OsNF-YB7 inactivates OsGLK1 to inhibit chlorophyll biosynthesis in rice embryo
eLife assessment
This paper provides important insights into the role of rice OsNF-YB7, an ortholog of Arabidopsis LEC1, in chlorophyll biosynthesis, uncovering the genetic and molecular basis for negative regulation of chlorophyll production in the rice embryo. Mutational analysis, gene expression profiles and protein interaction combine for convincing evidence that OsNF-YB7 represses chlorophyll biosynthesis.
https://doi.org/10.7554/eLife.96553.3.sa0Important: Findings that have theoretical or practical implications beyond a single subfield
- Landmark
- Fundamental
- Important
- Valuable
- Useful
Convincing: Appropriate and validated methodology in line with current state-of-the-art
- Exceptional
- Compelling
- Convincing
- Solid
- Incomplete
- Inadequate
During the peer-review process the editor and reviewers write an eLife Assessment that summarises the significance of the findings reported in the article (on a scale ranging from landmark to useful) and the strength of the evidence (on a scale ranging from exceptional to inadequate). Learn more about eLife Assessments
Abstract
As a master regulator of seed development, Leafy Cotyledon 1 (LEC1) promotes chlorophyll (Chl) biosynthesis in Arabidopsis, but the mechanism underlying this remains poorly understood. Here, we found that loss of function of OsNF-YB7, a LEC1 homolog of rice, leads to chlorophyllous embryo, indicating that OsNF-YB7 plays an opposite role in Chl biosynthesis in rice compared with that in Arabidopsis. OsNF-YB7 regulates the expression of a group of genes responsible for Chl biosynthesis and photosynthesis by directly binding to their promoters. In addition, OsNF-YB7 interacts with Golden 2-Like 1 (OsGLK1) to inhibit the transactivation activity of OsGLK1, a key regulator of Chl biosynthesis. Moreover, OsNF-YB7 can directly repress OsGLK1 expression by recognizing its promoter in vivo, indicating the involvement of OsNF-YB7 in multiple regulatory layers of Chl biosynthesis in rice embryo. We propose that OsNF-YB7 functions as a transcriptional repressor to regulate Chl biosynthesis in rice embryo.
Introduction
Angiosperms can be divided into chloroembryophytes and leucoembryophytes, depending on the presence or absence of chlorophyll (Chl) in the embryo, respectively (Puthur et al., 2013; Smolikova and Medvedev, 2016). Some plant species such as Arabidopsis (A. thaliana) produce chloroembryos, which have photochemically active chloroplasts capable of producing assimilates that are further converted into reserve biopolymers (Simkin et al., 2020). Grass species, such as rice (Oryza sativa), are unable to produce Chl during embryo development. To the best of our knowledge, it remains completely unclear what determines the ability to biosynthesize Chl in plant embryo. However, studies have identified several genes that contribute to Chl degradation for chloroembryos, the mutation of which can lead to a stay-green phenotype in mature seeds such as Mendel’s green pea (Sato et al., 2007; Delmas et al., 2013; Wang et al., 2018; Li et al., 2017; Thomas and Ougham, 2014).
Golden 2-Like (GLK) genes encode GARP-type transcription factors (TFs), which are key components regulating chloroplast development and Chl biosynthesis in plants (Rossini et al., 2001; Fitter et al., 2002; Nakamura et al., 2009). GLK can recognize the CCAATC cis-element of the Chl biosynthesis- and photosynthesis-associated nuclear genes to trigger their expression (Waters et al., 2009). The G-box (CACGTG) is also enriched in the GLK-targeted genes in Arabidopsis, possibly due to GLK being able to interact with certain G-box binding factors (Tamai et al., 2002). A genetic study showed that GLK activates Chl biosynthesis in roots, in a manner dependent on the G-box binding TF Elongated Hypocotyl 5 (HY5) (Kobayashi et al., 2012).
There are two copies of GLK in the rice genome, designated as OsGLK1 and OsGLK2 (Fitter et al., 2002). They redundantly regulate a set of genes, such as rice Chlorophyllide A Oxygenase (OsCAO) and Protochlorophyllide Oxidoreductase A (OsPORA) responsible for Chl biosynthesis, and Light Harvesting Complex B1 (OsLHCB1) and OsLHCB4 responsible for photosynthesis (Nakamura et al., 2009; Wang et al., 2013; Sakuraba et al., 2017). OsGLK1 overexpression in rice leads to green calli and chloroplast development in the vascular bundles (Nakamura et al., 2009; Wang et al., 2017). Organelle development in rice vascular sheath cells is induced by ectopically expressed maize (Zea mays) GLK genes, mimicking a key step in the evolutionary transition from C3 to C4 plants (Wang et al., 2017). In accordance with this, the ectopic expression of maize GLKs in rice can boost biomass and grain yield by facilitating chloroplast development and photosynthesis (Li et al., 2020; Yeh et al., 2022). Moreover, the overexpression of maize GLKs in calli was shown to improve the ability of rice and maize to regenerate (Luo et al., 2023). A recent study found that OsGLK1 also participates in tapetum plastid development and programmed cell death, consequently affecting pollen fertility in rice (Zheng et al., 2022). The findings indicate that GLK plays multiple roles in relation of plant development.
Leafy Cotyledon 1 (LEC1), a member of the nuclear factor Y (NF-Y) TF family, is a central regulator controlling many aspects of seed development, such as Chl accumulation in the embryo (Meinke et al., 1994; Pelletier et al., 2017). LEC1 can also act as a pioneer TF to regulate flowering by reprogramming the embryonic chromatin state (Tao et al., 2017). Previous studies have reported that lec1 mutants have paler green embryos than wild-type (WT) at maturation in Arabidopsis (Meinke et al., 1994; West et al., 1994). LEC1 transcriptionally regulates the expression of genes that encode light-reaction components of photosystems I and II, as well as the expression of genes involved in chloroplast biogenesis in Arabidopsis and soybean embryos (Pelletier et al., 2017; Jo et al., 2020). These findings suggest that LEC1 is important for photosynthesis and chloroplast development during seed development. However, the underlying molecular mechanisms remain largely unclear.
It is still a mystery why plant species such as rice cannot synthesize chlorophyll in embryos, while species such as Arabidopsis can. There are two LEC1 homologs, OsNF-YB7 and OsNF-YB9, encoded by the rice genome (Zhiguo et al., 2018). OsNF-YB7 is restricted to the embryo, and defective OsNF-YB7 may result in seed lethality (Niu et al., 2021b; Zhang and Xue, 2013). Here, we found that OsNF-YB7 acts as a key inhibitor of Chl biosynthesis in rice embryo. It inactivates the transactivation activity of OsGLK1, at multiple regulatory layers, to inhibit Chl accumulation in the embryo of rice, explaining the achlorophyllous embryo produced in rice.
Results
Loss of function of OsNF-YB7 leads to chloroembryos
By observing seeds produced by the loss-of-function mutant of OsNF-YB7, we surprisingly found that the osnf-yb7 embryo was greenish (Figure 1A and B), suggesting that OsNF-YB7 plays a negative role in chloroplast biogenesis or Chl biosynthesis, or both, during embryogenesis. We therefore examined the phenotype of green embryos at various seed development stages. The results showed that WT had an achlorophyllous embryo throughout embryonic development, whereas osnf-yb7 embryo turned green at 5 days after fertilization (DAF) and the chloroembryo remained green until maturity (Figure 1—figure supplement 1A–C). In support of this, transmission electron microscopy (TEM) showed well-developed chloroplast in the scutellum tip of the mutant, but this was not seen in the WT sections (Figure 1C). By measuring Chl contents in the developing and mature embryos, we found that total Chl content in osnf-yb7 was consistently higher than that in the WT, both at 10 DAF and at maturation (30 DAF) (Figure 1D). As revealed by confocal laser scanning microscopy (CLSM), Chl autofluorescence was detectable as early as 5 DAF in osnf-yb7, indicating the initiation of Chl accumulation at this stage (Figure 1E). This is consistent with our previous finding that OsNF-YB7 was highly activated at 5 DAF in rice embryo (Niu et al., 2021b).
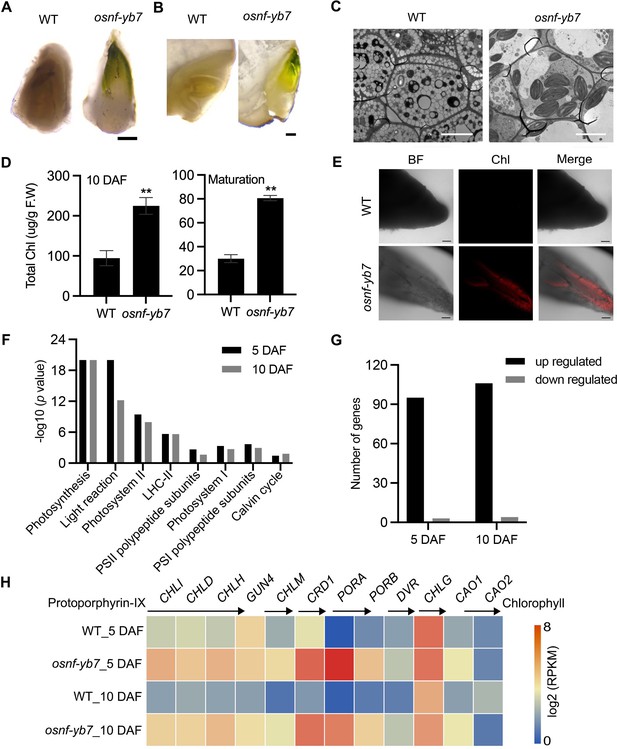
OsNF-YB7 negatively regulates Chlorophyll (Chl) biosynthesis in embryo.
(A, B) Morphologies of wild-type (WT) and osnf-yb7 detached embryos at 10 days after fertilization (DAF) (A) and longitudinally dissected embryos at maturation (B). Scale bars = 200 μm. (C) Transmission electron microscopy images of embryos from WT and osnf-yb7 at 10 DAF. Scale bars = 5 μm. (D) Chl levels in WT and osnf-yb7 embryos at 10 DAF and maturation. Data are means ± SD (n=3). **, p<0.01; Student’s t-test was used for statistical analysis. (E) Chl autofluorescence of WT and mutant embryos at 5 DAF. BF, bright field; Chl, Chl autofluorescence. Scale bars = 100 μm. (F) Photosynthesis-related pathways enriched among the differentially expressed genes (DEGs) identified from the 5- and 10-DAF-old osnf-yb7 embryos compared to WT, respectively. (G) Most of the photosynthesis-related genes were upregulated in the mutant embryos. (H) A heat map shows the expression of the Chl biosynthesis-related genes in the WT and osnf-yb7 embryos at 5 and 10 DAF. Reads per kilobase per million mapped reads (RPKM) is used to indicate the expression level.
Light is a critical signal triggering Chl biosynthesis (Wietrzynski and Engel, 2021). To determine whether the Chl biosynthesis in osnf-yb7 is induced by light, we investigated the embryo phenotype of WT and osnf-yb7 in the dark, using aluminum foil to cover rice panicles prior to flowering. The result showed that, similar to WT, osnf-yb7 embryo was achlorophyllous in the dark, although the embryogenesis defects, such as degenerated epiblast and coleorhiza, and maldeveloped coleoptile, were still observable (Figure 1—figure supplement 2A–H). This indicated that Chl biosynthesis in the mutant is light dependent. Only a small amount of light can be perceived by rice embryos because the external hulls block light penetration (Simkin et al., 2020). We removed the hulls to directly expose the embryo to light; however, Chl accumulation still failed to occur in the WT embryo (Figure 1—figure supplement 2I–L), suggesting that there are internal signals that repress Chl biosynthesis in rice embryo. We suspected that the OsNF-YB7 mutation may attenuate the activity of such inhibitors; alternatively, OsNF-YB7 itself could be an inhibitor.
OsNF-YB7 negatively regulates photosynthesis- and Chl biosynthesis-related genes
Using 5- and 10-DAF-old WT and osnf-yb7 embryos, we previously performed deep sequencing of the transcriptome (RNA-seq) to identify possible downstream genes of OsNF-YB7 (Niu et al., 2021a). As revealed by Mapman analysis, photosynthesis-related pathways, such as photosystem I, photosystem II, and the light reaction, were significantly enriched for the differentially expressed genes (DEGs) in the mutant embryos (Figure 1F, Figure 1—figure supplement 3A and B). Moreover, 96.9% (95/98) and 96.4% (106/110) of the photosynthesis-related DEGs that identified from 5- and 10–DAF-old embryos, respectively, were upregulated in the mutant (Figure 1G). To confirm this finding, we next examined the expression of OsLHCAs and OsLHCBs, which are primarily associated with photosystems I and II, respectively, via quantitative real-time PCR (RT-qPCR). It showed higher expression of all of the studied OsLHCAs and OsLHCBs in the osnf-yb7 embryo at 10 DAF (Figure 1—figure supplement 4A). Likewise, many of the genes participating in Chl biosynthesis, including rice Genomes Uncoupled 4 (OsGUN4), Mg-Chelatase H Subunit (OsCHLH), OsCHLI, OsCHLD, Copper Response Defect 1 (OsCRD1), OsPORA, OsPORB, and Divinyl Reductase (OsDVR), were significantly activated in the mutant (Figure 1H and Figure 1—figure supplement 4B). The findings indicated that OsNF-YB7 might act as a repressor of Chl biosynthesis and photosynthesis, which is opposite to the role of its homologue LEC1 in Arabidopsis.
Since OsNF-YB7 is a TF, we assumed that it may directly regulate the expression of genes related to Chl biosynthesis and photosynthesis, such as OsPORA, and OsLHCB4, which were significantly activated in the osnf-yb7 embryo (Figure 2A and B). We first generated transgenic lines that overexpressed OsNF-YB7, tagged with either green fluorescent protein (NF-YB7-GFP) or 3×Flag (NF-YB7-Flag) in the Zhonghua11 (ZH11, O. sativa ssp. geng/japonica) or Kitaake (O. sativa ssp. geng/japonica) background, respectively. As expected, OsPORA and OsLHCB4 were significantly downregulated in leaves of the OsNF-YB7 overexpressors (Figure 2C and D, and Figure 1—figure supplement 4C). Similar to previously reported (Zhang and Xue, 2013; Ito et al., 2011), the OsNF-YB7 overexpression lines displayed severe reproductive development defects, which prevented us from obtaining sufficient embryo tissues for subsequent experiments. Instead, using the NF-YB7-Flag seedling, we conducted a chromatin immunoprecipitation assay coupled with quantitative PCR (ChIP-qPCR). The results showed that OsNF-YB7 was highly enriched in the promoter regions of OsPORA and OsLHCB4 harboring the G-box motif (Figure 2E and F), a putative binding site of OsNF-YB7 (Guo et al., 2022). To confirm the ability of OsNF-YB7 to bind to the OsPORA and OsLHCB4 promoters, we next performed electrophoretic mobility-shift assays (EMSAs), using recombinant OsNF-YB7-His protein, and biotin-labeled subfragments of the OsPORA or OsLHCB4 promoters containing G-boxes. The results showed that OsNF-YB7-His was able to bind to the labeled probes, and the shifted band signals were substantially weakened upon application of the unlabeled cold probes or hot probes with a mutated G-box (Figure 2G and H).
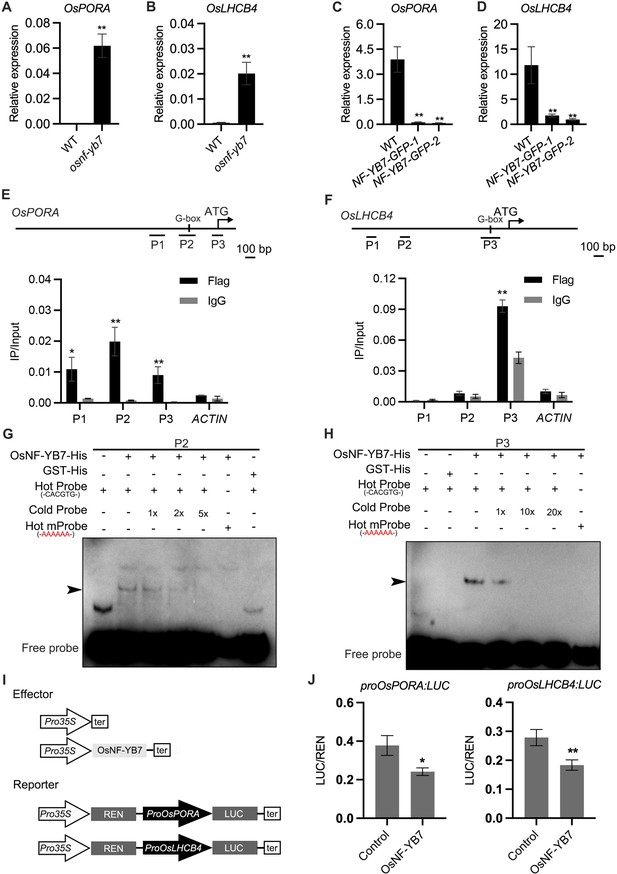
OsNF-YB7 binds to the promoters of OsPORA and OsLHCB4 to regulate their expression.
(A, B) Quantitative real-time PCR (RT-qPCR) analysis of the transcription levels of OsPORA (A) and OsLHCB4 (B) in the embryos of WT and osnf-yb7 at 10 DAF. Data are means ± SD (n=3). **, p<0.01; Student’s t-test was used for statistical analysis. (C, D) Expression of OsPORA (C) and OsLHCB4 (D) in the leaves of WT and OsNF-YB7-overexpressing transgenic plants (NF-YB7-GFP). Data are means ± SD (n=3). **, p<0.01; Student’s t-test was used for statistical analysis. (E, F) Chromatin immunoprecipitation assay coupled with quantitative PCR (ChIP-qPCR) analyses showing the enrichment of OsNF-YB7 at the OsPORA (E) and OsLHCB4 (F) promoters in 14-day-old OsNF-YB7-Flag seedlings. Precipitated DNA was quantified by qPCR and DNA enrichment is displayed as a percentage of input DNA. Data are means ± SD (n=3). *, p<0.05; **, p<0.01; Student’s t-test was used for statistical analysis. ACTIN was used as a nonspecific target gene. Diagrams in the upper panel showing the promoter structures of OsPORA and OsLHCB4, and the PCR amplicons used for ChIP-qPCR. (G, H) Electrophoretic mobility-shift assays (EMSAs) showing that OsNF-YB7 directly binds to the promoters of OsPORA (G) and OsLHCB4 (H). Hot probes were biotin-labeled. The hot mProbes contain mutant nucleic acid from CACATG to AAAAAA. The arrow heads indicate the shift bands. (I) Schematic diagram displaying the constructs used in the dual luciferase reporter (DLR) assays of J. LUC, firefly luciferase; REN, Renilla luciferase. (J) DLR assays showing that OsNF-YB7 directly represses the promoter activities of OsPORA and OsLHCB4. Data are means ± SD (n=3). *, p<0.05; **, p<0.01; Student’s t-test was used for statistical analysis.
-
Figure 2—source data 1
Uncropped and labelled gels.
- https://cdn.elifesciences.org/articles/96553/elife-96553-fig2-data1-v1.zip
-
Figure 2—source data 2
Raw unedited gels.
- https://cdn.elifesciences.org/articles/96553/elife-96553-fig2-data2-v1.zip
To confirm that the binding of OsNF-YB7 represses target gene expression, dual luciferase (LUC) reporter (DLR) assays were performed. We first generated LUC reporters driven by the OsPORA or OsLHCB4 promoters (designated as proOsPORA:LUC and proOsLHCB4:LUC hereafter) (Figure 2I). When the reporters were coexpressed with OsNF-YB7, which acted as an effector, in rice protoplasts, we found that OsNF-YB7 significantly repressed the activity of both proOsPORA:LUC and proOsLHCB4:LUC (Figure 2J). Taken together, these results suggested that OsNF-YB7 directly binds to the promoters of photosynthesis- and Chl-biosynthesis-related genes and represses their transcription.
OsNF-YB7 represses OsGLK1 in the embryo
Several TFs that regulate Chl biosynthesis or chloroplast development have been identified in plants (Jarvis and López-Juez, 2013). Some of these, such as rice OsGLK1, HY5-like 1 (OsHY5L1), PIF-like 14 (OsPIL14), and GATA Nitrate-inducible Carbon-metabolism-involved (OsGNC), were found to be upregulated in the embryo of osnf-yb7 (Figure 3A).
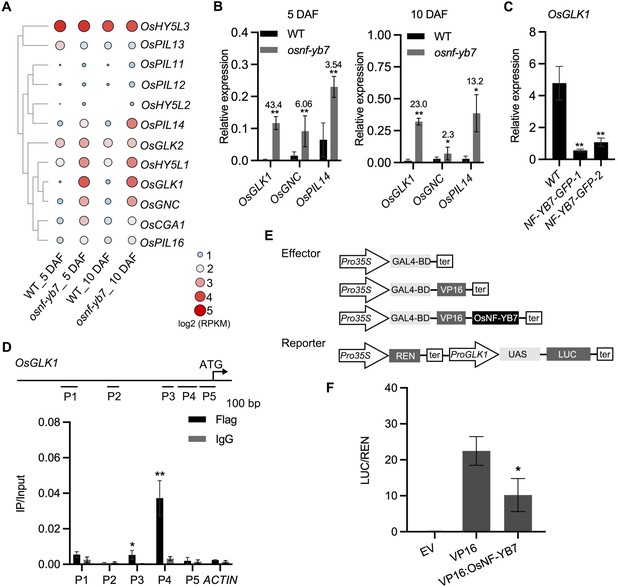
OsNF-YB7 associates with the promoter of OsGLK1 and represses its expression.
(A) A heat map showing the expression of transcription factors associated with Chl biosynthesis and chloroplast development in the 5- and 10-DAF-old embryos of WT and osnf-yb7. The colored dots indicate log2(RPKM mean) of the genes in three biological replicates. (B) RT-qPCR analysis of OsGLK1, OsGNC, and OsPIL14 expression levels in the embryos from WT and osnf-yb7 at 5- and 10 DAF. Numbers represent fold changes of expression. Data are means ± SD (n=3). *, p<0.05; **, p<0.01; Student’s t-test was used for statistical analysis. (C) RT-qPCR analysis of OsGLK1 expression levels in leaves from WT and NF-YB7-GFP. Data are means ± SD (n=3). **, p<0.01; Student’s t-test was used for statistical analysis. (D) ChIP-qPCR analysis showing the enrichment of OsNF-YB7 at the OsGLK1 promoter in 14-day-old OsNF-YB7-Flag seedling. Chromatin of each sample was immunoprecipitated using anti-Flag or IgG antibodies. Precipitated DNA was quantified by qPCR and DNA enrichment is displayed as a percentage of input DNA. Data are means ± SD (n=3). *, p<0.05; **, p<0.01; Student’s t-test was used for statistical analysis. ACTIN was used as a nonspecific target gene. The experiment was performed three times with similar results. The diagram in the upper panel showing the promoter structure of OsGLK1 and PCR amplicons (P1, P2, P3, P4, and P5) used for ChIP-qPCR. (E) Schematic diagram displaying the constructs used in the DLR assays of F. LUC, firefly luciferase; REN, Renilla luciferase; UAS, upstream activating sequence. (F) DLR assays showing that OsNF-YB7 represses the promoter activity of OsGLK1. Data are means ± SD (n=3). *, p<0.05; Student’s t-test was used for statistical analysis.
OsGLK1 was the most strikingly activated TF (Figure 3A and B), while it was significantly repressed in NF-YB7-GFP (Figure 3C). To test the idea that OsGLK1 is a direct downstream target of OsNF-YB7, a ChIP-qPCR assay was first carried out using NF-YB7-Flag transgenic seedlings. The results showed that the promoter segments P3 and P4 were significantly enriched in the immunoprecipitated chromatin (Figure 3D), suggesting that OsNF-YB7 was able to bind to the OsGLK1 promoter in vivo. However, we failed to validate the binding ability in vitro using the EMSA assay (Figure 3—figure supplement 1), suggesting that OsNF-YB7 requires other NF-Ys or TFs to form a TF complex to recognize the promoter, as many NF-Y members do for function (Laloum et al., 2013). A DLR assay was next performed to investigate the negative regulation of OsNF-YB7 on OsGLK1 transcription using rice protoplasts. The OsGLK1 promoter was inserted upstream of the 5×upstream activating sequence (UAS) as a reporter; OsNF-YB7 was fused C-terminally to the DNA binding domain (BD) of the yeast GAL4 and the herpes virus VP16 transactivation domain (VP16:OsNF-YB7), as an effector (Figure 3E). Coexpression of VP16:OsNF-YB7 with the reporter in rice protoplasts significantly decreased the transcriptional activity of VP16 (Figure 3F), indicating that OsNF-YB7 represses OsGLK1 promoter activity.
OsGLK1 is involved in OsNF-YB7-regulated Chl biosynthesis in embryo
To confirm the contribution of OsGLK1 for the production of chloroembryo in osnf-yb7, we first generated OsGLK1-overexpressing lines (OsGLK1-OX) driven by the rice ubiquitin promoter. In association with over-accumulated Chl in the glume and seed coat of the transformant, RT-qPCR and Western blot assays confirmed OsGLK1 activation in OsGLK1-OX (Figure 4—figure supplement 1A-C). As observed in osnf-yb7, green embryos were produced (Figure 4A). The Chl content in OsGLK-OX were higher than that in WT (Figure 4B and C), suggesting that OsGLK1 overexpression in rice embryo induces Chl biosynthesis.
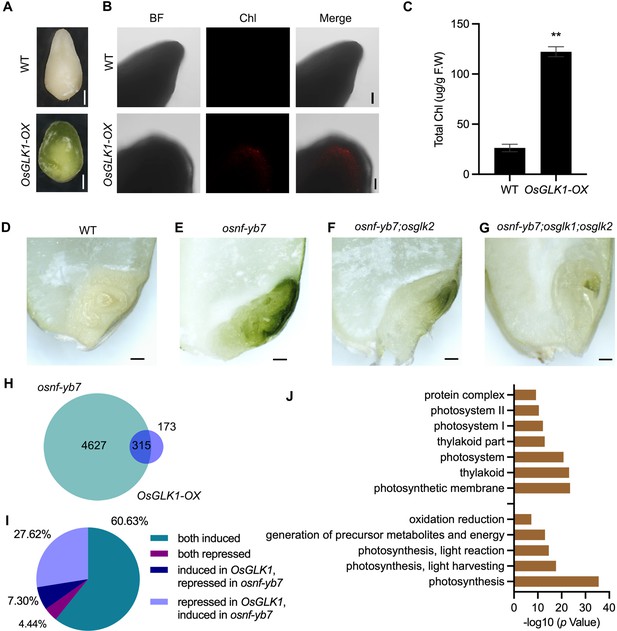
Chl biosynthesis in osnf-yb7 embryo requires active OsGLKs.
(A) Embryo morphologies of WT and OsGLK1-OX detached embryos at 10 DAF. Scale bars = 2 mm. (B) Chl autofluorescence of the WT and GLK-OX embryos at 10 DAF. BF, bright field; Chl, Chl autofluorescence. Scale bars = 100 μm. (C) Chl levels in WT and OsGLK1-OX embryos at maturation. Data are means ± SD (n=3). **, p<0.01; Student’s t-test was used for statistical analysis. (D–G) Morphologies of the embryos produced by WT (D), osnf-yb7 (E), osnf-yb7;osglk2 double mutant (F) and osnf-yb7;osglk1;osglk2 triple mutant (G). Scale bars = 1 mm. (H) A Venn diagram showing overlaps of the DEGs identified from the embryos of osnf-yb7 and OsGLK1-OX at 10 DAF. (I) A pie chart showing similar transcriptional changes of the common DEGs identified from osnf-yb7 and OsGLK1-OX. (J) Gene Ontology (GO) analysis of the common DEGs identified from osnf-yb7 and OsGLK1-OX.
-
Figure 4—source data 1
Differentially expressed genes (DEGs) in the 10-DAF-old embryos of OsGLK1-OX.
- https://cdn.elifesciences.org/articles/96553/elife-96553-fig4-data1-v1.xlsx
-
Figure 4—source data 2
Common DEGs identified from the OsGLK1-OX and osnf-yb7 embryos at 10 DAF.
- https://cdn.elifesciences.org/articles/96553/elife-96553-fig4-data2-v1.xlsx
Using a clustered regularly interspaced short palindromic repeats (CRISPR)/CRISPR-associated 9 (Cas9) cassette including three tandemly arrayed guide RNAs targeting OsNF-YB7, OsGLK1, and OsGLK2, respectively, we successfully obtained the osnf-yb7;osglk2 double mutant and the osnf-yb7;osglk1;osglk2 triple mutant (Figure 4—figure supplement 2A and B). By analyzing the embryos that the mutants produced, we found that significantly less Chl accumulated in osnf-yb7;osglk1;osglk2 than in osnf-yb7 and osnf-yb7;osglk2 (Figure 4D–G and Figure 4—figure supplement 3). In comparison to the achlorophyllous embryo of WT, the osnf-yb7;osglk1;osglk2 triple mutant still showed somewhat green coloration in the apical part of the embryos (Figure 4G). We hypothesized that this was at least partially due to that genes like OsLHCB4 and OsPORA can be induced by the mutation of OsNF-YB7, given the fact that OsNF-YB7 represses the genes’ expression independent of OsGLK1 (Figure 2J).
OsNF-YB7 and OsGLK1 regulate a common set of genes in the embryo
We next performed RNA-seq analysis to explore the transcriptomic changes in the chloroembryo of OsGLK1-OX at 10 DAF. More than 64.4% of the DEGs identified in OsGLK1-OX were overlapped with the ones identified in osnf-yb7, the vast majority of which were either activated (60.6%) or repressed (27.6%) in both OsGLK1-OX and osnf-yb7 (Figure 4H and I, and Figure 4—source data 1 and 2). As revealed by the Gene Ontology (GO) analysis, genes involved in Chl biosynthesis and photosynthesis, such as OsPORA and OsLHCB4, were markedly enriched among the common DEGs (Figure 4J and Figure 4—figure supplement 4), implying that OsNF-YB7 and OsGLK1 antagonistically regulate a common set of genes for Chl biosynthesis and photosynthesis.
The ChIP-qPCR results showed that OsGLK1 associated with the regions of the OsPORA and OsLHCB4 promoters to which OsNF-YB7 binds (Figure 5A and B). In agreement with this, the EMSA results suggested that OsGLK1 directly binds to the same DNA probes of OsPORA and OsLHCB4 in vitro (Figure 5C and D). In opposite to OsNF-YB7, when we coexpressed the OsGLK1 effector vector with the reporter vector proOsLHCB4:LUC or proOsPORA:LUC in rice protoplasts, OsGLK1 showed significant transactivation activity on OsPORA and OsLHCB4 (Figure 5—figure supplement 1A and B).
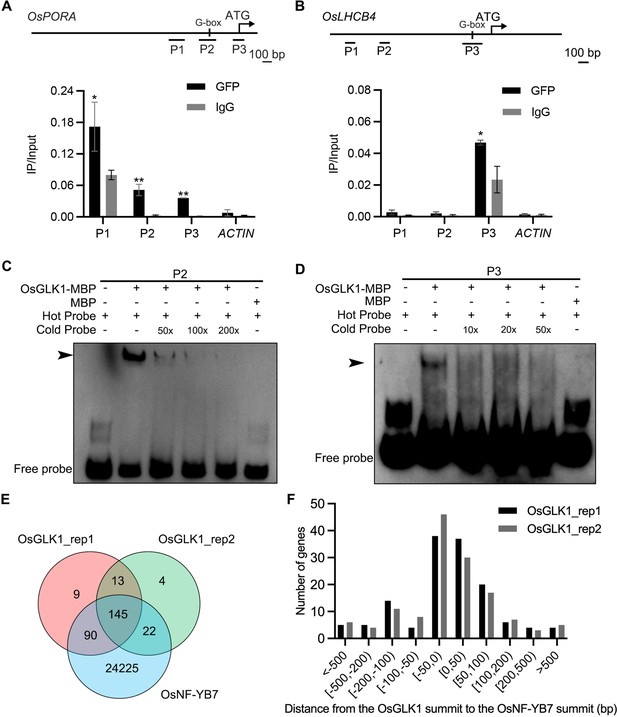
OsNF-YB7 and OsGLK1 regulate a common set of genes in the embryo.
(A, B) ChIP-qPCR analysis showing the enrichment of OsGLK1 on the OsPORA (A) and OsLHCB4 (B) promoters. PCR amplicons used for ChIP-qPCR are indicated in the schematic diagrams. OsGLK1-GFP was transiently expressed in protoplasts isolated from green tissues of the 14-day-old WT seedling. Chromatin of each sample was immunoprecipitated using anti-GFP or igG antibodies. Precipitated DNA was quantified by qPCR and DNA enrichment is displayed as a percentage of input DNA. Data are means ± SD (n=3). *, p<0.05; **, p<0.01; Student’s t-test was used for statistical analysis. ACTIN was used as a nonspecific target gene. The experiment was performed three times with similar results. (C, D) EMSA assays showing that OsGLK1 directly binds to the promoters of OsPORA (C) and OsLHCB4 (D). The arrow heads indicate the shift bands. (E) A Venn diagram showing overlaps of the target genes between OsGLK1 and OsNF-YB7. (F) Distribution of the distance between the OsNF-YB7 and OsGLK1 summits showing that OsNF-YB7 and OsGLK1 bind to proximal regions of their common targets.
-
Figure 5—source data 1
Common targets of OsGLK1 and OsNF-YB7.
- https://cdn.elifesciences.org/articles/96553/elife-96553-fig5-data1-v1.xlsx
-
Figure 5—source data 2
Uncropped and labelled gels.
- https://cdn.elifesciences.org/articles/96553/elife-96553-fig5-data2-v1.zip
-
Figure 5—source data 3
Raw unedited gels.
- https://cdn.elifesciences.org/articles/96553/elife-96553-fig5-data3-v1.zip
Recently, the putative binding sites of OsNF-YB7 and OsGLK1 were investigated at the whole genome scale (Guo et al., 2022; Tu et al., 2022). This allows us to test our hypothesis that OsNF-YB7 and OsGLK1 can target a common set of genes involved in Chl biosynthesis and photosynthesis. By reanalyzing the ChIP-seq data, we found that 91.4% (235/257) and 90.7% (167/184) of the OsGLK1-binding genes overlapped with the OsNF-YB7-binding genes in two replicates (Figure 5E and Figure 5—source data 1), although the peak number of OsNF-YB7 was much greater than that of OsGLK1 (Guo et al., 2022; Tu et al., 2022). A large number of the common targets were activated in the embryos of osnf-yb7 and OsGLK1-OX at 10 DAF (Figure 5—figure supplement 2A). GO analysis suggested that most of the common targets were genes involved in Chl biosynthesis or photosynthesis (Figure 5—figure supplement 2B). By retrieving the sequences of the OsNF-YB7 and OsGLK1 binding peaks in the common targets for MEME analysis, we found that the TFs probably recognize similar DNA motifs. A short sequence containing the G-box motif was the most significantly enriched (Figure 5—figure supplement 3A and B). There was also enrichment of another sequence containing a CCAAT motif recognized by the NF-Y TF complexes and a CCAATC motif recognized by GLKs, as previously reported (Waters et al., 2009; Pelletier et al., 2017; Figure 5—figure supplement 3A and B). The results suggested that OsNF-YB7 and OsGLK1 can bind to the same region of their common target. In agreement with this, approximately 75.0% and 76.5% of the peak summits of OsGLK1 were located proximally to the summit of OsNF-YB7 in two replicates, within an adjacent region no more than 100 bp away (Figure 5F and Figure 5—source data 1). Consistent with our biochemical evidence, the ChIP-seq results showed that OsNF-YB7 does bind to the same regions of the OsPORA and OsLHCB4 promoters as OsGLK1 binds to (Figure 5—figure supplement 3C).
OsNF-YB7 physically interacts with OsGLK1 to inhibit its transcriptional activity
To resolve how OsNF-YB7 and OsGLK1 bind to the same regions to regulate their common targets, we speculated that the TFs probably form a dimer in rice in order to exert their functions. We therefore carried out a yeast two-hybrid (Y2H) assay by transforming yeast cells with a bait construct expressing OsNF-YB7 fused with the GAL4 DNA-binding domain (BD), together with a prey construct expressing OsGLK1 fused with the yeast GAL4 activation domain (AD). The results showed that OsNF-YB7 interacts with OsGLK1 in yeast (Figure 6A and B). Furthermore, to determine the functional domains required for the interaction, we generated two truncated versions of OsNF-YB7, which contained the N- or C-terminal, and three truncated versions of OsGLK1, which contained the N-terminal, DNA-BD, or GCT box domain, as previously reported (Fitter et al., 2002; Zhang et al., 2021a; Figure 6A). We found that the C-terminus of OsNF-YB7 was sufficient for the interaction (Figure 6B). In addition, the GCT box domain of OsGLK1 strongly interacted with the full length or C-terminus of OsNF-YB7, while the DNA-BD of OsGLK1 showed a weak interaction (Figure 6B).
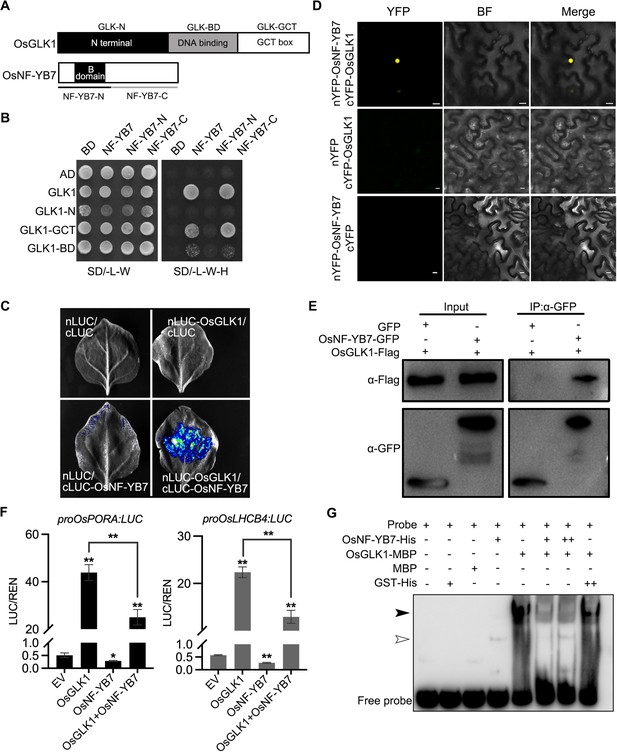
OsNF-YB7 interacts with OsGLK1 to regulate the expression of OsPORA and OsLHCB4.
(A) Schematic diagrams showing the protein structures of OsGLK1 and OsNF-YB7. (B) Yeast-two-hybrid (Y2H) assays showing the interaction between OsNF-YB7 and OsGLK1. AD and BD indicate the activation domain and binding domain of GAL4, respectively. The full length or truncated OsNF-YB7 and OsGLK1 were fused with BD and AD, respectively. The indicated combinations of constructs were cotransformed into yeast cells and grown on the nonselective medium SD/-L-W and selective medium SD/-L-W-H. (C) A split complementary luciferase (LUC) assay confirmed the interaction between OsNF-YB7 and OsGLK1. Coexpression of the fusion of OsGLK1 and the N-terminal half of LUC (nLUC-OsGLK1) and the fusion of the C-terminal half of LUC and OsNF-YB7 (cLUC-OsNF-YB7) in the epidermal cells of N. benthamiana leaves induced LUC activities, whereas the epidermal cells coexpressing nLUC-OsGLK1 and cLUC, nLUC and cLUC-OsNF-YB7, or nLUC and cLUC did not show LUC activities. (D) Bimolecular fluorescence complementation (BiFC) assays showed interactions between OsNF-YB7 and OsGLK1 in the nucleus. OsNF-YB7 was fused with the N-terminal of yellow fluorescent protein (nYFP-OsNF-YB7); OsGLK1 was fused with the C-terminal of yellow fluorescent protein (cYFP-OsGLK1). The indicated combinations of constructs were transiently coexpressed in the leaf epidermal cells of N. benthamiana. Scale bar = 10 μm. (E) Co-immunoprecipitation (Co-IP) assays showing that OsNF-YB7 interacts with OsGLK1 in vivo. 35 S::OsNF-YB7:GFP (OsNF-YB7-GFP) and 35 S::OsGLK1:3×Flag (OsGLK1-Flag) were coexpressed in rice protoplasts and were immunoprecipitated with an anti-GFP antibody, and the immunoblots were probed with anti-GFP and anti-Flag antibodies. 35 S::GFP (GFP) was used as a negative control. (F) DLR assays showing that OsNF-YB7 represses the transactivation activity of OsGLK1 on OsPORA and OsLHCB4. Protoplasts isolated from etiolated seedlings were used for the analyses. EV, empty vector. Data are means ± SD (n=3). *, p<0.05; **, p<0.01; Student’s t-test was used for statistical analysis. (G) EMSA assay indicated that OsNF-YB7 inhibits the DNA binding of OsGLK1 to the promoter of OsPORA. The black and white arrow heads indicate the OsGLK1- and OsNF-YB7-bound probes, respectively; “+” and “++” indicate that 2- and 4 µM recombinant proteins were used for the reactions. The GST-His was used as a negative control.
-
Figure 6—source data 1
Uncropped and labelled gels.
- https://cdn.elifesciences.org/articles/96553/elife-96553-fig6-data1-v1.zip
-
Figure 6—source data 2
Raw unedited gels.
- https://cdn.elifesciences.org/articles/96553/elife-96553-fig6-data2-v1.zip
A split complementary LUC assay further confirmed the interaction between OsNF-YB7 and OsGLK1 in the epidermal cells of Nicotiana benthamiana (Figure 6C). As suggested by the bimolecular fluorescence complementation (BiFC) analysis, the interaction occurred exclusively in the nuclei of N. benthamiana epidermal cells (Figure 6D). Moreover, we transiently coexpressed OsNF-YB7 tagged with GFP (OsNF-YB7-GFP) and OsGLK1 tagged with 3×Flag (OsGLK1-Flag) in rice protoplasts, and co-immunoprecipitation (Co-IP) analysis showed that OsGLK1-Flag could be immunoprecipitated by the anti-GFP antibody (Figure 6E), indicating that the interactions do occur in vivo. In addition, both Y2H and split complementary LUC assays showed that OsGLK2 could interact with OsNF-YB7 (Figure 6—figure supplement 1A and B). These findings indicated that OsNF-YB7 interacts with OsGLKs, explaining why OsNF-YB7 and OsGLKs share a common set of targets in rice.
To explore the biological meaning of the interaction, we next performed DLR assays using rice protoplasts. Transient expression of OsGLK1 in rice protoplasts substantially activated the reporters, driven by either the OsPORA or the OsLHCB4 promoter; however, when we coexpressed OsNF-YB7 with OsGLK1, the transactivation ability of OsGLK1 was significantly repressed in rice protoplasts (Figure 6F). These findings suggested that OsNF-YB7/OsGLK1 dimerization reduces the transactivation ability of OsGLK1 for fine-tuning the Chl biosynthetic and photosynthetic genes, such as OsPORA and OsLHCB4. The EMSA assay showed that OsGLK1-MBP recombinant proteins could bind to the promoter of OsPORA; however, when we incubated the probe with OsGLK1-MBP and OsNF-YB7-His together, the binding ability of OsGLK1 substantially decreased (Figure 6G). As a control, when the probe was incubated with OsGLK1-MBP and GST-His, the binding ability of OsGLK1 remained unchanged (Figure 6G). These findings implied that the reduced transactivity of OsGLK1 is likely due to the formation of OsNF-YB7/OsGLK1 heterodimers inhibiting the binding of OsGLK1 to its downstream genes.
Discussion
In crops such as soybean and canola, the presence of green embryos is considered as a valuable trait due to its association with increased photosynthetic capacity, which consequently promotes fatty acid biosynthesis (Ruuska et al., 2004). This highlights the potential value of the green embryo. Nevertheless, the degradation of chlorophyll in mature seeds is necessary to prevent adverse effects on seed viability and meal quality (Chung et al., 2006).
By surveying 1094 species from 666 genera and 182 families, Yakovlev and Zhukova, 1980 found that 428 angiosperms produce embryos with the presence of chlorophyll. Seeds with chlorophyllous embryo are scattered throughout the angiosperms and have evolved in many unrelated groups, but they are more prevalent in non-endospermous seeds (Dahlgren, 1980). Currently, the evolutionary force driving the divergence of chloroembryophytes and leucoembryophytes remains largely unknown. Embryo photosynthesis contributes a large amount of oxygen to fuel energy-generating pathways in seed (Simkin et al., 2020). The presence of chlorophyll in the embryo facilitates photosynthesis at early developmental stages, potentially leading to improved seedling growth and vigor (Smolikova and Medvedev, 2016). In many chloroembryophytes, such as Arabidopsis, the embryo occupies a large proportion of the seed. From an evolutionary perspective, the presence of chlorophyll in the embryo may promote adaptation in such chloroembryophytes, as more reserves can be accumulated in the seed through active photosynthesis, better supporting the embryo development and subsequent seedling growth (Sela et al., 2020). On the other hand, some leucoembryophytes, such as rice, have a persistent endosperm rich in storage reserves to nourish embryo development (Liu et al., 2022). The acquisition of the ability to accumulate chlorophyll in the embryo is not necessary in such species. Although chlorophyllous embryos are rare among the ‘primitive’ angiosperm superorders, they are observed in some (but not all) Nymphaeales species that are surrounded by some amount of endosperm (Yakovlev and Zhukova, 1980). As there is a paucity of knowledge regarding the evolution of chlorophyllous embryos, further comprehensive studies are necessary.
Some algae and gymnosperm species have evolved an ability to synthesize Chl in the dark (Myers, 1940; Ranade et al., 2019; Bogorad, 1950). However, it has remained largely unclear whether access to light accounts for the induction of chloroembryos (Dahlgren, 1980; Periasamy and Vivekanandan, 1981; Liu et al., 2017). Here, we found that removing the tissues covering an embryo failed to produce chlorophyllous embryos in the WT; however, light avoidance inhibited Chl accumulation in osnf-yb7 embryos (Figure 1—figure supplement 2A–H). These results suggest that light is necessary but insufficient to trigger chloroplast biogenesis in rice. Because light itself does not induce Chl accumulation in rice embryo (Figure 1—figure supplement 2I–L), we inferred that there are intrinsic cues to repress chloroplast development in rice embryo. However, to the best of our knowledge, the underlying mechanism that determines an embryo’s ability to synthesize Chl is completely unknown. Here, we showed that OsNF-YB7, a LEC1 homolog of rice, acts as an inhibitor to repress Chl accumulation in the embryo. In line with this, a recent independent study also showed that the OsNF-YB7 null mutant accumulates Chl in the embryo, although the cause of this remains unresolved (Guo et al., 2022).
Several TFs involved in chloroplast development and photomorphogenesis in rice have been identified (Nakamura et al., 2009; Li et al., 2019; Bai et al., 2019; Hudson et al., 2013). Interestingly, we found that many of them were upregulated in the osnf-yb7 embryos. For example, OsGLK1 was shown to be the most activated (Figure 3A and B). Our genetic and biochemical evidence suggests that OsGLK1 is involved in the OsNF-YB7-mediated repression of Chl biosynthesis in rice embryo. OsGLK1 overexpression mimicked the chloroembryo phenotype in WT, while knockout of OsGLK1 and OsGLK2, simultaneously, suppressed Chl accumulation in osnf-yb7 embryo (Figure 4A–G). The biochemical experiments suggested that OsNF-YB7 associates with the promoter of OsGLK1, in vivo, to transcriptionally inactivate OsGLK1 (Figure 3D–F), indicating that OsGLK1 is a downstream target of OsNF-YB7. However, OsNF-YB7 alone failed to bind to the promoter of OsGLK1 in vitro (Figure 3—figure supplement 1), presumably due to some as-yet-unidentified TFs being recruited by OsNF-YB7, assisting in recognizing OsGLK1 for transcriptional regulation. An NF-Y TF complex usually consists of three subunits (NF-YA/B/C) in order to exert its function (Laloum et al., 2013). NF-YA is responsible for DNA binding, while NF-YB and NF-YC are primarily responsible for transactivation (Chaves-Sanjuan et al., 2021; Gnesutta et al., 2017). OsNF-YB7 possibly forms a heterotrimer with NF-YA and NF-YC in the embryo to recognize OsGLK1. In addition, previous studies showed that Arabidopsis LEC1 can interact with different TFs for developmental regulation (Huang et al., 2015b; Yamamoto et al., 2009; Fatihi et al., 2016; Boulard et al., 2018; Huang et al., 2015a). Identifying such TFs may reveal how OsNF-YB7 recognizes OsGLK1 in order to exert its function.
OsNF-YB7 could recognize a common set of genes involved in Chl biosynthesis and photosynthesis that are recognized by OsGLK1 (Figure 2E–H and Figure 5—source data 1). The biochemical results suggested that OsNF-YB7 can directly repress OsPORA and OsLHCB4, which are activated by OsGLK1 (Figure 2J, and Figure 5—figure supplement 1). OsNF-YB7 probably hinders OsGLK1 to access the target genes by forming an OsGLK1/OsNF-YB7 heterodimer (Figure 6A–E), or by occupying the motif OsGLK1 recognized in the promoter, given the binding sites of OsGLK1 and OsNF-YB7 are likely overlapped (Figure 5F, Figure 5—figure supplement 3 and Figure 5—source data 1). The first of these hypotheses is more plausible because, upon co-incubation of OsGLK1 and OsNF-YB7 with the OsPORA promoter in vitro, the shifted band signal of OsGLK1 was substantially decreased (Figure 6G), indicating that the protein-protein interactions overwhelm the protein-DNA interactions. Thus, when OsNF-YB7 is expressed, it interacts with OsGLK1, then OsGLK1 is less available to activate downstream targets. The findings suggested that OsNF-YB7 plays a dual role in regulating Chl biosynthesis in rice embryo: first, it represses the downstream genes, achieved via its function as a transcriptional inactivator; second, OsNF-YB7 can interact with OsGLKs to disturb their abilities to transactivate genes related to Chl biosynthesis and photosynthesis (Figure 6—figure supplement 2). In addition to OsNF-YB7, a recent study showed that Deep Green Panicle 1, a plant-specific protein with a conserved TIGR01589 domain, can interact with OsGLKs to suppress OsGLK-mediated transcription (Zhang et al., 2021b). The findings suggested that, as the central regulator responsible for chloroplast development, GLK is tightly regulated at the post-translational level to fine-tune Chl biosynthesis in plants.
Previous studies suggested that Arabidopsis LEC1 is a positive regulator of Chl biosynthesis (Pelletier et al., 2017), given that the mature embryos of lec1 were paler than the WT (Meinke, 1992; West et al., 1994). However, the null mutation of OsNF-YB7 activated Chl biosynthesis, implying that the LEC1-type gene acts as a negative regulator in rice. By surveying the literature, we noted that Meinke reported that the cotyledons of the lec1 mutant remained green unusually late in development (Meinke, 1992). Although there was no significant difference in Chl content upon using whole seeds for quantification, Parcy et al. did observe that the tip of lec1 cotyledons accumulated more Chl (Parcy et al., 1997). Moreover, the lec1;abi3 double mutant embryos produced much more Chl than the abi3 single mutant (Parcy et al., 1997). Pelletier et al. recently reported that a cluster of LEC1 targets is enriched in genes related to photosynthesis and chloroplast development, and many of which are down-regulated in the embryo of lec1 (Pelletier et al., 2017); however, we noticed that in their dataset, the photosynthesis and Chl biosynthesis related genes were more strikingly enriched in the up-regulated genes of lec1, at either the mature green or postmature green stage. These observations challenge the concept that LEC1 positively regulates Chl biosynthesis and photosynthesis in Arabidopsis. Most studies in Arabidopsis have emphasized the importance of LEC1 in embryo development at the maturation stage. However, LEC1 is activated within 24 hr after fertilization (Lotan et al., 1998), but its role in the early embryo developmental stages for Chl biosynthesis is still unknown. Previously, we have expressed OsNF-YB7 in the lec1-1 background, driven by the native promoter of Arabidopsis LEC1 (Niu et al., 2021b). Given that OsNF-YB7 could rescue embryo morphogenesis defects in Arabidopsis (Niu et al., 2021b), we postulated that OsNF-YB7 in rice plays a similar role to that of LEC1 in Arabidopsis. In order to ascertain whether LEC1 can fully restore osnf-yb7, it is necessary to ectopically express LEC1 driven by the native OsNF-YB7 promoter in the osnf-yb7 background in the future, due to the possibility of functional divergence between the genes with regard to the regulation of chlorophyll biosynthesis in the embryo. It would also be worthwhile to carry out similar tests in other grass organisms to better understand the regulatory mechanism in rice.
In addition to producing chlorophyllous embryo, the osnf-yb7 mutants display an array of developmental defects, including abnormal embryogenesis, reduced dormancy, and desiccation intolerance, similar to those found in Arabidopsis lec1 mutants (Niu et al., 2021a). Mutation of osglk1 and osglk2 in osnf-yb7 could recover the chloroembyo phenotype, but did not alleviate the other embryo defects (Figure 4D–G). The findings suggest that OsGLKs specifically function in Chl biosynthesis, but that OsNF-YB7 is responsible for many aspects of embryo development. In agreement with this, the number of OsNF-YB7-targeted genes is far greater than that of OsGLK-targeted genes, and the DEGs in osnf-yb7 embryo is far greater than that in OsGLK1-OX embryo (Figure 4H and Figure 5E). The mechanisms underlying the OsNF-YB7-regulated multiple embryo developmental processes require further investigation.
Materials and methods
Plant materials and growth conditions
Request a detailed protocolThe osnf-yb7 mutant lines used in this study were previously generated in our laboratory (Niu et al., 2021b). Rice cultivars Zhonghua11 (ZH11) and Kitaake (Kit) were used for gene transformation. Rice plants were grown in a paddy field in Yangzhou, Jiangsu Province, China, or in a climate-controlled room under long-day conditions with a photocycle of 14 hr of light (32 °C) and 10 hr of darkness (28 °C), at 50% humidity. The N. benthamiana plants were grown in a growth chamber at 22 °C with long-day conditions (16 hr light/8 hr dark). To determine whether the chloroembryo of osnf-yb7 is light-dependent, the emerging panicles of the mutants were covered with aluminum foil until seed maturation. The lemmas of the WT were carefully removed with forceps at 1–2 DAF to expose the WT embryo to light in a climate-controlled room.
Vector construction and plant transformation
Request a detailed protocolTo generate the overexpression plants, full-length coding sequences (CDSs) of OsNF-YB7 or OsGLK1 (the stop codon removed) were cloned into pCAMBIA1300-Flag or pUN1301-GFP under the control of ubiquitin promoter, using the ClonExpress II One Step Cloning Kit (Vazyme). The constructs were transformed into rice calli through an Agrobacterium‐mediated strategy, as described previously (Chen et al., 2016). The higher order mutants of OsNF-YB7, OsGLK1 and OsGLK2 mutants, were generated using a previously described method for multiple gene editing in ZH11 (Cheng et al., 2021). The primers used for vector construction are listed in Supplementary file 1.
RNA extraction and RT-qPCR
Request a detailed protocolEmbryos or seedlings were collected by flash freezing in liquid nitrogen and stored at −80 °C until processing. Samples were finely ground using a mortar and pestle with liquid nitrogen. Total RNA was isolated using the RNA-easy Isolation Reagent (Vazyme, R701-01). The experiments were performed with at least three biological replicates. The relative expression levels of the tested genes were normalized using the rice Actin gene and calculated by the 2ΔCt method. The primers used for the RT-qPCR are listed in Supplementary file 1.
RNA-sequencing and data analysis
Request a detailed protocolTen-DAF-old embryos of the Wild-type (WT) and OsGLK1-OX were used for RNA-sequencing. Two biological replicates were set. RNA extraction, library preparation, and high-throughput sequencing of the collected samples were outsourced to BGI Genomics Co., Ltd., Shenzhen, China. CLC Genomics Workbench 12.0 software was used for RNA-seq data analysis, as previously reported (Xu et al., 2021). The thresholds of fold change >2 and Bonferroni-corrected FDR <0.05 were used for defining a DEG. The previously generated ChIP-seq data of OsNF-YB7 and OsGLK1 (Guo et al., 2022; Tu et al., 2022) were reanalyzed using CLC Genomics Workbench 12.0 software for peak calling. Enriched motifs were identified by the online tool MEME-ChIP (https://meme-suite.org/meme/tools/meme-chip) with default parameter set. The software Mapman was used for pathway analysis (Usadel et al., 2009). The online tool AgriGO 2.0 was used for GO analysis (Du et al., 2010). The Venn diagrams were drawn using an online tool (https://bioinfogp.cnb.csic.es/tools/venny/index.html). TBtools was used for heat map generation (Chen et al., 2020).
Dual luciferase reporter (DLR) assays
Request a detailed protocolThe OsGLK1, OsLHCB4 (2 kb upstream of translation start site), and OsPORA (1.5 kb upstream of translation start site) promoter sequences were amplified from ZH11 genomic DNA and cloned into the vector pGreenII 0800-LUC (Hellens et al., 2005), as reporters; the 35 S::OsGLK1 and 35 S::OsNF-YB7 constructs were used as effectors. The reporters and effectors were transfected into rice etiolated protoplasts in different combinations and incubated overnight. Firefly LUC and Renilla luciferase (REN) activities were measured using the DLR Assay Kit (Vazyme), following the manufacturer’s instructions, and the LUC:REN ratios were calculated for analysis. The primers used for generating these constructs are listed in Supplementary file 1.
Chromatin immunoprecipitation (ChIP) assays
Request a detailed protocolThe CDS (stop codons removed) of OsGLK1 was cloned into the pJIT163-GFP vector driven by a 35 S promoter to generate the OsGLK1-GFP construct. Protoplasts transformed with OsGLK1-GFP and OsNF-YB7-Flag transgenic lines were used for the ChIP assays, in accordance with a previously described method (Zhao et al., 2020). Briefly, protoplasts or 0.2 g of 14-day-old seedlings were harvested and crosslinked with 1% formaldehyde for 15 min, followed by neutralization using 0.125 M glycine for an additional 5 min. The seedlings were then ground into powder in liquid nitrogen. The nuclei were isolated and lysed using Buffer S (50 mM HEPES-KOH [pH 7.5], 150 mM NaCl, 1 mM ethylenediaminetetraacetic acid [EDTA], 1% Triton X-100, 0.1% sodium deoxycholate, 1% SDS) and Buffer F (50 mM HEPES-KOH [pH 7.5], 150 mM NaCl, 1 mM EDTA, 1% Triton X-100, 0.1% sodium deoxycholate). The chromatin was then sonicated with the segment size ranging from 200 to 600 bp. The lysates were then immunoprecipitated by anti-GFP (abcam no. ab290) and anti-Flag (Sigma no. F3165) antibodies, respectively. Immunocomplexes were washed with low-salt ChIP buffer (50 mM HEPES-KOH, 150 mM NaCl, 1 mM EDTA, 1% Triton X-100, 0.1% sodium deoxycholate, 0.1% SDS), high-salt ChIP buffer (low-salt ChIP buffer but replacing 150 mM NaCl with 350 mM NaCl), ChIP wash buffer (10 mM Tris-HCl pH 8.0, 250 mM LiCl, 0.5% NP-40, 1 mM EDTA, 0.1% sodium deoxycholate), and TE buffer (10 mM Tris-HCl, pH 8.0, and 1 mM EDTA). The protein-DNA complexes were eluted from beads using ChIP Elution buffer (50 mM Tris-HCl pH 7.5, 10 mM EDTA, 1% SDS) for 15 min at 65 °C and the crosslinking was reversed by incubation overnight with proteinase K. The fragment DNA was extracted with phenol:chloroform:isoamyl alcohol (25:24:1), precipitated with ethanol, and resuspended in TE buffer. The immunoprecipitated DNA was used as a template for qPCR. The primers used here are listed in Supplementary file 1.
Yeast two-hybrid assays
Request a detailed protocolThe CDSs of OsGLK1/2 and OsNF-YB7 were cloned into pGADT7 and pGBKT7, respectively. The constructs were cotransformed into yeast strain AH109 using Frozen-EZ Yeast Transformation II kit (Zymo), in accordance with the manufacturer’s instructions. The empty pGADT7 and pGBKT7 vectors were cotransformed in parallel as negative controls. The transformants were first selected on synthetic dropout medium (SD/-Leu-Trp) plates. We tested protein-protein interactions using selective SD/-Leu-Trp-His dropout medium. Interactions were observed after 3 days of incubation at 28 °C. The primers used for generating these constructs are listed in Supplementary file 1.
Split complementary LUC assays
Request a detailed protocolSplit complementary LUC assays were performed as previously described (Niu et al., 2020). The CDSs of OsGLK1/2 and OsNF-YB7 were cloned into JW771 and JW772 vectors to generate nLUC-OsGLK1/2 and cLUC-OsNF-YB7, respectively. The constructs were introduced into Agrobacterium tumefaciens strain GV3101 and then co-infiltrated into N. benthamiana leaves, and the LUC activities were analyzed after 48 hr of infiltration using Tanon Imaging System (5200 Multi; Tanon). The primers used for vector construction are shown in Supplementary file 1.
Bimolecular fluorescence complementation assays
Request a detailed protocolThe CDSs of OsNF-YB7 and OsGLK1 were cloned into the vector pSPYNE (nYFP) and pSPYCE (cYFP), respectively. The prepared plasmids were transformed into Agrobacterium strain GV3101, and the indicated transformant pairs were infiltrated into N. benthamiana leaves. Forty-eight hours after infiltration, the fluorescence signal of yellow fluorescent protein (YFP) was observed with a confocal laser scanning microscope (CLSM) (Carl Zeiss, LSM 710). Images were captured at 514 nm laser excitation and 519–620 nm emission for YFP. The primers used for vector construction are shown in Supplementary file 1.
Co-immunoprecipitation (Co-IP) assays
Request a detailed protocolCo-IP assays were performed using rice protoplast as described previously (Zhang et al., 2011). The CDSs (stop codons removed) of OsGLK1 and OsNF-YB7 were cloned into the vectors pUC19-35S-FLAG-RBS and pJIT163-GFP driven by a 35 S promoter, respectively. Ten micrograms of plasmid DNA (OsGLK1-GFP, GFP, and OsNF-YB7-Flag) was transformed or cotransformed into 200 μl of protoplasts and incubated in WI buffer (0.5 M mannitol, 20 mM KCl, and 4 mM MES at pH 5.7) for 12 hr. The protoplasts were collected and lysed in 500 μl of lysis buffer (50 mM Tris-HCl, 150 mM NaCl, 5 mM EDTA [pH 8.0], 1% NP-40, 0.1 mM PMSF). The extracts were incubated with GFP-Trap agarose beads at 4 °C for 3 hr and washed three times with washing buffer. Samples were boiled in SDS protein loading buffer. Immunoblots were detected using corresponding primary antibodies (anti-GFP, ABclonal no. AE012; anti-Flag, Sigma no. F3165). The primers used for vector construction are shown in Supplementary file 1.
Chl measurement and confocal imaging
Request a detailed protocolApproximately one hundred micrograms of embryos of the indicated genotypes were extracted in 1 ml of 100% dimethyl sulfoxide (DMSO) with incubation at 65 °C for 1 hr. Then, the absorbance values at wavelengths of 648.2 and 664.9 nm were measured by spectrophotometry and total Chl content was calculated as reported previously (Barnes et al., 1992). Chl autofluorescence signal was detected by CLSM (Carl Zeiss, LSM 710), with excitation and emission wavelengths of 633 and 625–730 nm, respectively.
Electrophoretic mobility-shift assays (EMSAs)
Request a detailed protocolOsNF-YB7 CDS was amplified by PCR and cloned into pET-28a vector to generate the OsNF-YB7-His construct. The full-length CDS of OsGLK1 was cloned into pMAL-c5X vector to generate OsGLK1-MBP construct. All constructs were transformed into E. coli strain BL21 to produce recombinant proteins. The promoter subfragments of OsPORA (42 bp, from –278––237) and OsLHCB4 (38 bp, from –259––222) were PCR amplified and labeled with biotin at the 3’ hydroxyl end of the double strands using EMSA Probe Biotin Labeling Kit (Beyotime, GS008). EMSA was performed using EMSA/Gel-Shift kit (Beyotime, GS009), in accordance with the manufacturer’s instructions. The labeled probes were detected in accordance with the instructions provided with the EMSA/Gel-Shift kit. All oligonucleotides used to generate the biotin-labeled probes are listed in Supplementary file 1.
Transmission electron microscopy (TEM)
Request a detailed protocolTEM analysis was performed as described previously (Cheng et al., 2021). Briefly, embryos of WT and osnf-yb7 were fixed overnight at 4 °C in 2.5% glutaraldehyde and 0.1 M PBS. The samples were subsequently washed three times with 0.1 M PBS and then fixed with 1% osmic acid for 4 hr. The samples were dehydrated in a series of ethanol and embedded in acrylic resin at 37 °C for 12 hr and at 60 °C for 48 hr. The samples were sectioned at 100 nm and observed by TEM (TECNAI 12).
Data availability
Sequencing data have been deposited in NCBI BioProject under the accession code PRJNA998591.
-
NCBI BioProjectID PRJNA998591. OsNF-YB7 inactivates OsGLK1 to prevent chlorophyll biosynthesis in rice embryo.
-
NCBI Gene Expression OmnibusID GSE220115. GLK ChIP-seq and ATAC-seq data in Arabidopsis, Tobacco, Tomato, Rice and Maize.
-
NCBI Gene Expression OmnibusID GSE179596. ChIP-seq of OsLEC1.
References
-
A reappraisal of the use of DMSO for the extraction and determination of chlorophylls A and b in lichens and higher plantsEnvironmental and Experimental Botany 32:85–100.https://doi.org/10.1016/0098-8472(92)90034-Y
-
LEC1 (NF-YB9) directly interacts with LEC2 to control gene expression in seedBiochimica et Biophysica Acta. Gene Regulatory Mechanisms 1861:443–450.https://doi.org/10.1016/j.bbagrm.2018.03.005
-
The taxonomic significance of chlorophyllous embryos in angiosperm seedsBotaniska Notiser 133:337–341.
-
agriGO: a GO analysis toolkit for the agricultural communityNucleic Acids Research 38:W64–W70.https://doi.org/10.1093/nar/gkq310
-
Rice leafy cotyledon1 hinders embryo greening during the seed developmentFrontiers in Plant Science 13:887980.https://doi.org/10.3389/fpls.2022.887980
-
Biogenesis and homeostasis of chloroplasts and other plastidsNature Reviews. Molecular Cell Biology 14:787–802.https://doi.org/10.1038/nrm3702
-
CCAAT-box binding transcription factors in plants: Y so many?Trends in Plant Science 18:157–166.https://doi.org/10.1016/j.tplants.2012.07.004
-
Rice fluorescent1 is involved in the regulation of chlorophyllPlant and Cell Physiology 60:2307–2318.https://doi.org/10.1093/pcp/pcz129
-
Cereal endosperms: development and storage product accumulationAnnual Review of Plant Biology 73:255–291.https://doi.org/10.1146/annurev-arplant-070221-024405
-
Overexpression of maize GOLDEN2 in rice and maize calli improves regeneration by activating chloroplast developmentScience China. Life Sciences 66:340–349.https://doi.org/10.1007/s11427-022-2149-2
-
Leafy cotyledon mutants of arabidopsisThe Plant Cell 6:1049–1064.https://doi.org/10.1105/tpc.6.8.1049
-
A study of the pigments produced in darkness by certain green algaePlant Physiology 15:575–588.https://doi.org/10.1104/pp.15.4.575
-
OsbZIP76 interacts with OsNF-YBs and regulates endosperm cellularization in rice (Oryza sativa)Journal of Integrative Plant Biology 62:1983–1996.https://doi.org/10.1111/jipb.12989
-
Chloroembryos: A unique photosynthesis systemJournal of Plant Physiology 170:1131–1138.https://doi.org/10.1016/j.jplph.2013.04.011
-
Embryonic photosynthesis affects post-germination plant growthPlant Physiology 182:2166–2181.https://doi.org/10.1104/pp.20.00043
-
Photosynthesis in non-foliar tissues: implications for yieldThe Plant Journal 101:1001–1015.https://doi.org/10.1111/tpj.14633
-
Photosynthesis in the seeds of chloroembryophytesRussian Journal of Plant Physiology 63:1–12.https://doi.org/10.1134/S1021443715060163
-
The stay-green traitJournal of Experimental Botany 65:3889–3900.https://doi.org/10.1093/jxb/eru037
-
Chlorophyll biogenesis sees the lightNature Plants 7:380–381.https://doi.org/10.1038/s41477-021-00900-6
-
OsLEC1/OsHAP3E participates in the determination of meristem identity in both vegetative and reproductive developments of riceJournal of Integrative Plant Biology 55:232–249.https://doi.org/10.1111/jipb.12025
-
Integrative analysis of reference epigenomes in 20 rice varietiesNature Communications 11:2658.https://doi.org/10.1038/s41467-020-16457-5
-
A group of nuclear factor Y transcription factors are sub-functionalized during endosperm development in monocotsJournal of Experimental Botany 69:2495–2510.https://doi.org/10.1093/jxb/ery087
Article and author information
Author details
Funding
National Natural Science Foundation of China (32170344)
- Chen Chen
National Natural Science Foundation of China (32300689)
- Zongju Yang
Project of Zhongshan Biological Breeding Laboratory (BM2022008-02)
- Chen Chen
Natural Science Foundation of the Higher Education Institutions of Jiangsu Province (21KJB21003)
- Zongju Yang
China Postdoctoral Science Foundation (2022M712702)
- Zongju Yang
Jiangsu Province Government (JBGS001)
- Chen Chen
Priority Academic Program Development of Jiangsu Higher Education Institutions (PAPD)
- Chen Chen
Independent Scientific Research Project Funds of the Jiangsu Key Laboratory of Crop Genomics and Molecular Breeding (PLR202101)
- Chen Chen
The funders had no role in study design, data collection and interpretation, or the decision to submit the work for publication.
Acknowledgements
We thank Prof. Hengxiu Yu and Dr. Chao Zhang of Yangzhou University for kindly providing the positive and negative control vectors for dual luciferase reporter assays. This research was supported by grants from the National Natural Science Foundation of China (32170344, 32300689), the Project of Zhongshan Biological Breeding Laboratory (BM2022008-02), the Jiangsu Province Government (JBGS001), the Natural Science Foundation of the Higher Education Institutions of Jiangsu Province (21KJB21003), the China Postdoctoral Science Foundation (2022M712702), the Independent Scientific Research Project Funds of the Jiangsu Key Laboratory of Crop Genomics and Molecular Breeding (PLR202101), the Priority Academic Program Development of Jiangsu Higher Education Institutions (PAPD).
Version history
- Preprint posted:
- Sent for peer review:
- Reviewed Preprint version 1:
- Reviewed Preprint version 2:
- Version of Record published:
Cite all versions
You can cite all versions using the DOI https://doi.org/10.7554/eLife.96553. This DOI represents all versions, and will always resolve to the latest one.
Copyright
© 2024, Yang, Bai, E et al.
This article is distributed under the terms of the Creative Commons Attribution License, which permits unrestricted use and redistribution provided that the original author and source are credited.
Metrics
-
- 948
- views
-
- 307
- downloads
-
- 3
- citations
Views, downloads and citations are aggregated across all versions of this paper published by eLife.
Download links
Downloads (link to download the article as PDF)
Open citations (links to open the citations from this article in various online reference manager services)
Cite this article (links to download the citations from this article in formats compatible with various reference manager tools)
Further reading
-
- Plant Biology
- Structural Biology and Molecular Biophysics
The Calvin-Benson-Bassham cycle (CBBC) performs carbon fixation in photosynthetic organisms. Among the eleven enzymes that participate in the pathway, sedoheptulose-1,7-bisphosphatase (SBPase) is expressed in photo-autotrophs and catalyzes the hydrolysis of sedoheptulose-1,7-bisphosphate (SBP) to sedoheptulose-7-phosphate (S7P). SBPase, along with nine other enzymes in the CBBC, contributes to the regeneration of ribulose-1,5-bisphosphate, the carbon-fixing co-substrate used by ribulose-1,5-bisphosphate carboxylase/oxygenase (Rubisco). The metabolic role of SBPase is restricted to the CBBC, and a recent study revealed that the three-dimensional structure of SBPase from the moss Physcomitrium patens was found to be similar to that of fructose-1,6-bisphosphatase (FBPase), an enzyme involved in both CBBC and neoglucogenesis. In this study we report the first structure of an SBPase from a chlorophyte, the model unicellular green microalga Chlamydomonas reinhardtii. By combining experimental and computational structural analyses, we describe the topology, conformations, and quaternary structure of Chlamydomonas reinhardtii SBPase (CrSBPase). We identify active site residues and locate sites of redox- and phospho-post-translational modifications that contribute to enzymatic functions. Finally, we observe that CrSBPase adopts distinct oligomeric states that may dynamically contribute to the control of its activity.
-
- Plant Biology
Soil salinity is one of the major threats to agricultural productivity worldwide. Salt stress exposure alters root and shoots growth rates, thereby affecting overall plant performance. While past studies have extensively documented the effect of salt stress on root elongation and shoot development separately, here we take an innovative approach by examining the coordination of root and shoot growth under salt stress conditions. Utilizing a newly developed tool for quantifying the root:shoot ratio in agar-grown Arabidopsis seedlings, we found that salt stress results in a loss of coordination between root and shoot growth rates. We identify a specific gene cluster encoding domain-of-unknown-function 247 (DUF247), and characterize one of these genes as Salt Root:shoot Ratio Regulator Gene (SR3G). Further analysis elucidates the role of SR3G as a negative regulator of salt stress tolerance, revealing its function in regulating shoot growth, root suberization, and sodium accumulation. We further characterize that SR3G expression is modulated by WRKY75 transcription factor, known as a positive regulator of salt stress tolerance. Finally, we show that the salt stress sensitivity of wrky75 mutant is completely diminished when it is combined with sr3g mutation. Together, our results demonstrate that utilizing root:shoot ratio as an architectural feature leads to the discovery of a new stress resilience gene. The study’s innovative approach and findings not only contribute to our understanding of plant stress tolerance mechanisms but also open new avenues for genetic and agronomic strategies to enhance crop environmental resilience.