area from an injection in the barrel cortex (Left panel) as well as the visual cortex and cingulate connection from an injection in the visual cortex (Right panel).
Images are of connectivity map projections from the Allen Brain Atlas derived from a Cre-dependent AAV tracing of axonal projections (Oh et al., 2014)
Videos
Video illustrating how animals are set up through the cradle using the implanted radio frequency (RF) coil for awake mouse imaging.
Video showing the real-time EPI raw images from awake mice.
The real-time tracer from the selected point demonstrates the time points with motion, as well as the motion-induced image distortion during awake mouse fMRI.
Tables
Table showing the training paradigm for each of the four phases of training.
rs → resting-state fMRI, stim → whisker stimulation fMRI.
Training day of phase | Phase 1(hold in hand) | Phase 2(holder+pupil) | Phase 3(mock-MRI+pupil) | Phase 4(EPI+pupil) |
---|---|---|---|---|
1 | 5 min | 15 min | 30 min | 60 min (rs) |
2 | 10 min | 30 min | 30 min | 60 min (rs) |
3 | 30 min | 60 min (stim) | ||
4 | 30 min | 60 min (stim) | ||
5 | 60 min | |||
6 | 60 min | |||
7 | 60 min | |||
8 | 60 min |
Parts list for construction of 200 1H coils configured for 600 MHz (14 T).
Part description | Cost |
---|---|
FR-4 PCB Chip, 0.4×0.8 in, 0.062 in thick | $214.00/200 |
Capacitor 2.2 pF 2KV | $309.80/400 |
Trimmer 0.6–2.5 pF | $1649.28/200 |
Trimmer 5–18 pF | $1640.56/200 |
Magnet wire 20AWG | $118.28/spool/710.8’ |
MCX socket | $1027.14/200 |
Entire coil built by MRIBOT | $300/each |
Training Day of Each Phase | Phase 1 (hold in hand) | Phase 2 (holder +pupil) | Phase 3 (Mock-MRI+pupil) | Phase 4 (EPI +pupil) |
---|---|---|---|---|
1 | 5 mins | 15 mins | 30 mins | 60 mins (rs) |
2 | 10 mins | 30 mins | 30 mins | 60 mins (rs) |
3 | 30 mins | 60 mins (stim) | ||
4 | 30 mins | 60 mins (stim) | ||
5 | 60 mins | |||
6 | 60 mins | |||
7 | 60 mins | |||
8 | 60 mins |