A genome-wide CRISPR/Cas9 screen identifies calreticulin as a selective repressor of ATF6α
Figures
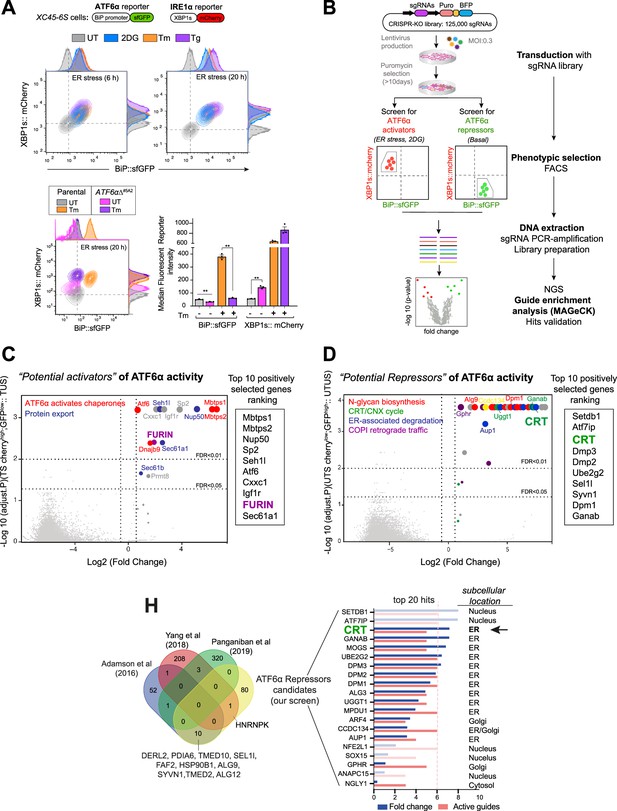
High-throughput CRISPR/Cas9 screens to identify new modulators of ATF6α signalling.
(A) Characterisation of the XC45-6S cells, a dual unfolded protein response (UPR) reporter CHO-K1 cell line stably expressing XBP1s::mCherry to report on IRE1 activity and BiP::sfGFP to report on ATF6α activity was generated to perform the CRISPR/Cas9 screens. Top panel: Two-dimensional contour plots of BiP::sfGFP and XBP1s::mCherry signals in untreated cells (UT, grey) and cells treated with the endoplasmic reticulum (ER) stressors 2-deoxy-D-glucose (2DG, 4 mM, blue), tunicamycin (Tm, 2.5 µg/ml, orange), or thapsigargin (Tg, 0.5 µM, purple) over a short (6 hr) and long period of time (20 hr). Histograms of the signal in each channel are displayed on the oppsite axes. Lower panel: Contour plots as in ‘A, top panel’ from XC45-6S cells (parental) and a knockout ATF6αΔ clone (ATF6αΔ#5A2) generated by CRISPR/Cas9 gene editing. Cells were analysed under basal (UT) and ER stress conditions (Tm, 2.5 µg/ml, 20 hr). The bar graph shows the mean ± SEM of the median fluorescent reporter intensity normalised to untreated cells from three independent experiments. Statistical analysis was performed by a two-sided unpaired Welch’s t-test and significance is indicated by asterisks (**p < 0.01). (B) Flowchart illustrating the two parallel CRISPR/Cas9 screenings aimed to identify genetic ATF6α regulators. ATF6α/IRE1 dual reporter cells were transduced with a CHO sgRNA CRISPR knockout (KO) library. After puromycin selection, the transduced population was split into two subpopulations, subjected to either ER stress with 2DG treatment (4 mM) for 18 hr or left untreated (basal) to identify the activators and repressors of ATF6α, respectively. A BiP::sfGFPlow;XBP1s::mCherryhigh population under ER stress and a BiP::sfGFPhigh; XBP1s::mCherrylow population devoid of ER stress were collected through fluorescent-activated cell sorting (FACS) and processed to determine sgRNAs frequencies after two rounds of selection, expansion, and enrichment. (C) Volcano plot depicting the Log2 (fold-change) and the negative Log10 (adjusted p-value) of the genes targeted by sgRNAs in 2DG-treated-sorted (TS) cells. This analysis identified genes whose loss confers repression of the ATF6α reporter during ER stress without impacting ER-stress-induced IRE1, compared to treated-unsorted (TUS) cells. The table lists the top 10 genes positively selected in the ATF6α activator screen, with FURIN highlighted as a hit for further analysis. (D) Volcano plot, as in ‘C’, depicting the genes targeted by sgRNAs in untreated-sorted (UTS) cells. This analysis identified genes whose loss confers activation of the ATF6α reporter under basal condition, compared to untreated-unsorted (UTUS) cells. The table lists the top 10 genes positively selected in the ATF6α repressor screen, with calreticulin (CRT) highlighted as the hit for further focussed analysis. (H) Venn diagram depicting unique and common upregulated top genes examined in ‘D’, between our screen (yellow) and previous high-throughput UPR screenings (Adamson et al., 2016; Panganiban et al., 2019; Yang et al., 2018) to discard hits that were confirmed to be IRE1- or PERK-dependent UPR target genes. The Log2 (fold-change) and the number of active sgRNA are provided for the 20 genes that remained in our screen, to be put forth as possible ATF6α repressor candidates.
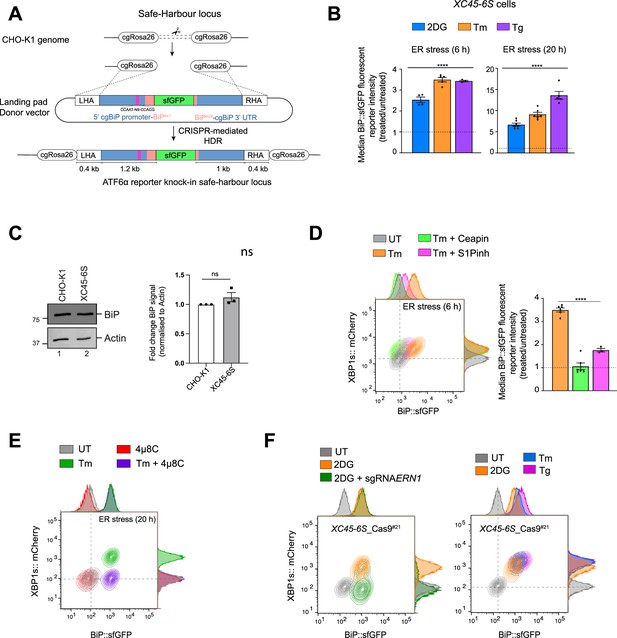
Characterisation of the ATF6α/IRE1 dual unfolded protein response (UPR) reporter cell (XC45-6S).
(A) Schematic representation of the strategy for targeted integration at the cgROSA26 safe-harbour locus in the CHO genome, mediated by CRISPR/Cas9-mediated homology-directed repair (HDR). The approach involves a unique sgRNA/Cas9 sequence targeting the cgROSA26 locus (Gaidukov et al., 2018) and a landing pad donor plasmid containing homology arms (LHA and RHA) flanking a minigene encoding the promoter region of the cgBiP fused with the superfolder green fluorescent protein (sfGFP) to create a BiP::sfGFP reporter to monitor ATF6α activity in cells. (B) Related to Figure 1A, top panel. Quantification of fold-change BiP::sfGFP signals in XC45-6S dual UPR reporter cells under basal conditions (untreated) and treated with the endoplasmic reticulum (ER) stressors 2-deoxy-D-glucose (2DG), Tm, and Tg (6 or 20 hr). Shown is the normalised (treated/untreated) median fluorescence intensity (MFI) as mean ± standard error of the mean (SEM) of independent repetitions (n > 3). (C) Representative immunoblots of endogenous BiP protein levels in cell lysates from parental CHO-K1 cells and ATF6α/IRE1 dual UPR reporter cells (XC45-6S). The samples were also blotted for actin (loading control). The right graph bar shows the fold-change of BiP signal normalised to the signal of the loading control in three independent experiments indicated by mean ± SEM. (D) Two-dimensional contour plots of BiP::sfGFP and XBP1s::mCherry levels in XC45-6S cells under basal conditions (UT, grey) or after treatment with tunicamycin alone (2.5 µg/ml, Tm, orange) or in combination with Ceapin A7 (5 µM, green) or S1P inhibitor (15 µM, pink) for 6 hr. Histograms of the signal in each channel are displayed on the axes. The bar graph shows the mean ± SEM of the normalised median BiP::sfGFP reporter intensity from more than four independent experiments. (E) Contour plot, as in ‘D’, from parental cells treated with tunicamycin alone (Tm, 2.5 µg/ml, green) or in combination with the IRE1 inhibitor 4µ8C (10 µM, purple) for 18 hr. A representative dataset from two independent experiments is shown. (F) Characterisation of the ATF6α/IRE1 dual UPR reporter cell line used in the CRISPR/Cas9 screen after stable integration of Cas9. Left panel: Contour plots to confirm Cas9 stable integration. Cells were analysed under basal conditions (grey) or after treatment with 2DG alone (orange) or transfection with an individual lentiGuide-Puro plasmid (without expression of Cas9) targeting exon 18 of IRE1 (encoded by cgERN1, green). ER stress treatments with 4 mM 2DG lasted 24 hr. Right panel: As in ‘left panel’, but cells were treated with three different ER stressors for 20 hr: 2DG (4 mM), Tm (2.5 µg/ml), or Tg (0.5 µM). A representative dataset from two independent experiments is shown. All statistical analysis was performed by a two-sided unpaired Welch’s t-test and significance is indicated by asterisks (****p < 0.0001; ns: non-significant).
-
Figure 1—figure supplement 1—source data 1
Raw images for gels shown in Figure 1—figure supplement 1.
- https://cdn.elifesciences.org/articles/96979/elife-96979-fig1-figsupp1-data1-v1.zip
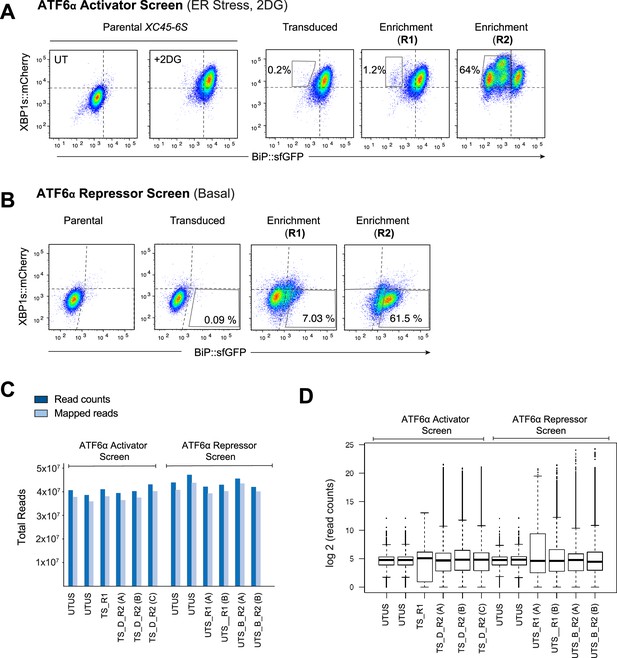
Enrichment method and quality control data analysis by MAGeCK of the CRISPR/Cas9 screens.
(A) Two-dimensional contour plots of BiP::sfGFP and XBP1s::mCherry signals in XC45-6S obtained after each sort of enrichment (R1: round 1; R2: round 2) in the screen designed to identify ATF6α activators. The plots reveal a progressive enrichment of cells displaying CRISPR/Cas9-induced genetic perturbations that specifically impairing BiP::sfGFP activation upon unfolded protein response (UPR) induction with 2-deoxy-D-glucose (2DG) (4 mM). (B) Contour plots, as in ‘A’, representing the screen designed to identify ATF6α repressors by successive rounds of enrichments. The focus is on cells exhibiting CRISPR/Cas9-induced genetic perturbations that lead to the constitutive activation of the BiP::sfGFP reporter under basal conditions, while leaving the XBP1s::mCherry reporter unaffected. (C) Analysis of total read counts and mapped reads to the CHO library analysed by MAGeCK [UTUS: untreated and unsorted; TS: treated (plus 2DG) and sorted; R1: round 1 of enrichment; R2: round 2 of enrichment; D: dull green fluorescent protein (GFP) population; B: bright GFP population; A–C: pools of cells]. (D) Frequency distribution of sgRNA in each sample, showing the median-normalised read counts.
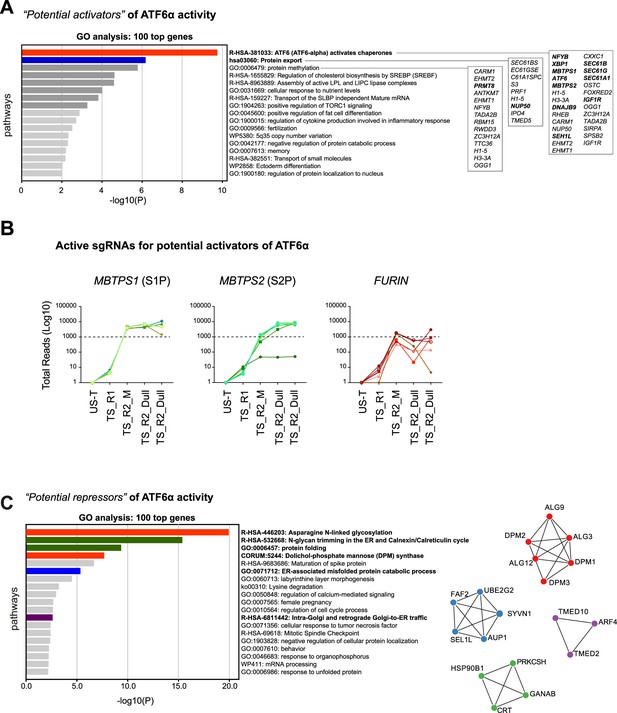
Integration of CRISPR screen hits.
(A) Pathway enrichment analysis for biological processes using Gene Ontology (GO) terms for the top 100 hits identified in the CRISPR/Cas9 screen aimed to identify potential activators of ATF6α signalling. Selected pathways are ranked by p-value. (B) Total reads count enrichment through the selection process for each active sgRNA targeting the most significant genes identified in the activator ATF6α screen, including known regulators of ATF6α (MBTPS1-S1P and MBPTPS2-S2P) and those with possible roles (FURIN) in the activation of ATF6α signalling. (C) Pathway enrichment analysis, as in ‘A’, for the top 100 hits identified in the CRISPR/Cas9 screen aimed to identify potential repressors of ATF6α signalling. Selected pathways are ranked by p-value. On the left, a protein–protein interaction network (Metascape) for the 100 top hits identified in the CRISPR/Cas9 screen for potential repressors of ATF6α signalling is shown.
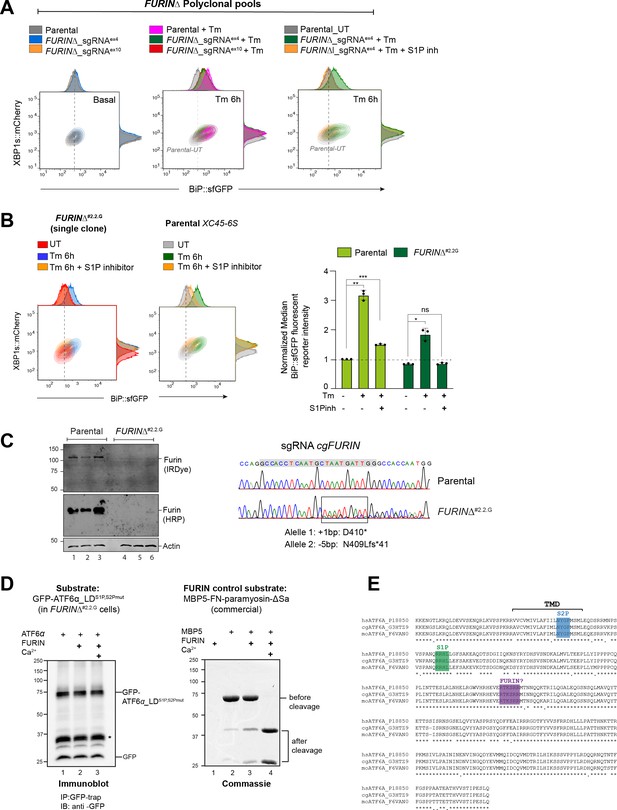
FURIN depletion decreases responsiveness to ATF6α activation upon endoplasmic reticulum (ER) stress.
(A) Two-dimensional contour plots of BiP::sfGFP and XBP1s::mCherry signals in a sorted pooled population with FURIN depletion through CRISPR/Cas9 sgRNAs targeting exons 4 and 10. The analysis was conducted under basal conditions (left panel), after tunicamycin (Tm)-ER stress induction alone for 6 hr (middle panel) or in combination with S1P inhibitor treatment (15 µM) (right panel). Parental cells were used as a reference. A representative dataset from more than three experiments is shown. (B) Contour plots as in ‘A’ using parental cells and a single FURIN∆ clone (#2.2.G). The cells were assessed under basal conditions (UT) or after treatment with tunicamycin alone (Tm, 2.5 µg/ml) or in combination with the S1P inhibitor (15 µM) for 6 hr. The bar graph shows the mean ± standard error of the mean (SEM) of the normalised median BiP::sfGFP reporter intensity from three independent experiments. All statistical analysis was performed by a two-sided unpaired Welch’s t-test and significance is indicated by asterisks (*p < 0.05; **p < 0.01; ***p < 0.001; ns: non-significant). (C) Immunoblot of whole-cell lysates from the indicated genotyped probed with antibodies against FURIN and the loading control actin. FURIN protein levels were determined using horseradish peroxidase (HRP) or IRDye fluorescently labelled secondary antibodies. On the right, DNA sequence chromatograms of FURIN-targeted region are represented, showcasing parental cells and an FURIN∆ clone. The rectangle indicates where the FURIN indel mutation occurs, with the sgRNA/Cas9 sequence indicated in a grey rectangle. (D) FURIN cleavage assay. Left panel: FURIN∆ cells were transfected with a plasmid encoding GFP-ATF6α_TMS1P,S2P mut-LDWT to be used as substrate for in vitro FURIN cleavage reactions. Forty-eight hours after transfection, cells were lysed, ATF6α was immunoprecipitated (IP) using GFP-Trap Agarose and then subjected to FURIN cleavage by incubating with 1 U of commercial FURIN (#P8077S, NEB) for 6 hr at 25°C in the presence or absence of calcium (Ca2+). Beads were eluted in 2xLB-DTT, boiled for 5 min at 90°C and loaded into 10% sodium dodecyl sulfate–polyacrylamide gel electrophoresis (SDS–PAGE) gels. Membranes were immunoblotted (IB) with an anti-GFP antibody. The expected molecular weight of GFP-ATF6α_TM-S1P,S2P mut-LDWT before cleavage is 67 and 45 kDa post-FURIN cleavage. Right panel: As in left panel but using an FURIN control substrate (MBP5-FN-paramyosin-ΔSa). MBP5FN-paramyosin-ΔSa (2.5 µg) was also incubated for 6 hr at 25°C in the presence or absence of calcium with 1 U of FURIN. The molecular weight of the MBP5-FN-paramyosin-ΔSal substrate before cleavage is 70.6 kDa. FURIN cleavage resulted in an MBP5 mass of 42.8 kDa and a mass of 27.8 kDa. All samples were subjected to SDS–PAGE, and bands were detected by Coomassie blue staining. (E) Amino acid sequence homology between residues 349 and 670 of human (hs), hamster (cg), and mouse (mo) ATF6α indicating the transmembrane domain (TMD) and the cleavage sites for S1P and S2P proteases. The FURIN putative cleavage site is highlighted in purple. The alignment was performed using the MacVector programme.
-
Figure 1—figure supplement 4—source data 1
Raw images for gels shown in Figure 1—figure supplement 4.
- https://cdn.elifesciences.org/articles/96979/elife-96979-fig1-figsupp4-data1-v1.zip
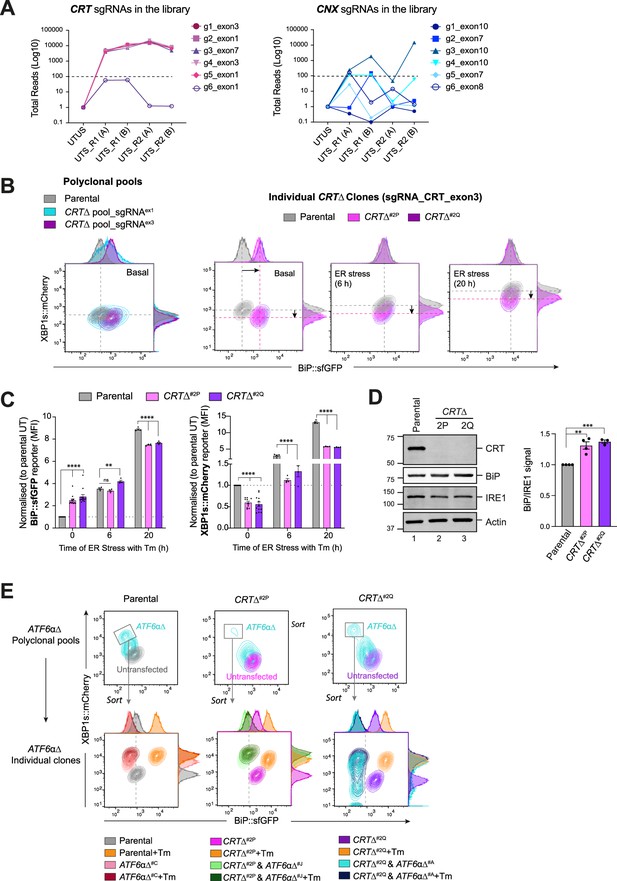
Calreticulin (CRT)-depleted cells exhibit constitutive activation of ATF6α signalling.
(A) Total read count enrichment through the selection process for each active sgRNA targeting CRT and calnexin (CNX) [UTUS: untreated and unsorted; UTS: untreated and sorted; R1: round 1 of enrichment; R2: round 2 of enrichment; A–C: pools of cells; g1–g6: sgRNAs]. (B) Two-dimensional contour plots of BiP::sfGFP and XBP1s::mCherry signals examined in two derivative CRTΔ polyclonal pools (left) and two independent CRTΔ clones (right, named CRTΔ#2P and CRTΔ#2Q) under basal conditions or endoplasmic reticulum (ER) stress induced with 2.5 µg/ml Tm for a short (6 hr) and extended period of time (20 hr). A representative dataset from more than four independent experiments is shown. (C) Normalised quantification expressed as fold-change of the median fluorescence intensity (MFI) of BiP::sfGFP (left) and XBP1s::mCherry signals (right) in the two independent CRTΔ clones from more than four independent experiments, as described in ‘B’, indicated by mean ± standard error of the mean (SEM). (D) Representative immunoblots of endogenous CRT and BiP protein levels in cell lysates from XC45-6S parental cells and two CRTΔ derivatives clones selected for functional experiments. The samples were also blotted for IRE1 and actin (loading controls). The right graph bar shows the ratio of BiP to IRE1 signal in four independent experiments indicated by mean ± SEM. (E) Top panel: Two-dimensional contour plots of BiP::sfGFP and XBP1s::mCherry signals in polyclonal pools of parental cells and two CRTΔ clones after depleting the endogenous ATF6α locus by CRISPR/Cas9 gene editing. Lower panel: Grey rectangles in top panels indicate the subcellular polyclonal population re-sorted to analyse single ATF6αΔ clones in parental and CRTΔ clones. ER stress treatments with 2.5 µg/ml Tm lasted 20 hr. All statistical analysis was performed by two-sided unpaired Welch’s t-test and significance is indicated by asterisks (**p < 0.01; ***p < 0.001; ****p < 0.0001).
-
Figure 2—source data 1
Raw images for gels shown in Figure 2.
- https://cdn.elifesciences.org/articles/96979/elife-96979-fig2-data1-v1.zip
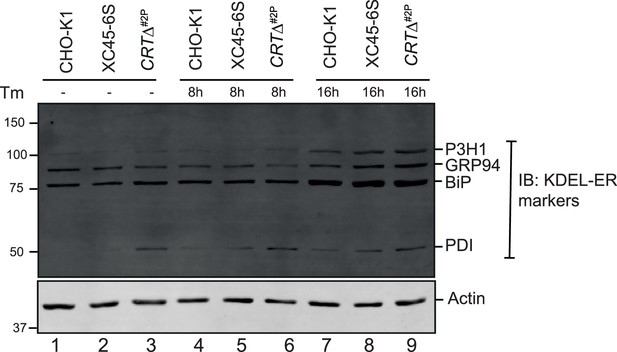
ER-resident proteins (with a KDEL-COOH tag) in calreticulin (CRT)-depleted cells.
Representative immunoblots from two independent experiments show the expression levels of endogenous KDEL (Lys-Asp-Glu-Leu) receptors in cell lysates from plain CHO-K1 cells, XC45-6S dual unfolded protein response (UPR) reporter cells and a derivative CRTΔ clone (2P). The samples were also blotted for actin as a loading control.
-
Figure 2—figure supplement 1—source data 1
Raw images for gels shown in Figure 2—figure supplement 1.
- https://cdn.elifesciences.org/articles/96979/elife-96979-fig2-figsupp1-data1-v1.zip
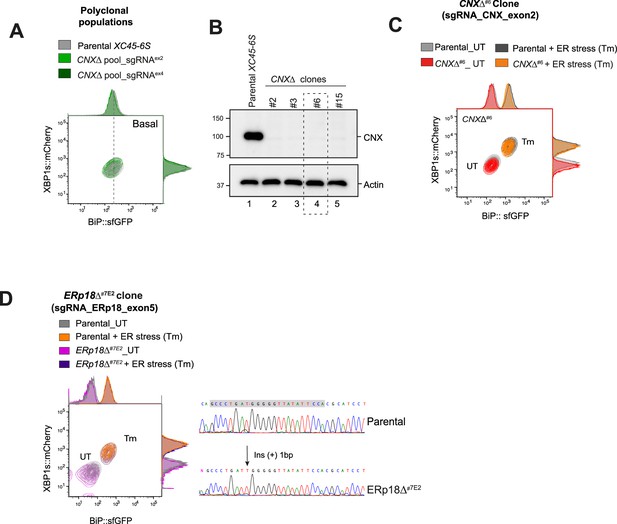
Calnexin and ERp18 depletion do not deregulate ATF6α pathway.
(A) Two-dimensional contour plots of BiP::sfGFP and XBP1s::mCherry signals in basal conditions in sorted pooled populations where calnexin (CNX) was depleted by CRISPR/Cas9 sgRNAs targeting exons 2 and 4 (in green) and in parental XC45-6S cells. (B) Immunoblot depicting CNX protein levels in whole-cell lysates from parental cells and four CNX∆ derivatives clones. The CNX∆#6 clone (highlighted into a rectangle dashed box) was selected for subsequent functional experiments in ‘C’. Actin was used as a loading control marker. (C) Contour plot, as in ‘A’, from parental cells and the CNX∆#6 single clone treated with Tm for 20 hr (black and orange) or left untreated (grey and red). (D) Left panel: Contour plot, as in ‘C’, from parental cells and the ERp18∆#7E2 single clone treated with Tm for 20 hr (orange and purple) or left untreated (grey and pink). Right panel: DNA sequence chromatograms of ERp18-targeted region, in parental cells and an ERp18∆ clone with the arrow indicating the peak where the ERp18 indel mutation occurs. sgRNA Cas9 sequence is indicated with a grey rectangle.
-
Figure 2—figure supplement 2—source data 1
Raw images for gels shown in Figure 2—figure supplement 2.
- https://cdn.elifesciences.org/articles/96979/elife-96979-fig2-figsupp2-data1-v1.zip
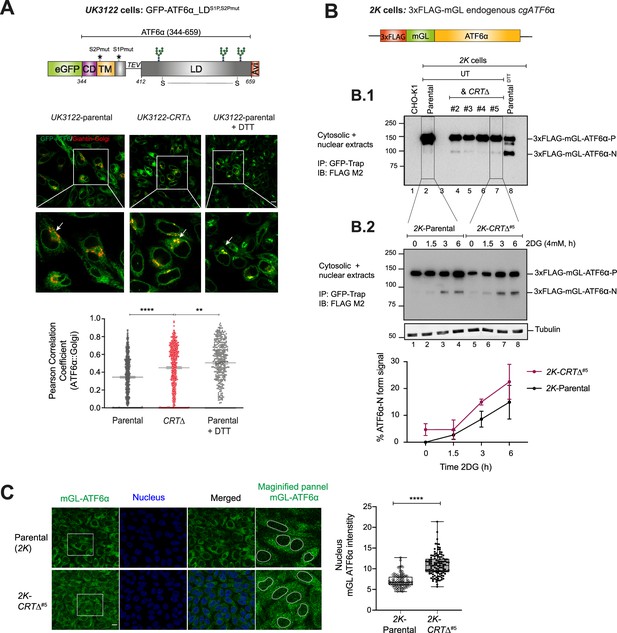
Loss of calreticulin promotes higher proportion of ATF6α trafficking to Golgi and nucleus.
(A) Top panel: Schematic representation of the genetic construct (UK3122) designed for generating a CHO-K1 stable cell line expressing a N-terminus-tagged ATF6α, where eGFP replaces most of the cytosolic domain of cgATF6α, and the S2P and S1P cleavage sites are mutated to increase the intact fraction in the Golgi. Colour code: eGFP tag in green; cytosolic domain (CD) in purple; transmembrane domain (TM) in yellow; Tobacco Etch Virus (TEV) protease cleavage site (ENLYFQ↓G) is represented with a linker line; luminal domain (LD) in grey; and a C-terminus Avi-tag in red. Lower panels: Representative live cell confocal microscopy images of UK3122 CHO-K1 cells stably expressing GFP-ATF6α_LDS1P,S2Pmut and transiently expressing a pmScarlet _Giantin-C1 plasmid as a red fluorescent Golgi marker. Both parental cells and a CRISPR/Cas9 gene edited CRT∆ derivative pool were imaged. Dithiothreitol (DTT) treatment (4 mM, 1 hr) was applied to parental cells to positively influence ATF6α’s Golgi localisation. Each panel’s magnified sections are presented in the insets. Arrows indicate GFP-ATF6α_LDS1P,S2Pmut::Golgi co-localisation. Scale bar: 10μm. Pearson coefficients for the co-localisation of GFP-ATF6α_LDS1P,S2Pmut with the Golgi apparatus marker Giantin in parental cells (n > 150), parental cells treated with 4 mM DTT for 1 hr (n > 100) and CRT∆ cells (n > 150) are presented in the bar graph, including cells from three independent experiments. Volocity software was used for co-localisation quantification. Statistical analysis was performed by a two-sided unpaired Welch’s t-test and significance is indicated by asterisks (**p < 0.01; ****p < 0.0001). (B) Schematic representation of engineered stable 2K cells with the endogenous cgATF6α locus (in orange) tagged with 3xFLAG (in red) and mGreenLantern (mGL) (in green) at the N-terminus. (B.1) Cell lysates from 2K cells and four independent 2K-CRT∆ derivative clones were harvested, immunoprecipitated (IP) using GFP-Trap Agarose (ChromoTek) and analysed by immunoblot (IB) using an anti-FLAG M2 antibody to detect processing of ATF6α. Full-length ATF6α-Precursor (ATF6α-P) and processed ATF6α-N forms were identified. Treatment with DTT for 1 hr (4 mM) was used to induce ATF6α processing. Parental untagged cells were used as control for background. Data shown are representative of one experiment. (B.2) Parental 2K cells and a derivative single CRT∆ clone (#5) were treated with 4 mM 2-deoxy-D-glucose (2DG) at the indicated time points (1.5, 3, and 6 hr). Cells were harvested and analysed by immunoblot as in ‘B.1’. Lower panel shows the percentage of ATF6α-N form signal/total ATF6α signal from three independent experiments indicated by mean ± standard error of the mean (SEM). (C) ATF6α nuclear translocation live cell microscopy assay showing unstressed 2K parental cells and a 2K-CRT∆ clone. mGL-ATF6α is in green, and nucleus in blue. mGL-ATF6α signal intensity in the nucleus (shown by dashed lines) was measured using Volocity software. Scale bar: 10μm. Data from parental cells (n > 100) and CRT∆ cells (n > 100) are displayed in a box and whiskers graph, displaying all points (with min. and max. intensities).
-
Figure 3—source data 1
Raw images for gels shown in Figure 3.
- https://cdn.elifesciences.org/articles/96979/elife-96979-fig3-data1-v1.zip
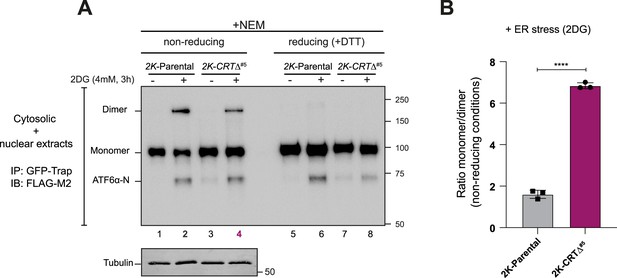
Analyses of ATF6α redox status in calreticulin (CRT)-depleted cells.
(A) Whole-cell lysates from stable 2K cells with the endogenous cgATF6α locus tagged with 3xFLAG and mGreenLantern (mGL) and a derivative 2K-CRT∆ clone (#5) were separated under non-reducing (-DTT) and reducing (+50 mM DTT) sodium dodecyl sulfate/polyacrylamide gel electrophoresis (SDS/PAGE) conditions. ATF6α redox status was analysed under basal conditions and following 2-deoxy-D-glucose (2DG) treatment (4 mM, 3 hr) to induce endoplasmic reticulum (ER) stress and ATF6α trafficking. Samples were harvested and lysed in the presence of 20 mM N-ethylmaleimide (NEM), immunoprecipitated (IP) using GFP-Trap Agarose (ChromoTek) and analysed by immunoblot (IB) using an anti-FLAG M2 antibody to detect ATF6α. Shown is a representative immunoblot from three independent repetitions. (B) The graph bar shows the ratio of ATF6αmonomer to ATF6αdimer signals in 2K cells (line 2 from ‘A’) and 2K-CRT∆ clone (line 4 from ‘A’) under stress conditions in non-reducing conditions from three independent experiments indicated as mean ± standard error of the mean (SEM). Statistical analysis was performed by a two-sided unpaired Welch’s t-test and significance is indicated by asterisks (****p < 0.0001).
-
Figure 3—figure supplement 1—source code 1
Raw images for gels shown in Figure 3—figure supplement 1.
- https://cdn.elifesciences.org/articles/96979/elife-96979-fig3-figsupp1-code1-v1.zip
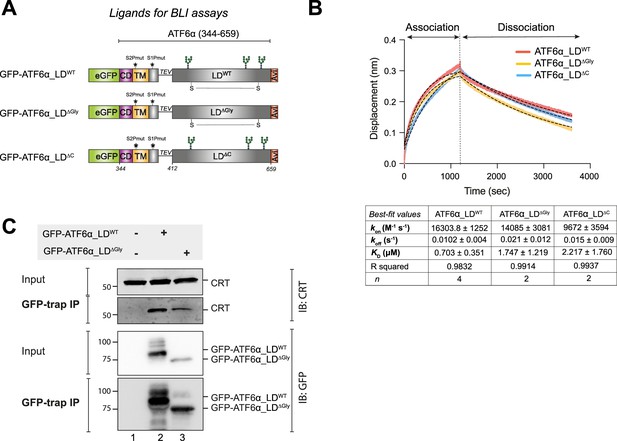
Calreticulin (CRT) interacts directly with the ATF6α luminal domain (LD) in vitro and in cells.
(A) Schematic representation of the constructs designed to produce three LDs of ATF6α to be used as ligands in biolayer interferometry (BLI) assays: wild-type (WT) ATF6α_LD, non-glycosylated (∆Gly) ATF6α_LD, and ATF6α_LD lacking its cysteines (∆C). The probes underwent in vivo biotinylation, purification using GFP-Trap Agarose (ChromoTek), and were eluted via TEV cleavage. Colour code: eGFP tag in green; cytosolic domain (CD) in purple; transmembrane domain (TM) in yellow; TEV cleavage site with a linker line; LD in grey; C-terminus Avi-tag in red. S–S indicates for the disulphide bond in the LD between two cysteines. (B) Representative BLI signals depicting the interaction of rat (r) CRT (analyte) with different forms of cgATF6α_LD (from HEK293T cells) immobilised on streptavidin biosensors: ATF6α_LDWT in red, ATF6α_LD∆Gly in yellow, and ATF6α_LD∆C in blue. The interaction kinetics were calculated over the entire 1200s of the association phase and 2400s of the dissociation phase. Dotted lines represent the best-fit association then dissociation curves using a nonlinear regression to calculate kinetic constants by Prism10. The table represents the BLI measurements of the kinetics of the interaction between rCRT and cgATF6α_LD from two to four independent experiments (means ± standard error of the mean [SEM]). Interaction kinetics were measured at an rCRT concentration of 10 µM. (C) Representative immunoblots (IB) of endogenous CRT recovered in complex with ATF6α in HEK293T cells overexpressing GFP-cgATF6α_LDWT, GFP-cgATF6α_LD∆Gly, or neither. Immunoprecipitations (IP) were performed using GFP-Trap Agarose, and then membranes were blotted with anti-human CRT and anti-GFP antibodies. The immunoblots are representative for three independent experiments.
-
Figure 4—source data 1
Raw images for gels shown in Figure 4.
- https://cdn.elifesciences.org/articles/96979/elife-96979-fig4-data1-v1.zip
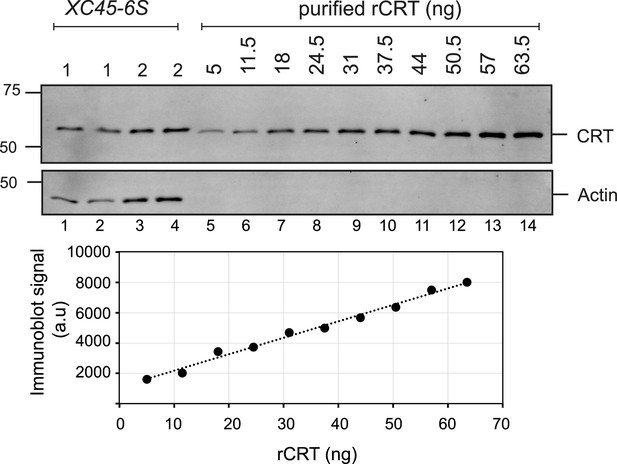
Estimation of calreticulin’s (CRT) physiological abundance by quantitative immunoblotting.
Top panel: Immunoblot showing CRT and actin levels for parental XC45-6S cells and known amounts of purified bacterially expressed rCRT. Lower panel: Calibration curve derived from known quantities of purified rCRT, fitted to a linear function. Assuming the endoplasmic reticulum (ER) constitutes 10% of cell total volume (Alberts et al., 2002), the concentration of CRT in the ER of CHO-K1 cells was estimated to be around 7 µM.
-
Figure 4—figure supplement 1—source data 1
Raw images for gels shown in Figure 4—figure supplement 1.
- https://cdn.elifesciences.org/articles/96979/elife-96979-fig4-figsupp1-data1-v1.zip
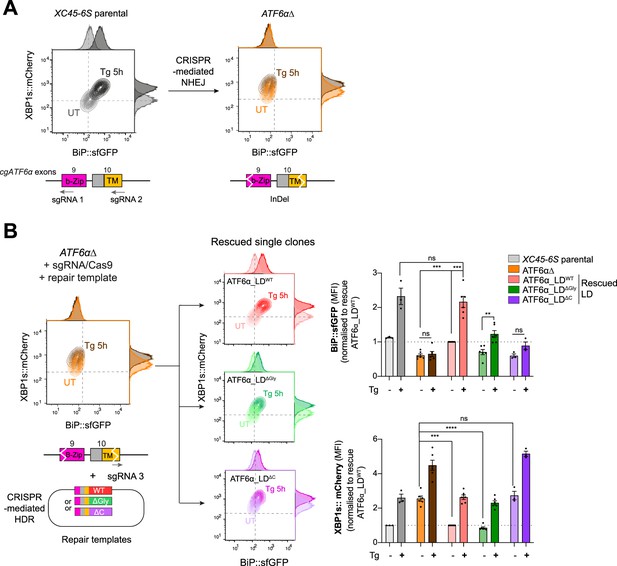
A homologous CRISPR/Cas9 gene editing recombination platform to study ATF6α variants at endogenous levels in CHO-K1 cells.
(A) Two-dimensional contour plots of BiP::sfGFP and XBP1s::mCherry signals in XC45-6S parental cells (in grey; left) and in a null functional ATF6α clonal cell (ATF6α∆, in orange; right) generated by CRISPR/Cas9-mediated NHEJ (non-homologous end joining) to introduce frameshifting mutations into the DNA-binding (b-Zip, pink) and transmembrane (TM, yellow) encoding regions by targeting exons 9 and 10 of the endogenous locus of cgATF6α. Cells were analysed in basal conditions (UT) or upon endoplasmic reticulum (ER) induction with Tg (0.5 µM, 5 hr). A schema for the sgRNAs/Cas9 that target cgATF6 is shown below the plots. (B) ATF6αΔ cells were re-targeted with a sgRNA/Cas9 directed to the mutated exon 10 and offering three repair templates: ATF6α_LDWT in red, ATF6α_LD∆Gly in green, and ATF6α_LD∆C in purple. Successfully rescued single clones by CRISPR/Cas9-mediated homology-directed repair (HDR) were evaluated in the absence (pale colour) and presence of ER stress (0.5 µM Tg, 5 hr, dark colour). Contour plots are representative from three to six independent experiments. Bar graphs display the quantification of the fold-change of BiP::sfGFP (top) and XBP1s::mCherry signals (bottom) in more than three independent experiments indicated by mean ± standard error of the mean (SEM). Statistical analysis was performed by a two-sided unpaired Welch’s t-test and significance is indicated by asterisks (***p < 0.001; ****p < 0.0001; ns: non-significant).
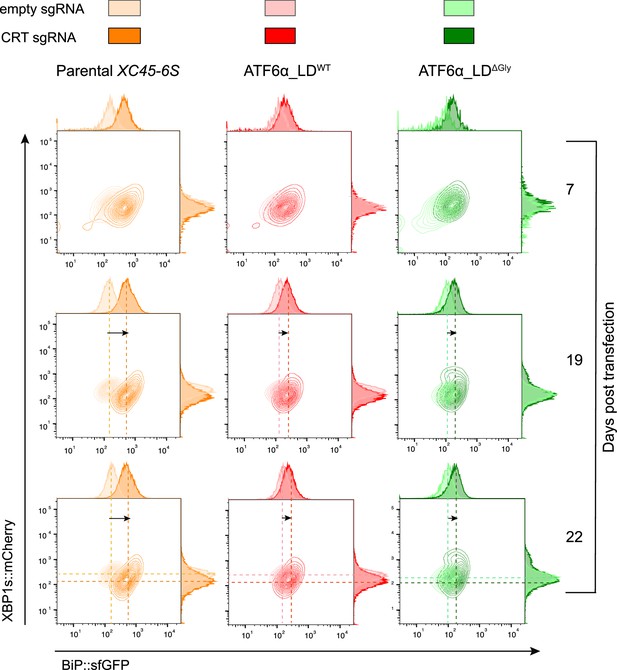
Calreticulin (CRT) depletion in ATF6α_LD∆Gly knock-in cells does not derepress basally ATF6α reporter activity.
Contour plots of BiP::sfGFP and XBP1s::mCherry signals from parental cells (orange) and ATF6α_LDWT (in red) or ATF6α_LD∆Gly (in green) rescued cells (generated in Figure 5B) where endogenous CRT was depleted by transient transfection with a sgRNA/Cas9 (Puromycin selection) targeting cgCRT locus. Transfected cells were selected in the presence of puromycin (8 μg/ml) and analysed under basal conditions at 7-, 19-, or 22-day post-transfection. Cells were also transfected with an empty sgRNA/Cas9 plasmid (pale colour) as control for the transfection effect. The presented dataset is representative of a single experiment.
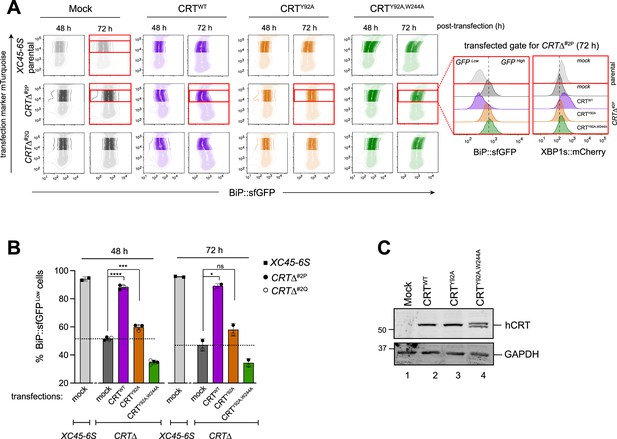
Impact of calreticulin (CRT) overexpression on cells depleted of CRT.
(A) Two-dimensional contour plots of BiP::sfGFP and mTurquoise signals (transfection marker) in parental cells and two derivative CRTΔ clones transiently transfected for 48 and 72 hr with plasmids encoding wild-type human CRT (CRTWT) or two lectin CRT mutants, CRTY92A and CRTY92A,W244A. As a control for transfection, cells were transfected with a mock plasmid. Shadowed rectangles mark cells that were not selected for analysis because they had low levels of mTurquoise-tagged plasmid (indicating low transfection) or have very high transfection levels that could be toxic. Cells within unshadowed rectangles and marked with red delineate those cells expressing moderate/high levels of mTurquoise-tagged plasmid selected for analysis of BiP::sfGFP and XBP1s::mCherry signals distribution. Right histograms indicate the transfected, gated cells 72 hr post-transfection (red rectangles). (B) Bar graph displaying the percentage of BiP::sfGFPlow cells (rescue phenotype) in mTurquoise-positive cells gated by the unshadowed boxes in ‘A’ as mean ± standard deviation (SD) of data obtained from one (72 hr) to two (48 hr) independent experiments. Data from both CRTΔ clones have been combined in each time point of analysis. Statistical analysis was performed by a two-sided unpaired Welch’s t-test and significance is indicated by asterisks (*p < 0.05; ***p < 0.001; ****p < 0.0001; ns: non-significant). (C) CHO-K1 cells were transiently transfected with the hCRT plasmids used in ‘A’ for 72 hr. Following transfection, cell lysates were recovered, and equal protein amounts were loaded into 12.5% sodium dodecyl sulfate–polyacrylamide gel electrophoresis (SDS–PAGE) gels and immunoblotted for hCRT to analyse protein stability in CRT mutants. GAPDH served as a loading control. The immunoblots are representative for two independent experiments.
-
Figure 6—source data 1
Raw images for gels shown in Figure 6.
- https://cdn.elifesciences.org/articles/96979/elife-96979-fig6-data1-v1.zip
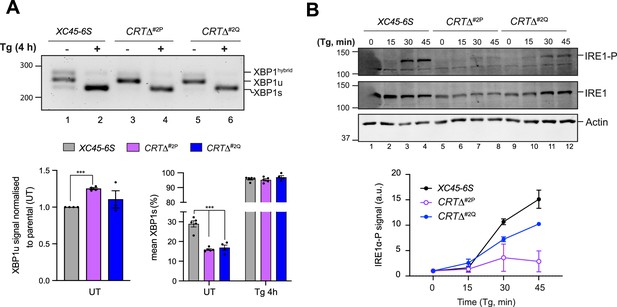
Calreticulin depletion exposes a negative feedback loop of ATF6α activity on IRE1.
(A) Top panel: Agarose gel of XBP1 cDNA from thapsigargin (Tg)-treated parental cells and two CRT∆ clones. Migration of the unspliced (XBP1u), spliced XBP1 (XBP1s), and hybrid (XBP1hybrid) stained DNA fragments is indicated. The XBP1hybrid band represents DNA species containing one strand of XBP1u and one strand of XBPs (Shang and Lehrman, 2004). Splicing was assessed by reverse transcription (RT)-PCR. Lower panel: Plots displaying the total XBP1u signal in the left panel, and the fraction of XBP1s in the right panel. For quantification, 50% of the hybrid band signal was assumed to be XBP1s and the other 50% XBP1u. The bars and error bars represent the mean ± standard error of the mean (SEM) of data obtained from more than three independent experiments. Statistical analysis was performed by a two-sided unpaired Welch’s t-test and significance is indicated by asterisks (***p < 0.001). (B) Representative immunoblots of endogenous levels of cgIRE1 and phosphorylated IRE1 (IRE1-P) in parental cells and two CRTΔ clones basally and upon endoplasmic reticulum (ER) stress induction with 0.5 μM Tg for the indicated times. The levels of IRE1-P signal over the time in each genotype are represented in a graph as mean ± standard deviation (SD) of data obtained from two independent experiments.
-
Figure 7—source data 1
Raw images for gels shown in Figure 7.
- https://cdn.elifesciences.org/articles/96979/elife-96979-fig7-data1-v1.zip
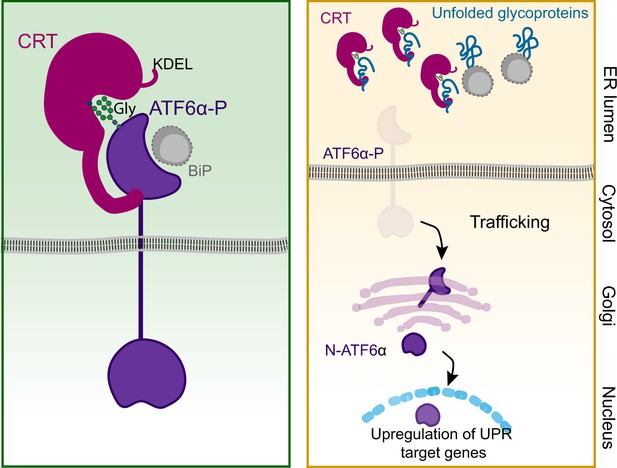
Proposed regulatory model of ATF6α by calreticulin (CRT) proteostasis.
Left panel: When concentrations of competing ligands (unfolded glycoproteins) are low, endoplasmic reticulum (ER) retention of the ATF6α–CRT complex is favoured (via CRT’s KDEL signal). The ATF6α–CRT interaction is cartooned here as having both glycan (Gly)-dependent and -independent components. Right panel: Unfolded glycoproteins compete with ATF6α for CRT and their increasing concentration favours ATF6α trafficking to the Golgi in stressed cells. A similar mechanism, involving BiP and shared by other unfolded protein response (UPR) transducers, has been previously proposed (Bertolotti et al., 2000) and is included here as a complement to ATF6α-specific regulation by CRT. ATF6α-P refers to the precursor form of ATF6α, while N-ATF6α denotes the active form of ATF6α.
Tables
Reagent type (species) or resource | Designation | Source or reference | Identifiers | Additional information |
---|---|---|---|---|
Recombinant DNA reagent | pRK793 His6_TEV(S219V)_Arg | Gift from Wang Lab | UK759 | His-TEV(S219V)-Arg from pRK793 |
Recombinant DNA reagent | pSpCas9(BB)-2A-Puro | Gift from Richard Timms | UK1367 | Puro-tagged CRISPR introduces double strand breaks (Addgene 48139) (Zhang PX459) |
Recombinant DNA reagent | Lenti-Cas9-Blast | Harding et al., 2019 | UK1674 | Lenti-Cas9-blasticidin resistance to make stable Cas9 expressing cells for CRISPR KO screening |
Recombinant DNA reagent | pMD2.G | Gift from Didier Trono | UK1700; RRID:Addgene_12259 | Addgene plasmid #12259, lentiviral packaging helper (VSVG) |
Recombinant DNA reagent | psPAX2 | Gift from Didier Trono | UK1701; RRID:Addbebe_12260 | Addgene plasmid #12260, lentiviral packaging helper |
Recombinant DNA reagent | pKLV-U6gRNA(BbsI)PGKpuro2ABFP | Koike-Yusa et al., 2014 | UK1789; RRID:Addgene_73542 | Addgene #50946, BFP-2A-Puro-tagged gRNA vector |
Recombinant DNA reagent | pKLV-CHO_libA-PGKpuro2ABFP | Ordóñez et al., 2021 | UK2561 | CRISPR-KO library of 125,030 selected guides for whole CHO genome screening |
Recombinant DNA reagent | cgROSA26_BiP::sfGFP_HDR | This study | UK2846 | DNA repair plasmid to integrate an ATF6 reporter (BiP::sfGFP) at ROSA26 locus in CHO genome |
Recombinant DNA reagent | cgROSA26_sgRNA_pSp Cas9(BB)-2A-Puro | This study | UK2847 | Puro-tagged sgRNA/Cas9 introduce double strand breaks in cgROSA26 |
Recombinant DNA reagent | pSpCas9 (BB)-2A-mTurquoise | This study | UK2915 | pSpCas9(BB)-2A vector to express mTurquoise together with guide RNA and Cas9 |
Recombinant DNA reagent | cgATF6α_sgRNA_Ex8_pSp Cas9(BB)-2A-mTurquoise | This study | UK2919 | mTurquoise-tagged sgRNA/Cas9 introduces double strand breaks in exon 8 of cgATF6α |
Recombinant DNA reagent | cgERp18_sgRNA_g1_pSp Cas9(BB)-2A-mTurquoise | This study | UK2920 | mTurquoise-tagged sgRNA/Cas9 introduces double strand breaks in exon 2 of TXNDC12 |
Recombinant DNA reagent | cgERp18_sgRNA_g2_pSp Cas9(BB)-2A-mTurquoise | This study | UK2921 | mTurquoise-tagged sgRNA/Cas9 introduces double strand breaks in exon 5 of TXNDC12 |
Recombinant DNA reagent | cgIRE1sgRNA_lentiGuide-Puro | This study | UK 2929 | Lentiviral vector expressing cgIRE1 CRISPR guide targeting exon 18 of IRE1 without expression of Cas9 |
Recombinant DNA reagent | cgATF6α_sgRNA_Ex2_pSpCas9 (BB)-2A-mCherry (MP1) | This study | UK2942 | mCherry-tagged sgRNA/Cas9 introduces double strand breaks in exon 2 of cgATF6α |
Recombinant DNA reagent | cgATF6_3xFLAG-mGL- TEV_HDR_pBS_V3 (MP1) | This study | UK2955 | HDR template for mGreenLantern knock-in at N-term of Hamster ATF6 |
Recombinant DNA reagent | SP_FLAGM1_9E10_BirA_WT_K DEL_pCDNA5_FRT_TO_MP3 | This study | UK2969 | WT BirA, targeted to ER (with a signal peptide and a KDEL) |
Recombinant DNA reagent | cgFURIN_sgRNA_Ex4_pSp Cas9(BB)-2A-mTurquoise | This study | UK2988 | mTurquoise-tagged sgRNA/Cas9 introduces double strand breaks in exon 4 of cgFURIN |
Recombinant DNA reagent | cgFURIN_sgRNA_Ex10_pSp Cas9(BB)-2A-mTurquoise | This study | UK2989 | mTurquoise-tagged sgRNA/Cas9 introduces double strand breaks in exon 10 of cgFURIN |
Recombinant DNA reagent | cgCRT_sgRNA_Ex1_pSp Cas9(BB)-2A-mTurquoise | This study | UK2990 | mTurquoise-tagged sgRNA/Cas9 introduces double strand breaks in exon 1 of cgCRT |
Recombinant DNA reagent | cgCRT_sgRNA_Ex3_pSp Cas9(BB)-2A-mTurquoise | This study | UK2991 | mTurquoise-tagged sgRNA/Cas9 introduces double strand breaks in exon 3 of cgCRT |
Recombinant DNA reagent | cgATF6_g1A_ex9_pSp Cas9(BB)2A-mTurquoise_MP2 | This study | UK3021 | mTurquoise-tagged sgRNA/Cas9 introduces double strand breaks in exon 9 of cgATF6α (PR3125 and 3126 based) |
Recombinant DNA reagent | cgATF6_g2A_ex10_pSp Cas9(BB)2A-mTurquoise_MP2 | This study | UK3024 | mTurquoise-tagged sgRNA/Cas9 introduces double strand breaks in exon 10 of cgATF6α (PR3133 and 3134 based) |
Recombinant DNA reagent | cgATF6_g2B_ex10_pSp Cas9(BB)2A-mTurquoise_MP2 | This study | UK3025 | mTurquoise-tagged sgRNA/Cas9 introduces double strand breaks in exon 10 of cgATF6α (PR3135 and 3136 based) |
Recombinant DNA reagent | cgATF6_TV_EX8-9_Strep-Tag- Twin_V.1.3_pUC57 | This study | UK3054 | Genescript plasmid encodes cgATF6 LDWT for rescue experiments |
Recombinant DNA reagent | cgATF6_410-659_noGly_V1_pUC57 | This study | UK3086 | pUC57 with ATF6 CDS gene fragment (all three glycans removed) for rescue experiments |
Recombinant DNA reagent | EGFP_cgATF6_TEV_410-659_ S12MUT_AviTag_pCEFL_pu (MP4) | This study | UK3122 | EGFP replacing the cytosolic domain of cgATF6 LD with a -AviTag and with S1P/S2P mutations in |
Recombinant DNA reagent | EGFP_cgATF6_TEV_410-659_ S12MUT_noGly_AviTag_pCEFL_pu (MP1) | This study | UK3141 | Glycan-free counterpart of UK3122 (TEV cleavage after TM) |
Recombinant DNA reagent | rCRT_18-416_pSUMO3 (MP3) | This study | UK3142 | Bacterial expression of luminal fragment of rat Calreticulin |
Recombinant DNA reagent | cgATF6_TV_EX8-9_Strep- tagtwin_CtoS_pUC57 mp7 | This study | UK3147 | Recombination template, UK3054 based, two cysteines changed to serine for rescue experiments |
Recombinant DNA reagent | EGFP_cgATF6_TEV_410-659_S1& 2MUT_CtoS_AviTag_pCEFL_pu_MP2 | This study | UK3157 | Cysteine free counterpart of UK3122 (TEV cleavage after TM) |
Recombinant DNA reagent | cgCNX_sgRNA_Ex2_pSp Cas9(BB)-2A-mTurquoise_MP1 | This study | UK3206 | mTurquoise-tagged sgRNA/Cas9 introduces double strand breaks in exon 2 of cgCNX (PR3364 and 3365 based) |
Recombinant DNA reagent | cgCNX_sgRNA_Ex4_pSp Cas9(BB)-2A-mTurquoise_MP2 | This study | UK3207 | mTurquoise-tagged sgRNA/Cas9 introduces double strand breaks in exon 4 of cgATF6α (PR3366 and 3367 based) |
Recombinant DNA reagent | hCRT_WT_pCEFL_BFP | This study | UK3231 | BFP-marked mammalian expression of untagged, WT human Calreticulin |
Recombinant DNA reagent | hCRT_Y92A_pCEFL_BFP | This study | UK3232 | BFP-marked mammalian expression of untagged, human Calreticulin lectin mutant |
Recombinant DNA reagent | hCRT_Y92A&W244A_pCEFL_BFP | This study | UK3233 | BFP-marked mammalian expression of untagged, human Calreticulin functional doble mutant |
Sequence-based reagent | cgATF6a_ex2_sgRNA_1S | This study | 3010 | CACCGGCTGGTGCGGCCGGGACCA |
Sequence-based reagent | cgATF6a_ex2_sgRNA_2AS | This study | 3011 | AAACTGGTCCCGGCCGCACCAGCC |
Sequence-based reagent | cgATF6a_sgRNA_ex8_1S | This study | 2921 | CACCGAAGAAGAAGGAGTATATGC |
Sequence-based reagent | cgATF6a_sgRNA_ex8_1S | This study | 2922 | AAACGCATATACTCCTTCTTCTTC |
Sequence-based reagent | cgATF6a_sgRNA_ex9_1S | This study | 3125 | CACCGTTTCCTAGATTGCTGTGCTA |
Sequence-based reagent | cgATF6a_sgRNA_ex9_2AS | This study | 3126 | AAACTAGCACAGCAATCTAGGAAAC |
Sequence-based reagent | cgATF6a_sgRNA_ex10_1S | This study | 3133 | CACCGCATTTATAATGCTGAACTA |
Sequence-based reagent | cgATF6a_sgRNA_ex10_2AS | This study | 3134 | AAACTAGTTCAGCATTATAAATGC |
Sequence-based reagent | cgATF6a_sgRNA_ex10_3S | This study | 3135 | CACCGAGGTGAGTATTCAAGTGTT |
Sequence-based reagent | cgATF6a_sgRNA_ex10_4AS | This study | 3136 | AAACAACACTTGAATACTCACCTC |
Sequence-based reagent | cgCRT_sgRNA_ex1_1S | This study | 3068 | CACCGTTCCGCGGCGGCCAGGCCG |
Sequence-based reagent | cgCRT_sgRNA_ex1_2AS | This study | 3069 | AAACCGGCCTGGCCGCCGCGGAAC |
Sequence-based reagent | cgCRT_sgRNA_ex3_1S | This study | 3070 | CACCGCGCGTAAAATCGGGCATCC |
Sequence-based reagent | cgCRT_sgRNA_ex3_2AS | This study | 3071 | AAACGGATGCCCGATTTTACGCGC |
Sequence-based reagent | cgFURIN_sgRNA_ex4_1S | This study | 3064 | CACCGTGGTCTCCATCCTGGACGA |
Sequence-based reagent | cgFURIN_sgRNA_ex4_2AS | This study | 3065 | AAACTCGTCCAGGATGGAGACCAC |
Sequence-based reagent | cgFURIN_sgRNA_ex10_1S | This study | 3066 | CACCGCCACCTCAATGCTAATGAT |
Sequence-based reagent | cgFURIN_sgRNA_ex10_2AS | This study | 3067 | AAACATCATTAGCATTGAGGTGGC |
Sequence-based reagent | cgCNX_sgRNA_ex2_1S | This study | 3364 | CACCGCAAAGCTCCAGTTCCAACAG |
Sequence-based reagent | cgCNX_sgRNA_ex2_2AS | This study | 3365 | AAACCTGTTGGAACTGGAGCTTTGC |
Sequence-based reagent | cgCNX_sgRNA_ex4_3S | This study | 3366 | CACCGGCTTGGTATCAAACAGGAA |
Sequence-based reagent | cgCNX_sgRNA_ex4_4AS | This study | 3367 | AAACTTCCTGTTTGATACCAAGCC |
Sequence-based reagent | cgERp18_sgRNAg1_ex2_1S | This study | 2962 | CACCGTTTTGGAGATCATATTCAC |
Sequence-based reagent | cgERp18_sgRNAg1_ex2_2AS | This study | 2963 | AAACGTGAATATGATCTCCAAAAC |
Sequence-based reagent | cgERp18_sgRNAg2_ex5_1S | This study | 2964 | CACCGTGGAATATAACCCCCATCA |
Sequence-based reagent | cgERp18_sgRNAg2_ex5_2AS | This study | 2965 | AAACTGATGGGGGTTATATTCCAC |
Sequence-based reagent | cgXBP1.19S5 | This study | 1470 | GGCCTTGTAATTGAGAACCAGGAG |
Sequence-based reagent | cgXBP1.14AS | This study | 5 | GAATGCCCAAAAGGATATCAGACTC |
Antibody | Polyclonal Rabbit anti-calreticulin | Abcam | Cat# ab19261, RRID:AB_955722 | Used at 1:1000 |
Antibody | Polyclonal Chicken anti-calreticulin | Invitrogen | Cat# PA1-902A, RRID:AB_2069607 | Used at 1:1000 |
Antibody | Polyclonal Rabbit anti-calnexin | Enzo Life Sciences | Cat# ADI-SPA-860-D, RRID:AB_2038898 | Used at 1:1000 |
antibody | Polyclonal Rabbit anti-FURIN | Abcam | Cat# ab3467, RRID:AB_303828 | Used at 1:1000 |
Antibody | Monoclonal Mouse anti IRE1a_LD serum | Bertolotti et al., 2000 | NY200 | Used at 1:1000 |
Antibody | Monoclonal Rabbit anti p-IRE1 | Genetech | Used at 1:1000 | |
Antibody | Polyclonal Chicken anti-hamster BiP | Avezov et al., 2013 | anti-BiP | Used at 1:1000 |
Antibody | Monoclonal Mouse anti FLAG-M2 | Sigma | Cat# F1804, RRID:AB_262044 | Used at 1:1000 |
Antibody | Polyclonal Rabbit anti GFP | Marciniak et al., 2004 | NY | Used at 1:1000 |
Antibody | Monoclonal Mouse anti KDEL (10C3) | Enzo Life Sciences | ENZ-ABS679 | Used at 1:1000 |
Antibody | Monoclonal Mouse anti-actin | Fisher Scientific | clone C4-691002 | Used at 1:1000 |
Antibody | Monoclonal Rabbit anti-GAPDH | Cell Signalling | 14C10; mab# 2118 | Used at 1:2000 |
Antibody | Polyclonal rabbit polyclones anti-α/β-Tubulin | Cell Signalling | CST2148S | Used at 1:1000 |
Chemical compound, drug | DMEM | Sigma | D6429 | |
Chemical compound, drug | HyClone II Serum | Thermo Fisher Scientific | SH30066.03 | |
Chemical compound, drug | Penicillin/Streptomycin | Sigma | P0781 | |
Chemical compound, drug | L-Glutamine | Sigma | G7513 | |
Chemical compound, drug | Non-essential amino acids solution | Sigma | M7145 | |
Chemical compound, drug | Nutrient Mixture F12 | Sigma | N4888 | |
Chemical compound, drug | Lipofectamine LTX | Thermo Fisher Scientific | A12621 | |
Chemical compound, drug | Puromycin | MERCK-milipore | 540222 | |
Chemical compound, drug | EDTA-free Protease inhibitor Cocktail | Roche | 11873580001 | |
Chemical compound, drug | Anti-FLAG M2 Affinity Gel | Sigma | F3165 | |
Chemical compound, drug | ChromoTek GFP-Trap Agarose | Thermo Fisher Scientific | Tag-20 | |
Chemical compound, drug | Tunicamycin | Melford | T2250 | |
Chemical compound, drug | 2-Deoxyglucose (2DG) | ACROS Organics | D6134 | |
Chemical compound, drug | Ceapin A7 | Sigma | SML2330 | |
Chemical compound, drug | Thapsigargin | Calbiochem | CAS 67526-95-8 | |
Chemical compound, drug | PF-429242 dihydrochloride (S1P inhibitor) | Tocris | SML0667 | |
Chemical compound, drug | 4μ8C | Tocris Bioscience | 4479 | |
Chemical compound, drug | TRIzolä Reagent | Ambion/Invitrogen | 15596026 | |
Chemical compound, drug | PureLinkä RNA mini kit | Invitrogen | 12183018A | |
Chemical compound, drug | RevertAid Reverse Transcriptase | Thermo Scientific | EP0441 | |
Chemical compound, drug | FURIN | New England Biolabs | P8077S | |
Chemical compound, drug | MBP5-FN-paramyosin-ΔSal | New England Biolabs | E8052S | |
Chemical compound, drug | MycoAlert (TM) Mycoplasma Detection Kit | Lonza | LT07-118 | |
Cell line (Cricetulus griseus) | CHO XC45-6S | This study | XC45-6S | Parental ATF6α/IRE dual UPR reporter CHO cell line expressing XBP1s::mCherry and BiP:sfGFP reporters |
Cell line (Cricetulus griseus) | XC45-6S cells-Cas9 stable#21 | This study | XC45-6S-Cas9 stable#21 | Parental ATF6α/IRE dual UPR reporter CHO cell line expressing XBP1s::mCherry and BiP:sfGFP reporters stably expressing Cas9 |
Cell line (Cricetulus griseus) | CHO-K1 XC45-6S, XBP1s::mCherry & BiP:sfGFP ATF6α∆ (KO) | This study | XC45-6S, ATF6∆, #B6 | ATF6α/IRE dual reporter cell line with depletion of ATF6_clone B6 |
Cell line (Cricetulus griseus) | CHO-K1 XC45-6S, XBP1s::mCherry & BiP:sfGFP ATF6α∆ (KO) | This study | XC45-6S, ATF6∆, #5A2 | ATF6α/IRE dual reporter cell line with depletion of ATF6_clone 5A2 |
Cell line (Cricetulus griseus) | CHO-K1 XC45-6S, ATF6αLDWT rescue | This study | XC45-6S, ATF6αLDWT #R2_9 | ATF6α/IRE dual reporter cell line with depletion of ATF6_LD and rescue with ATF6αLDWT _clone R2.9 |
Cell line (Cricetulus griseus) | CHO-K1 XC45-6S, ATF6αLD∆Gly rescue | This study | XC45-6S, ATF6αLD∆Gly#GF6 | ATF6α/IRE dual reporter cell line with depletion of ATF6_LD and rescue with ATF6αLD∆Gly_clone 6 |
Cell line (Cricetulus griseus) | CHO-K1 XC45-6S, ATF6αLD∆C rescue | This study | XC45-6S, ATF6αLD∆C#CS10 | ATF6α/IRE dual reporter cell line with depletion of ATF6_LD and rescue with ATF6αLD∆C_clone 10 |
Cell line (Cricetulus griseus) | CHO-K1 3xFLAG-mGL_ATF6α knock-in cells | This study | 2K cells | CHO-K1 cells with endogenous ATF6 tagged with a 3xFLAG-mGL tag |
Cell line (Cricetulus griseus) | CHO-K1 GFP-cgATF6αLD_S1P&S2Pmut | This study | UK3122 cells | CHO-K1 stable expressing a GFP-cgATF6αLD_S1P&S2Pmut |
Cell line (Cricetulus griseus) | CHO-K1 XC45-6S, CRT∆#2P | This study | XC45-6S, CRTα∆#2P | ATF6α/IRE dual reporter cell line with depletion of CRT_clone 2P |
Cell line (Cricetulus griseus) | CHO-K1 XC45-6S, CRT∆#2Q | This study | XC45-6S, CRTα∆#2Q | ATF6α/IRE dual reporter cell line with depletion of ATF6-clone 2Q |
Cell line (Cricetulus griseus) | CHO-K1 XC45-6S, FURIN∆#2.2.G | This study | XC45-6S, FURIN∆#2.2.G | ATF6α/IRE dual reporter cell line with depletion of Furin_ clone 2.2.G |
Cell line (Cricetulus griseus) | CHO-K1 XC45-6S, CNX∆#6 | This study | XC45-6S, CNX∆#6 | ATF6α/IRE dual reporter cell line with depletion of CNX_clone 6 |
Cell line (Cricetulus griseus) | CHO-K1 XC45-6S, ERp18∆#7E2 | This study | XC45-6S, ERp18∆#7E2 | ATF6α/IRE dual reporter cell line with depletion of ERp18_clone 7E2 |
Software, algorithm | MAGeCK | Li et al., 2014 | ||
Software, algorithm | Metascape | Zhou et al., 2019 | ||
Software, algorithm | MacVector | |||
Software, algorithm | Photoshop and Illustrator | |||
Software, algorithm | FlowJo | |||
Software, algorithm | ImageJ | |||
Software, algorithm | GraphPad-Prism V8 | |||
Software, algorithm | Volocity V6.3 | PerkinElmer |
Additional files
-
Supplementary file 1
Ranking of genes enriched in the activator and repressor ATF6α screens.
Genes are ranked by ‘pos | rank’ value. Notably, MBTPS1, encoding for S1P protease, is on the top of positively selected genes in the activator screen, while SETDB1 (SET Domain Bifurcated Histone Lysine Methyltransferase 1) is on the top position among the positively selected genes in the repressor screen. The top 100 positively selected genes correspond to Figure 1C, D.
- https://cdn.elifesciences.org/articles/96979/elife-96979-supp1-v1.xlsx
-
Transparent reporting form
- https://cdn.elifesciences.org/articles/96979/elife-96979-transrepform1-v1.docx