Disordered proteins interact with the chemical environment to tune their protective function during drying
Figures
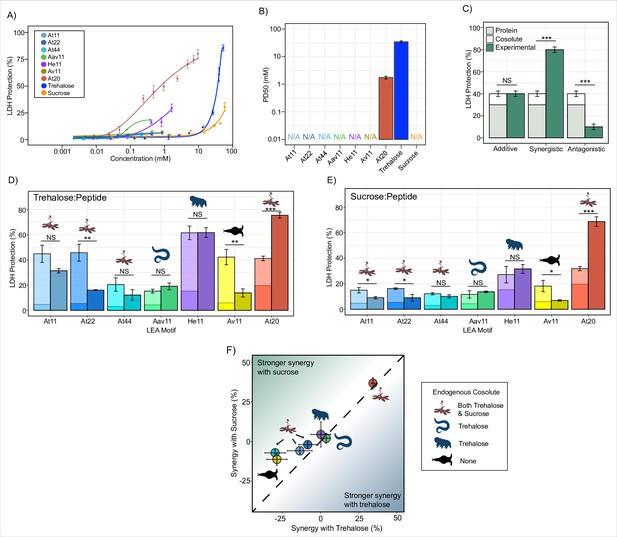
Late embryogenesis abundant (LEA) motifs are not synergistic with endogenous cosolutes.
(A) Sigmoidal plot representing percent of lactate dehydrogenase (LDH) stabilization by LEA motifs and cosolutes as a function of the molar concentration. error bars = standard deviation. (B) Protective dose 50 (PD50) for additives obtained by sigmoidal fitting of data in (A). N/A represents instances where 50% protection was not achieved. (C) Example plot showing possible outcomes (additive, synergistic, or antagonistic effect) from cosolute:IDP mixtures. ‘Cosolute’ and ‘Protein’ represent the percent LDH protection by cosolute and protein respectively. Experimental represents the experimental protection resulting from cosolute and protein mixtures. (D) Synergy plots for trehalose:LEA peptide at 100:1 molar ratio. Welch’s t-test was used for statistical comparison, error bars = standard deviation. (E) Synergy plots for sucrose:LEA peptide at 100:1 molar ratio. (F) Plot representing synergy with trehalose vs. sucrose from (D, E). Dotted line represents instances where there is equal synergy with both trehalose and sucrose. All LDH experiments were done with three technical replicates.
-
Figure 1—source data 1
Lactate dehydrogenase (LDH) assay data for motifs and cosolutes used in the study related to Figure 1A and B.
- https://cdn.elifesciences.org/articles/97231/elife-97231-fig1-data1-v1.xlsx
-
Figure 1—source data 2
Synergy data for cosolute:motif mixtures related to Figure 1C–F.
- https://cdn.elifesciences.org/articles/97231/elife-97231-fig1-data2-v1.xlsx
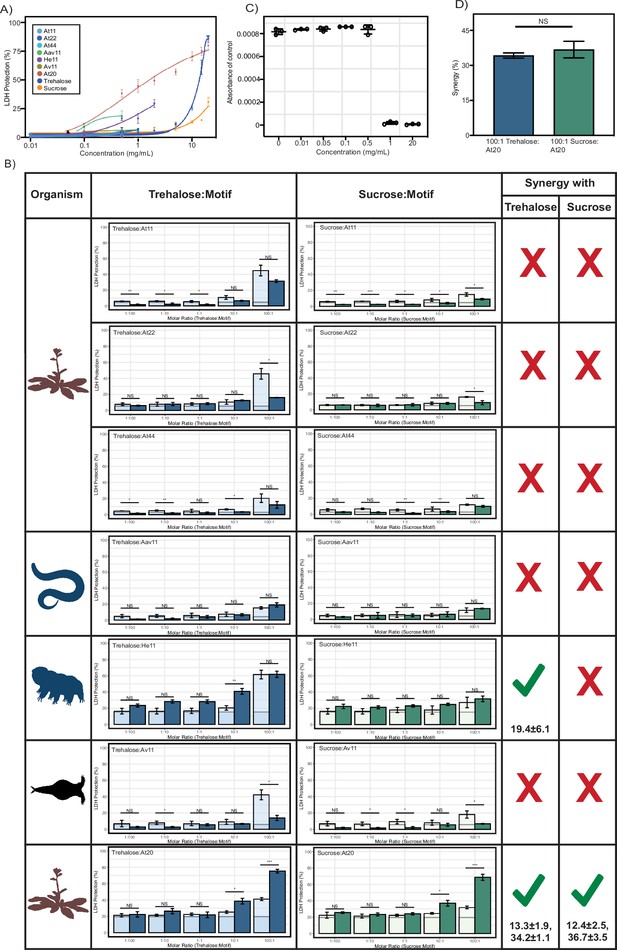
Lactate dehydrogenase (LDH) assay for late embryogenesis abundant (LEA) motifs and cosolutes.
(A) Sigmoidal plot representing concentration dependence of LDH protection by LEA motifs and cosolutes as a function of the weight/volume concentration. (B) Synergy plots for cosolutes with LEA motifs at 1:100, 1:10, 1:1, 10:1, and 100:1 molar ratios. n = 3, Welch’s t-test was used for statistical comparison, error bars = standard deviation. Numbers under the checkmarks represent the mean percentage synergy and standard deviation. (C) Change in NADH absorbance values for the refrigerated controls of Aav11 at different concentrations after 16 hr. (D) Percent synergy comparison for At20 with trehalose and sucrose at 100:1 ratio. Error bars represent standard deviation. Welch’s t-test was used for statistical comparison. All LDH assays were performed with three technical replicates. Organismal icons that are colored blue indicate the species uses trehalose as an endogenous cosolute, brown indicates the species uses both trehalose and sucrose, and black indicates the species uses neither trehalose nor sucrose as an endogenous cosolute.
-
Figure 1—figure supplement 1—source data 1
All data related to Figure 1—figure supplement 1.
- https://cdn.elifesciences.org/articles/97231/elife-97231-fig1-figsupp1-data1-v1.xlsx
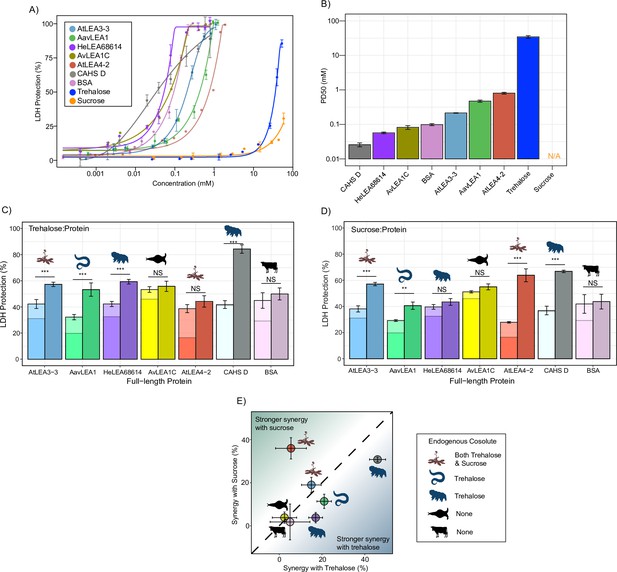
Full-length desiccation-related intrinsically disordered proteins (IDPs) act synergistically with cosolutes.
(A) Concentration dependence of lactate dehydrogenase (LDH) protection by full-length proteins and cosolutes used in this study. (B) PD50 for additives obtained by sigmoidal fitting of concentration-dependent LDH protection from (A). N/A represents instances where 50% protection was not achieved. (C) Synergy plots for trehalose:protein at 100:1 molar ratio. Welch’s t-test was used for statistical comparison, error bars = standard deviation. (D) Synergy plots for sucrose:protein at 100:1 molar ratio. Welch’s t-test was used for statistical comparison, error bars = standard deviation. (E) Plot representing synergy with trehalose vs. sucrose from (C, D). All LDH experiments were done with three technical replicates.
-
Figure 2—source data 1
Lactate dehydrogenase (LDH) assay data for full-length proteins and cosolutes used in the study related to Figure 2A and B.
- https://cdn.elifesciences.org/articles/97231/elife-97231-fig2-data1-v1.xlsx
-
Figure 2—source data 2
Synergy data for cosolute:protein mixtures related to Figure 1C–E.
- https://cdn.elifesciences.org/articles/97231/elife-97231-fig2-data2-v1.xlsx
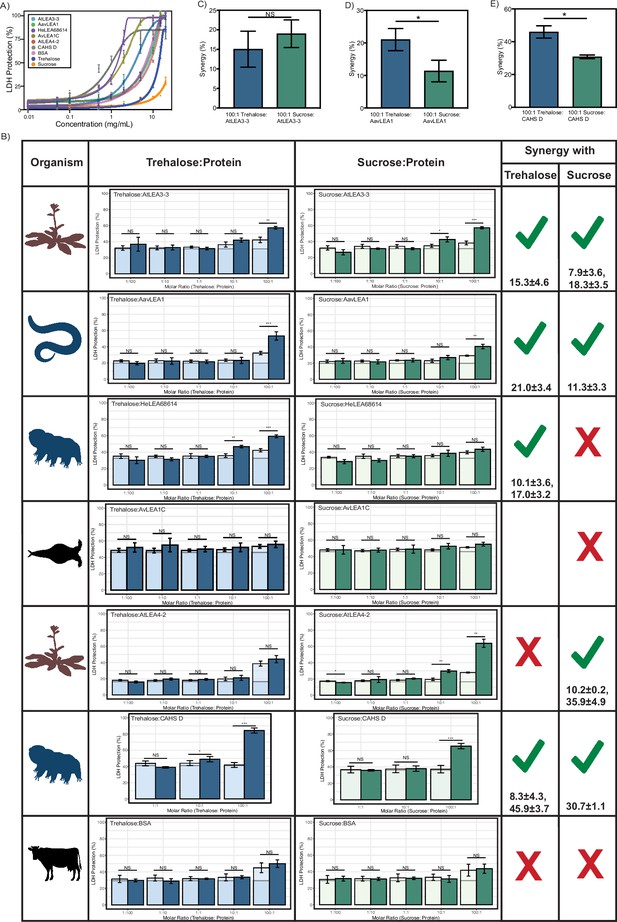
Lactate dehydrogenase (LDH) assay for full-length proteins and cosolutes.
(A) Sigmoidal plot representing concentration dependence of LDH protection by full-length proteins and cosolutes as a function of the weight/volume concentration. (B) Synergy plots for cosolute with full-length proteins at respective molar ratios. n = 3, Welch’s t-test was used for statistical comparison, error bars = standard deviation. Numbers under the checkmarks represent the mean percentage synergy and standard deviation. (C–E) Percent synergy comparison for (C) AtLEA3-3, (D) AavLEA1, and (E) CAHS D with trehalose and sucrose at 100:1 ratio. n = 3, error bars = standard deviation. Welch’s t-test was used for statistical comparison. All LDH assays were performed with three technical replicates. Organismal icons that are colored blue indicate the species uses trehalose as an endogenous cosolute, brown indicates the species uses both trehalose and sucrose, and black indicates the species uses neither trehalose nor sucrose as an endogenous cosolute.
-
Figure 2—figure supplement 1—source data 1
All data related to Figure 2—figure supplement 1.
- https://cdn.elifesciences.org/articles/97231/elife-97231-fig2-figsupp1-data1-v1.xlsx
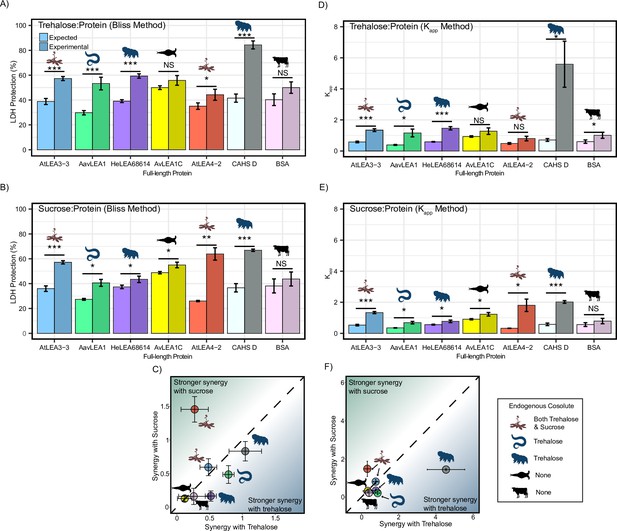
Synergy calculation using alternative methods.
Synergy plots for (A) trehalose:protein and (B) sucrose:protein calculated using the Bliss method. (C) Plot represents synergy with trehalose vs. sucrose using the Bliss method. (D, E) Synergy plots for trehalose:protein and sucrose:protein respectively calculated using the Kapp method. (F) Plot represents synergy with trehalose vs. sucrose using the Kapp method. All lactate dehydrogenase (LDH) experiments were done once with three technical replicates. Error bars represent standard deviation.
-
Figure 2—figure supplement 2—source data 1
All data related to Figure 2—figure supplement 2.
- https://cdn.elifesciences.org/articles/97231/elife-97231-fig2-figsupp2-data1-v1.xlsx
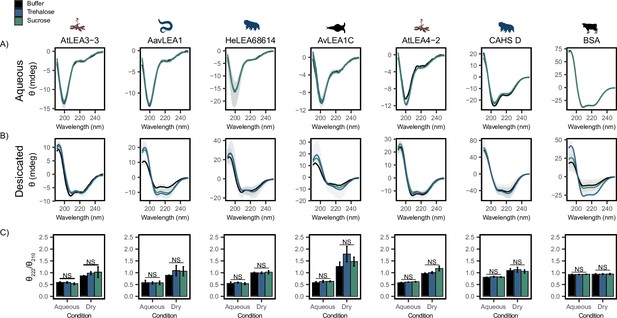
Functional synergy is not mediated by secondary structural changes.
Buffer represents the circular dichroism (CD) analysis for the proteins without cosolutes. Trehalose and sucrose represent the CD analysis for trehalose:protein mixture and sucrose:protein mixture at 100:1 molar ratio respectively. (A, B) CD spectra for protein and cosolute:protein mixtures at 100:1 molar ratio under aqueous (A) and (B) desiccated conditions. Each plot represents the average of three technical replicates, with the shaded region representing the standard deviation of the average. (C) Changes in the ratio of CD signal at 222 and 210 nm for individual protein and cosolute:protein mixtures under aqueous and desiccated conditions. error bars = standard deviation, statistical analysis was done by pairwise t-test between each protein and its cosolute mixtures. Organismal icons that are colored blue indicate the species uses trehalose as an endogenous cosolute, brown indicates the species uses both trehalose and sucrose, and black indicates the species uses neither trehalose nor sucrose as an endogenous cosolute.
-
Figure 3—source data 1
Circular dichroism (CD) data related to Figure 3.
- https://cdn.elifesciences.org/articles/97231/elife-97231-fig3-data1-v1.xlsx
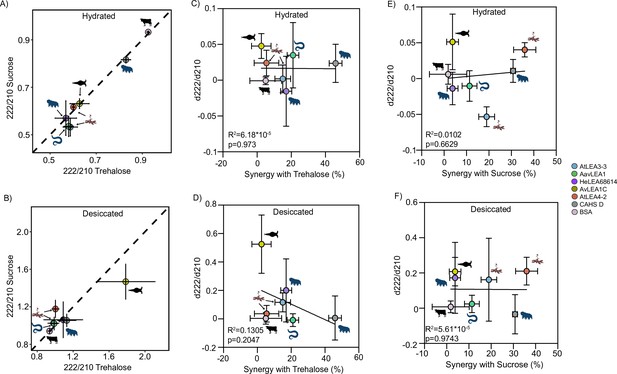
Synergy and secondary structure.
(A) Plot comparing secondary structural changes in proteins with trehalose vs. sucrose at 100:1 molar ratio under hydrated conditions. Dotted line represents cases where there is equal structural shift in both trehalose and sucrose mixture. (B) Plot comparing secondary structural alterations in proteins with trehalose vs. sucrose at 100:1 molar ratio under desiccated conditions. Dotted line represents cases where there is equal structural shift in both trehalose and sucrose mixture. (C) Correlation plots for synergy vs. secondary structural shift in the presence of trehalose in the hydrated state. (D) Correlation plots for synergy vs. secondary structural shift in the presence of trehalose in the desiccated state. (E) Correlation plots for synergy vs. secondary structural shift in the presence of sucrose in the hydrated state. (F) Correlation plots for synergy vs. secondary structural shift in the presence of sucrose in the desiccated state. Organismal icons that are colored blue indicate the species uses trehalose as an endogenous cosolute, brown indicates the species uses both trehalose and sucrose, and black indicates the species uses neither trehalose nor sucrose as an endogenous cosolute.
-
Figure 3—figure supplement 1—source data 1
All data related to Figure 3—figure supplement 1.
- https://cdn.elifesciences.org/articles/97231/elife-97231-fig3-figsupp1-data1-v1.xlsx
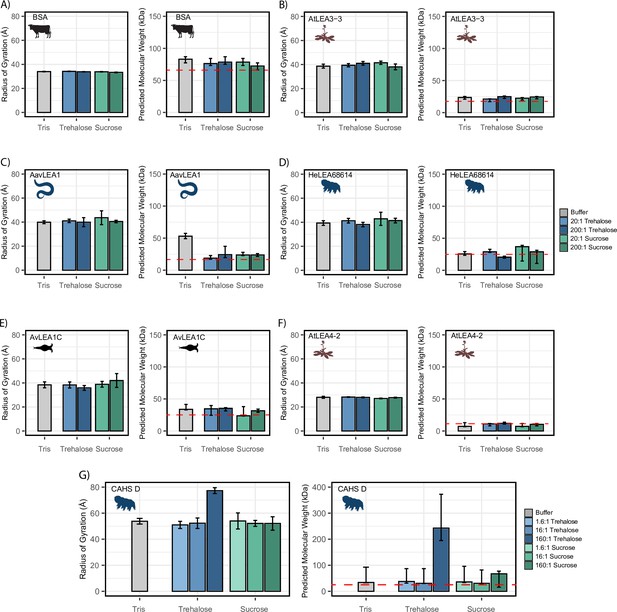
Cosolutes increase the global dimensions of CAHS D, but not late embryogenesis abundant (LEA) or bovine serum albumin (BSA).
Analyzed data from small-angle X-ray scattering (SAXS) experiments of proteins at 4 mg/mL in 20 mM Tris-HCl pH 7. Proteins tested include (A) BSA (0.0578 mM), (B) AtLEA3-3 (0.22 mM), (C) AavLEA1 (0.249 mM), (D) HeLEA68614 (0.156 mM), (E) AvLEA1C (0.163 mM), (F) AtLEA4-2 (0.38 mM), and (G) CAHS D (0.156 mM). The left plot shows the radius of gyration of the protein in the presence of no cosolute (gray), increasing molar ratios of trehalose (blue shades), and increasing molar ratios of sucrose (green shades). All SAXS experiments were performed once with three technical replicates. Error bars represent uncertainty in the measurement, provided by BioXTAS RAW. The right plot shows molecular weight values derived from Guinier analysis (see ‘Materials and methods’). The red dashed line indicates the monomeric protein’s molecular weight. Color scheme is the same as in the left figure. Error bars represent >90% confidence interval, which is directly obtained from the analysis. Organismal icons that are colored blue indicate the species uses trehalose as an endogenous cosolute, brown indicates the species uses both trehalose and sucrose, and black indicates the species uses neither trehalose nor sucrose as an endogenous cosolute.
-
Figure 4—source data 1
Small-angle X-ray scattering (SAXS) data related to Figure 4.
- https://cdn.elifesciences.org/articles/97231/elife-97231-fig4-data1-v1.xlsx
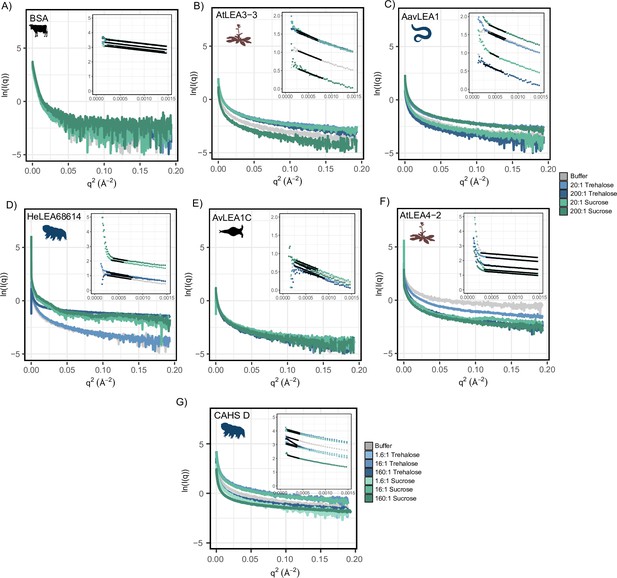
Raw small-angle X-ray scattering (SAXS) data from the experiments shown in Figure 4 for 4mg/mL.
(A) Bovine serum albumin (BSA) (0.0578 mM), (B) AtLEA3-3 (0.22 mM), (C) AavLEA1 (0.249 mM), (D) HeLEA68614 (0.156 mM), (E) AvLEA1C (0.163 mM), (F) AtLEA4-2 (0.38 mM), and (G) CAHS D (0.156 mM). Each plot displays raw SAXS data on a Guinier scale, with a zoomed-in portion in the top right displaying the Guinier region. The Guinier fit is displayed as a black trendline. In each plot, the protein’s scattering profile in several solution environments is shown, including protein in the presence of no cosolute (black), a 20:1 molar ratio of trehalose (light blue), a 200:1 molar ratio of trehalose (dark blue), a 20:1 molar ratio of sucrose (light green), and a 200:1 molar ratio of sucrose (dark green). CAHS D data contains slightly different molar ratios of 1.6:1 trehalose (very light blue), 16:1 trehalose (light blue), 160:1 trehalose (dark blue), 1.6:1 sucrose (very light green), 16:1 sucrose (light green), and 160:1 sucrose (dark green). Unlike other samples, a Guinier analysis with a qMaxRg of 1.1 could not be obtained. A best attempt to establish the Rg of this sample (qMaxRg ~1.35) revealed a significant increase in the Rg. Given the clear curvature of the Guinier region, this data was consistent with the presence of large oligomeric species. Organismal icons that are colored blue indicate the species uses trehalose as an endogenous cosolute, brown indicates the species uses both trehalose and sucrose, and black indicates the species uses neither trehalose nor sucrose as an endogenous cosolute.
-
Figure 4—figure supplement 1—source data 1
All data related to Figure 4—figure supplement 1.
- https://cdn.elifesciences.org/articles/97231/elife-97231-fig4-figsupp1-data1-v1.xlsx
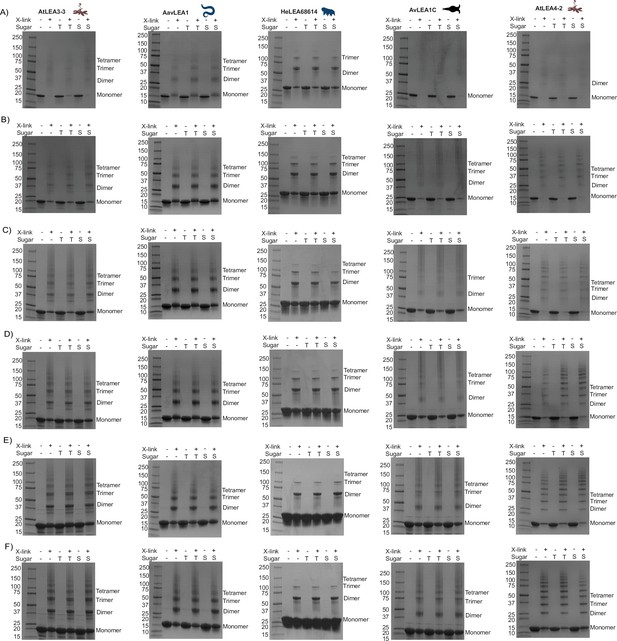
Photo-induced crosslinking of unmodified proteins (PICUP) gels for full-length late embryogenesis abundant (LEA) proteins and in mixtures with trehalose or sucrose.
A range of protein concentrations were used to cover the concentrations used in the synergy assay. Protein concentrations used were (A) 25 μM, (B) 50 μM, (C) 75 μM, (D) 100 μM, (E) 150 μM, and (F) 200 μM. Each gel contains protein ladder (Precision Plus Protein Dual Xtra Standards, Bio-Rad, Cat# 161-0377) in the first lane, protein at desired concentration in the second lane, crosslinked protein in the third lane, 100:1 trehalose:protein in the fourth lane, crosslinked 100:1 trehalose:protein in the fifth lane, 100:1 sucrose:protein in the sixth lane, and crosslinked 100:1 sucrose:protein in the last lane. Organismal icons that are colored blue indicate the species uses trehalose as an endogenous cosolute, brown indicates the species uses both trehalose and sucrose, and black indicates the species uses neither trehalose nor sucrose as an endogenous cosolute.
-
Figure 4—figure supplement 2—source data 1
Original raw files for all PICUP gels in Figure 4—figure supplement 2.
- https://cdn.elifesciences.org/articles/97231/elife-97231-fig4-figsupp2-data1-v1.zip
-
Figure 4—figure supplement 2—source data 2
Files for PICUP gels in Figure 4—figure supplement 2 and original gels with highlighted portions and labels.
- https://cdn.elifesciences.org/articles/97231/elife-97231-fig4-figsupp2-data2-v1.docx
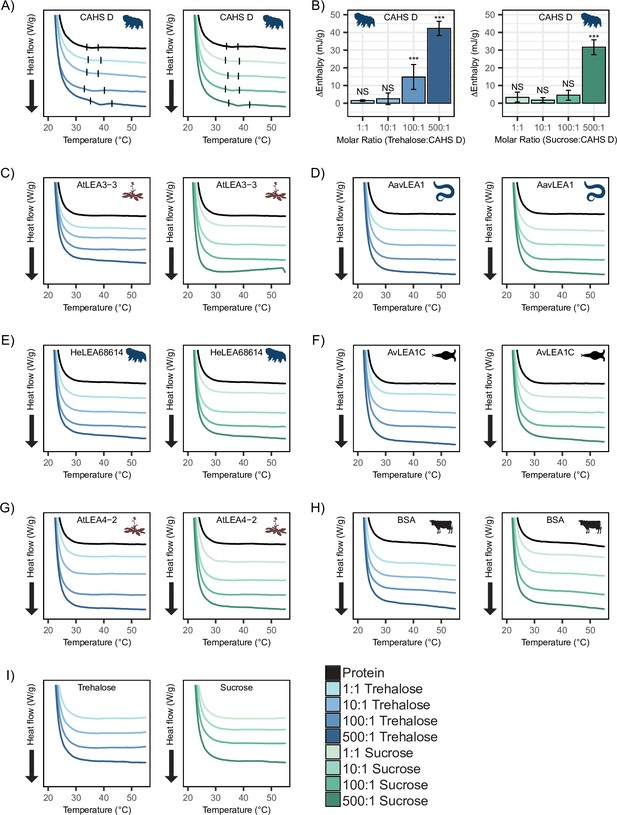
Differential scanning calorimetry (DSC) thermograms show cosolutes promote gelation of CAHS D but not late embryogenesis abundant (LEA) proteins.
All DSC thermograms represent the average of three technical replicates from which the enthalpy were derived. (A) DSC thermogram of 0.235 mM CAHS D with trehalose (left, blue lines) and sucrose (right, green lines) at increasing cosolute:protein molar ratios. (B) Change in enthalpy measurements for cosolute:CAHS D mixtures relative to CAHS D. Enthalpy measurements were done by taking the area of gel melt peaks represented by black dashes in (A) (see ‘Materials and methods’). t-test was used to determine pairwise comparisons between CAHS D and its cosolute mixtures. Error bars represent standard deviation. (C–H) DSC thermogram of LEA proteins and bovine serum albumin (BSA) at 0.235 mM and with the addition of trehalose and sucrose at molar ratios of 1:1, 10:1, 100:1, and 500:1 (cosolute:protein). (I) DSC thermogram for trehalose and sucrose in the absence of proteins at respective concentrations for different molar ratios. Organismal icons that are colored blue indicate the species uses trehalose as an endogenous cosolute, brown indicates the species uses both trehalose and sucrose, and black indicates the species uses neither trehalose nor sucrose as an endogenous cosolute.
-
Figure 5—source data 1
Heat flow and enthalpy measurements for CAHS D related to Figure 5A and B.
- https://cdn.elifesciences.org/articles/97231/elife-97231-fig5-data1-v1.xlsx
-
Figure 5—source data 2
Overlay of differential scanning calorimetry (DSC) thermograms for CAHS D from which the resulting enthalpy was calculated.
- https://cdn.elifesciences.org/articles/97231/elife-97231-fig5-data2-v1.docx
-
Figure 5—source data 3
Heat flow and enthalpy measurements for bovine serum albumin (BSA) and late embryogenesis abundant (LEA) proteins related to Figure 5C–I.
- https://cdn.elifesciences.org/articles/97231/elife-97231-fig5-data3-v1.xlsx
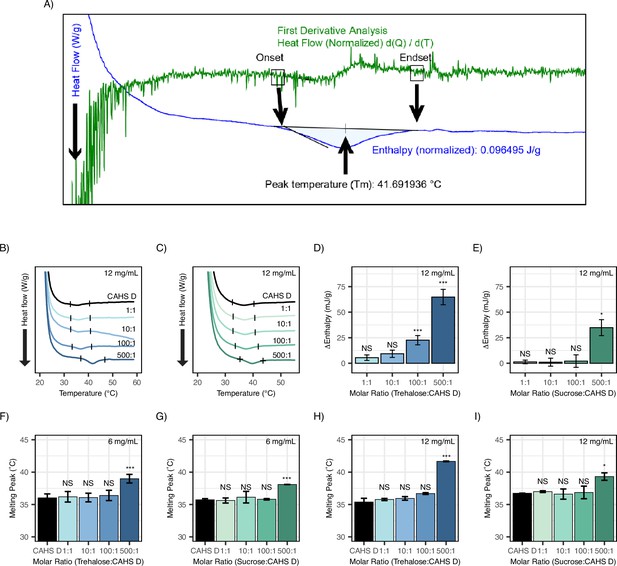
Differential scanning calorimetry (DSC) thermograms and calculation of enthalpy and melting points.
(A) Sample enthalpy analysis using TRIOS software. First derivative analysis was used to aid in selecting the onset and endset in the thermogram representative of protein gelation. This is done by identifying observable spikes that correspond to where the curve begins to dip (onset) or level out (endset). The area between the approximate onset and endset is selected and TRIOS software is used to calculate defined onset and endset values, enthalpy (J/g), and peak temperature (Tm, °C) by analyzing the area above this feature within the identified range. (B) DSC thermograms of CAHS D at 12 mg/mL (0.47 mM) in varying molar ratios of trehalose (0:1, 1:1, 10:1, 100:1, and 500:1). (C) DSC thermograms of CAHS D at 12 mg/mL (0.47 mM) in varying molar ratios of sucrose (0:1, 1:1, 10:1, 100:1, and 500:1). DSC thermogram represents the average for three technical replicates. (D) Change in enthalpy measurements for trehalose:CAHS D mixtures relative to CAHS D at 12 mg/mL. Enthalpy measurements were done by taking the area of gel melt peaks represented by black dashes in (B). (E) Change in enthalpy measurements for sucrose:CAHS D mixtures relative to CAHS D at 12 mg/mL. Enthalpy measurements were done by taking the area of gel melt peaks represented by black dashes in (C). (F–I) Melting temperature for the gel melts as calculated from the DSC thermograms for CAHS D and cosolute:CAHS D mixtures at respective ratios. t-test was used for pairwise comparisons of CAHS D with its cosolute mixtures.
-
Figure 5—figure supplement 1—source data 1
All data related to Figure 5—figure supplement 1.
- https://cdn.elifesciences.org/articles/97231/elife-97231-fig5-figsupp1-data1-v1.xlsx
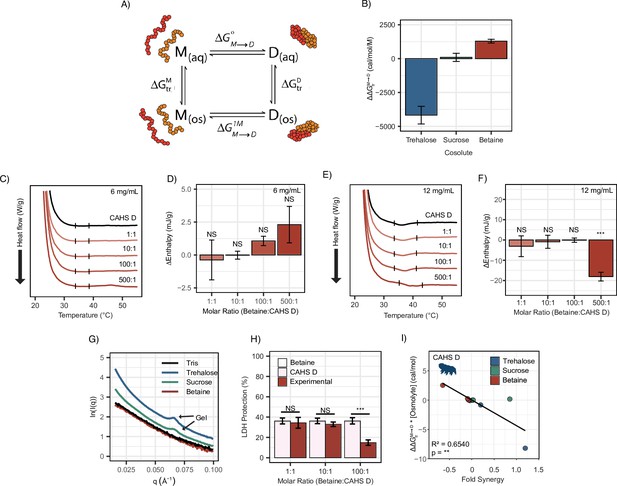
Transfer free energy of CAHS D highlights the difference between synergistic and non-synergistic cosolutes.
(A) Tanford’s transfer model, depicting the effect of cosolutes on the dimerization of two proteins. ‘M’' represents the protein’s monomeric state, ‘D’ represents the dimeric state, ‘aq’' represents an aqueous solution, and ‘os’' represents a solution containing some osmolyte. (B) The difference in between two CAHS D monomers and a CAHS D dimer. All structures are predicted by AlphaFold2 (Jumper et al., 2021; Jumper et al., 2020). (C) Differential scanning calorimetry (DSC) thermograms of CAHS D at 6 mg/mL (0.235 mM) in varying molar ratios of glycine betaine. (D) Change in enthalpy measurements for betaine:CAHS D mixtures relative to CAHS D at 6 mg/mL. Enthalpy measurements were done by taking the area of gel melt peaks represented by black dashes in (C). (E) Same as (C) but with CAHS D at 12 mg/mL (0.47 mM). (F) Change in enthalpy measurements for betaine:CAHS D mixtures relative to CAHS D at 12 mg/mL. (G) Small-angle X-ray scattering (SAXS) data depicting scattering profiles of 4 mg/mL CAHS D in Tris (black), 1000:1 trehalose (blue), 1000:1 sucrose (green), and 1000:1 glycine betaine (red). (H) Lactate dehydrogenase (LDH) synergy assay for glycine betaine and CAHS D at various molar ratios. Welch’s t-test was used for statistical comparison. n = 3, error bars represent standard deviation. (I) A correlation of of a given cosolute with CAHS D and its synergy in the LDH assay at different molar ratios. p-value is given from a Pearson correlation.
-
Figure 6—source data 1
All data related to Figure 6B–I.
- https://cdn.elifesciences.org/articles/97231/elife-97231-fig6-data1-v1.xlsx
-
Figure 6—source data 2
Equations and overlay of differential scanning calorimetry (DSC) thermograms for CAHS D from which the resulting enthalpy was calculated.
- https://cdn.elifesciences.org/articles/97231/elife-97231-fig6-data2-v1.docx
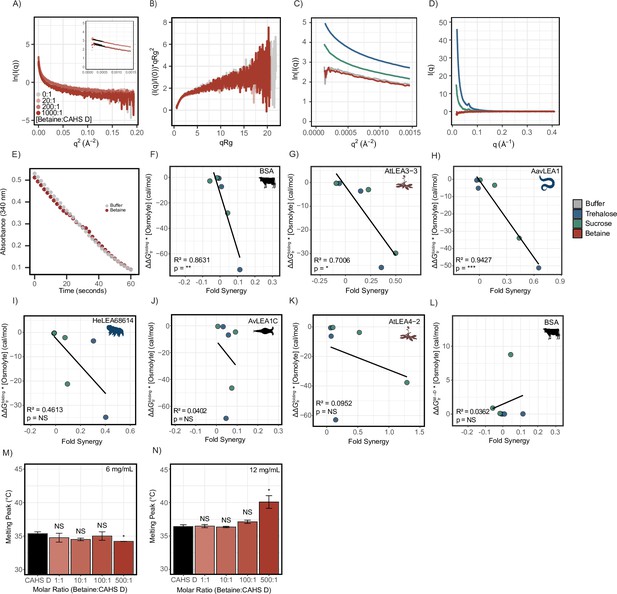
Supplementary figures for Figure 6.
(A) Scattering curves for CAHS D in Tris (gray), 10:1 glycine betaine (light red), 100:1 glycine betaine (medium red), and 1000:1 glycine betaine (dark red). Guinier regions are displayed in the top left with Guinier fits drawn in black. (B) Dimensionless Kratky analysis of the data from (A). (C) Zoomed-in Guinier regions of CAHS D in Tris (gray), 1000:1 glycine betaine (red), 1000:1 trehalose (blue), and 1000:1 sucrose (green). (D) Scattering profiles for CAHS D in glycine betaine, trehalose, and sucrose normalized to the protein’s scattering profile in Tris. (E) Positive controls of LDH in Tris buffer (20 mM, pH 7.0) and highest concentration of glycine betaine used in this study. Absorbance readings at 340 nm measure NADH reporter as a function of time. (F–K) Scatterplots of and synergy for bovine serum albumin (BSA) and late embryogenesis abundant (LEA) proteins. All fits are done using linear regression. All p-values derived from Pearson correlation. (L) Scatterplot of and synergy for BSA. (M, N) Melting temperature for the gel melts as calculated from the differential scanning calorimetry (DSC) thermograms for CAHS D and Betaine:CAHS D mixtures at respective ratios. n = 3, t-test was used for pairwise comparisons of CAHS D with its cosolute mixtures. Organismal icons that are colored blue indicate the species uses trehalose as an endogenous cosolute, brown indicates the species uses both trehalose and sucrose, and black indicates the species uses neither trehalose nor sucrose as an endogenous cosolute.
-
Figure 6—figure supplement 1—source data 1
All data related to Figure 6—figure supplement 1.
- https://cdn.elifesciences.org/articles/97231/elife-97231-fig6-figsupp1-data1-v1.xlsx
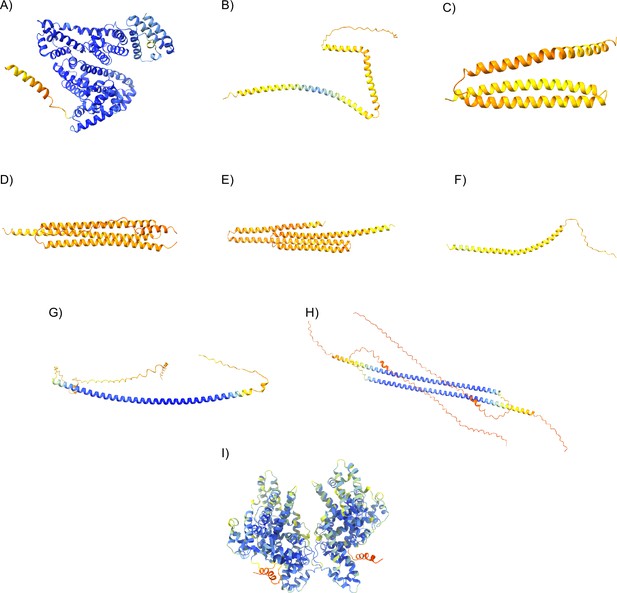
Representative images of the pdb structures obtained from our AlphaFold2 predictions.
All structures are colored by their pLDDT on a scale from 0 (red) to 100 (blue). (A) Monomeric bovine serum albumin (BSA). (B) Monomeric AtLEA3-3. (C) Monomeric AavLEA1. (D) Monomeric HeLEA68614. (E) Monomeric AvLEA1C. (F) Monomeric AtLEA4-2. (G) Monomeric CAHS D. (H) Dimeric CAHS D. (I) Dimeric BSA. See supplementary data for pdb files of each prediction and their exact pLDDT. We note that the structural predictions from AlphaFold2 contain largely ordered structures. We believe this is due to the propensity of these proteins to form helices in the context of drying or when interacting with a client. This has been shown in cases where an IDR contains residual helicity or is folded upon binding (Alderson et al., 2023).
-
Figure 6—figure supplement 2—source data 1
All data related to Figure 6—figure supplement 2.
- https://cdn.elifesciences.org/articles/97231/elife-97231-fig6-figsupp2-data1-v1.zip
Tables
Summary of organisms, disaccharides, and proteins used in this study.
Table displaying the organismal source of representative LEA_4, LEA_1, and CAHS proteins used in this study. In addition, the table displays endogenous cosolute reported in the literature to be co-enriched alongside late embryogenesis abundant (LEA) and cytoplasmic abundant heat-soluble (CAHS) proteins during desiccation in the given organism. The consensus sequence of 11-mer LEA_4 or 20-mer LEA_1 motifs as well as the length of the full-length proteins and predicted disorder using Metapredict (Emenecker et al., 2021) are shown. Shaded areas in the disorder plot correspond to the motif coordinates in the full-length LEA proteins. We note that the reason many of these profiles contain large folded regions is because the amphipathic LEA and CAHS proteins are predicted to form helices, which metapredict infers and incorrectly highlights these regions as ‘folded’ when really they are disordered in isolation.
Organism | Enriched disaccharide during desiccation | Protein family | LEA motif | Representative protein | Length(amino acid) | Motif(%) | Metapredict disorder |
---|---|---|---|---|---|---|---|
![]() | ![]() & ![]() Sucrose (high) & Trehalose (high) Angeles-Núñez and Tiessen, 2010; Fait et al., 2006 | LEA_4 | AKSKADETLES | AtLEA3-3 | 169 | 19.5 | ![]() |
![]() | ![]() (high) Higa and Womersley, 1993; Browne et al., 2004 | LEA_4 | LRDTAAEKLHQ | AavLEA1 | 143 | 30.06 | ![]() |
![]() | ![]() | LEA_4 | LKDKAGSAWNQ | HeLEA68614 | 238 | 26.85 | ![]() |
![]() | X Tunnacliffe and Lapinski, 2003; Lapinski and Tunnacliffe, 2003 | LEA_4 | KDKAAEALDAI | AvLEA1C | 231 | 25.54 | ![]() |
![]() | ![]() & ![]() Sucrose (high) & Trehalose (high) Angeles-Núñez and Tiessen, 2010; Fait et al., 2006 | LEA_1 | AKEKLNIGGA KAQGHAEKTM | AtLEA4-2 | 97 | 73.19 | ![]() |
![]() | ![]() | CAHS | X | CAHS D | 227 | X | ![]() |
-
Table 1—source data 1
LEA motif coordinates presented in Table 1.
- https://cdn.elifesciences.org/articles/97231/elife-97231-table1-data1-v1.xlsx
Reagent type (species) or resource | Designation | Source or reference | Identifiers | Additional information |
---|---|---|---|---|
Strain, strain background Escherichia coli BL21 (DE3) | CAHS D | This study | NA | Sequence information presented in Supplementary file 1 |
Strain, strain background E. coli BL21 (DE3) | AtLEA3-3 | This study | NA | Sequence information presented in Supplementary file 1 |
Strain, strain background E. coli BL21 (DE3) | AavLEA1 | This study | NA | Sequence information presented in Supplementary file 1 |
Strain, strain background E. coli BL21 (DE3) | HeLEA68614 | This study | NA | Sequence information presented in Supplementary file 1 |
Strain, strain background E. coli BL21 (DE3) | AvLEA1C | This study | NA | Sequence information presented in Supplementary file 1 |
Strain, strain background E. coli BL21 (DE3) | AtLEA4-2 | This study | NA | Sequence information presented in Supplementary file 1 |
Recombinant DNA reagent | CAHS D (plasmid) | This study | NA | Cloned via Gibson assembly in pEt28b |
Recombinant DNA reagent | AtLEA3-3 (plasmid) | Twist Bioscience | NA | Cloned in pEt28a |
Recombinant DNA reagent | AavLEA1 (plasmid) | This study | NA | Cloned via Gibson assembly in pEt28b |
Recombinant DNA reagent | HeLEA68614 (plasmid) | This study | NA | Cloned via Gibson assembly in pEt28b |
Recombinant DNA reagent | AvLEA1C (plasmid) | This study | NA | Cloned via Gibson assembly in pEt28b |
Recombinant DNA reagent | AtLEA4-2 (plasmid) | This study | NA | Cloned via Gibson assembly in pEt28b |
Peptide, recombinant protein | At11 | GenScript | NA | |
Peptide, recombinant protein | At22 | GenScript | NA | |
Peptide, recombinant protein | At44 | GenScript | NA | |
Peptide, recombinant protein | Aav11 | GenScript | NA | |
Peptide, recombinant protein | He11 | GenScript | NA | |
Peptide, recombinant protein | Rt11 | GenScript | NA | |
Peptide, recombinant protein | At20 | GenScript | NA | |
Peptide, recombinant protein | BSA | Sigma- Aldrich | 9048-46-8 | |
Peptide, recombinant protein | L-LDH | Sigma- Aldrich | 9001-60-9 | |
Chemical compound, drug | NADH | Sigma- Aldrich | 606-68-8 | |
Chemical compound, drug | Sodium pyruvate | TCI Chemicals | 113-24-6 | |
Chemical compound, drug | D-Trehalose dihydrate | VWR | 6138-23-4 | |
Chemical compound, drug | Sucrose | Sigma- Aldrich | 57-50-1 | |
Chemical compound, drug | Betaine | Cayman Chemical | 107-43-7 | |
Chemical compound, drug | (2,2’-bipyridyl)dichlororuthenium(II)hexahydrate | Sigma- Aldrich | 50525-27-4 | |
Software, algorithm | GraphPad (version 9.5.1) | GraphPad Software | https://www.graphpad.com/ RRID:SCR_002798 | |
Software, algorithm | R-Studio (version 4.1.2) | R-Studio | https://www.r-project.org/ RRID:SCR_001905 | |
Software, algorithm | SOURSOP (version 0.2.4) | Lalmansingh et al., 2023 | https://github.com/holehouse-lab/soursop | |
Software, algorithm | AlphaFold2 (version 2.3.2) | Deepmind, Jumper et al., 2021 | https://colab.research.google.com/github/deepmind/alphafold/blob/main/notebooks/AlphaFold.ipynb | |
Other | Tzero aluminum pans | TA Instruments | 901683.901 |
Additional files
-
Supplementary file 1
Concentration for synergy assays derived from the concentration range of LDH assays.
- https://cdn.elifesciences.org/articles/97231/elife-97231-supp1-v1.pdf
-
Supplementary file 2
Sequences of all peptides and proteins.
- https://cdn.elifesciences.org/articles/97231/elife-97231-supp2-v1.doc
-
MDAR checklist
- https://cdn.elifesciences.org/articles/97231/elife-97231-mdarchecklist1-v1.docx
-
Source code 1
All codes used in this study.
- https://cdn.elifesciences.org/articles/97231/elife-97231-code1-v1.zip