The subthalamic nucleus contributes causally to perceptual decision-making in monkeys
Figures

Behavioral task and model predictions.
(A) Behavioral task. The monkey was required to report the perceived motion direction of the random-dot stimulus by making a saccade toward the corresponding choice target at a self-determined time. (B) Three previous models predicted different patterns of subthalamic nucleus (STN) activity. Sensitive to choice: differential responses for trials ending with different choices. Sensitive to uncertainty: differential responses for trials with different evidence strength.

Subthalamic nucleus (STN) neurons have diverse response profiles.
(A) Activity of three STN neurons (rows) aligned to motion (left) and saccade (right) onsets and grouped by choice × motion coherence (see legend). For motion-onset alignment, activity was truncated at 100 ms before saccade onset. For saccade-onset alignment, activity was truncated before 200 ms after motion onset. (B) Summary of average activity patterns. Each row represents the activity of a neuron, z-scored by baseline activity in a 300 ms window before target onset and averaged across all trial conditions. Rows are grouped by monkey (red and green shown to the right of each panel: monkeys F and C respectively) and sorted by the time of peak values relative to motion onset. Only correct trials were included. (C) Heatmaps of linear regression coefficients for choice (top), coherence for trials with contralateral choices (middle), and coherence for trials with ipsilateral choices (bottom), for activity aligned to motion (left) and saccade (right) onsets. Regression was performed in running windows of 300 ms. Regression coefficients that were not significantly different from zero (t-test, p>0.05) were set to zero (green) for display purposes. Neurons were sorted in rows by the time of peak coefficient magnitude. Only correct trials were included. (D) Time courses of the fractions of regression coefficients that were significantly different from zero (t-test, p<0.05), for choice (black), coherence for trials with contralateral choices (red), and coherence for trials with ipsilateral choices (blue). Dashed line indicates chance level. (E) Time courses of the fractions of non-zero regression coefficients for coherence. Separate fractions were calculated for trials with the preferred (purple) and null (green) choices from choice-selective activity and for all trials from activity that was not choice selective (gray). Only time points after motion onset with fractions > 0.05 for choice-selective activity were included. Dashed line indicates chance level.
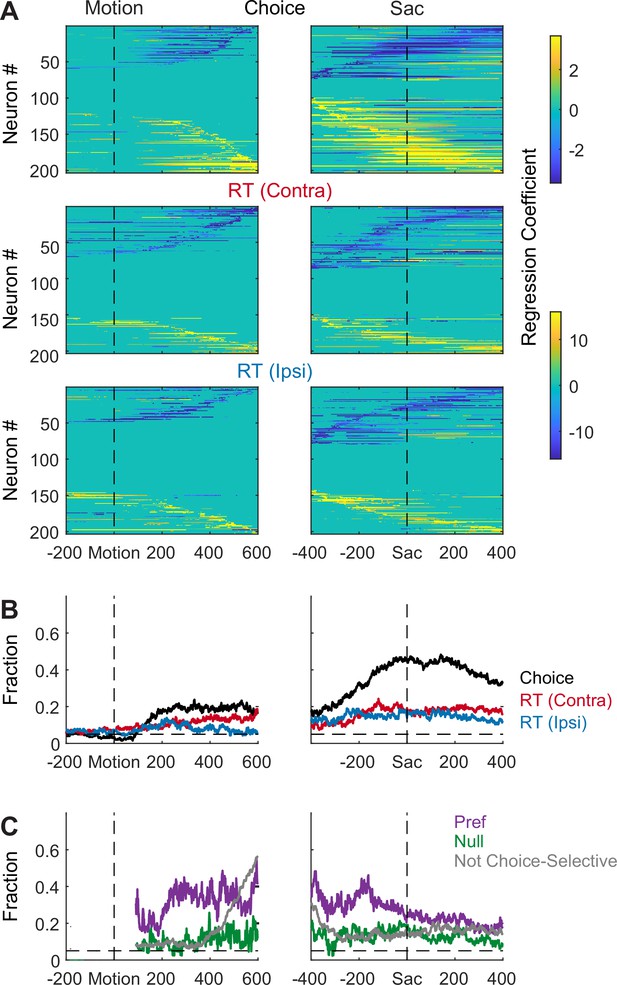
Subthalamic nucleus (STN) activity is modulated by choice and reaction time (RT).
Same format as Figure 2, except using choice and RT as regressors.

Subthalamic nucleus (STN) contains distinct subpopulations.
(A) Three activity vectors that were constructed based on theoretical predictions in Figure 1B and used as seeds for k-means clustering (see Methods). (B) Each panel shows the average activity of neurons in a cluster, same format as Figure 2A. The numbers indicate the cluster size. (C) Visualization of the clusters using the t-distributed stochastic neighbor embedding (t-SNE) dimension-reduction method. (D) Average activity of clusters identified using random-seeded k-means clustering. Same format as Figure 3B. (E) Visualization of the random-seeded clusters in the same t-SNE space.
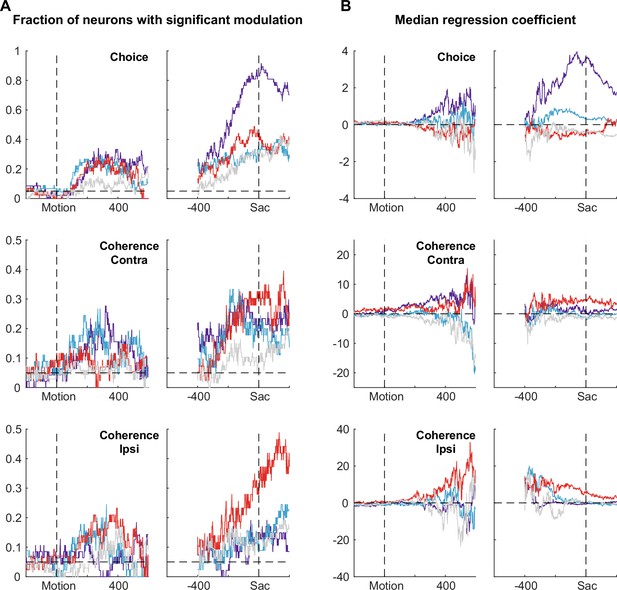
Summary of regression results, separated for different subpopulations.
(A) Fractions of neurons in each category that showed significant modulation (t-test, p<0.05) at each time window by choice (top), coherence for trials with contralateral choices (middle), and coherence for trials with ipsilateral choices (bottom). Dashed horizontal lines indicate the 5% chance level. Colors indicate categories as in Figure 3. (B) Median values of regression coefficients for choice and coherence as a function of time.
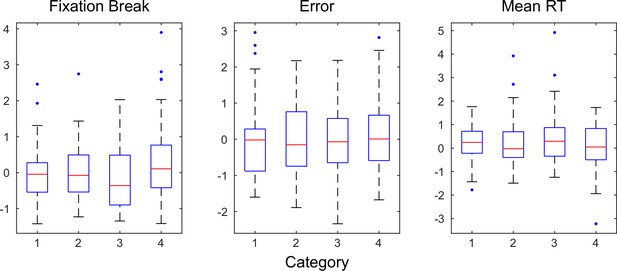
Indices of motivational state did not differ among sessions with different neuron subpopulations.
Panels show the summary of rate of fixation break (left), overall error rate (middle), and mean reaction time (RT) (right) for the four categories identified in Figure 3. All indices were z-scored across sessions for each monkey. Red lines indicate median values. The bottom and top edges of the box indicate the 25th and 75th percentiles, respectively. The whiskers extend to the most extreme data points not considered outliers, and the outliers are plotted individually as dots. ANOVA, p=0.06, 0.91, and 0.29, respectively. No significant difference was observed for each monkey separately (p>0.08 and 0.12 for all indices for monkeys C and F, respectively).
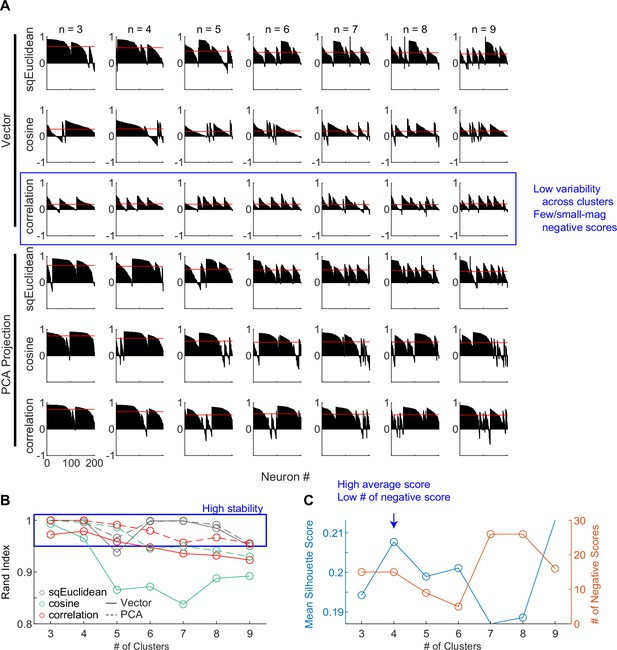
Clustering parameters.
(A) Silhouette plots for clustering results using different combinations of settings. Silhouette scores for neurons are grouped by clusters and sorted. Red lines indicate the mean scores. (B) Average Rand indices for different clustering settings. For each setting, the k-means algorithm was run 50 times, each time picking the best clusters out of 100 repetitions. Higher Rand index indicates greater cluster stability across different runs. Blue box indicates settings with Rand indices > 0.95. (C) Mean silhouette scores and the number of negative scores as a function of number of clusters, using the firing rate vectors and correlation distance. Higher mean score and fewer negative scores indicate better clustering.
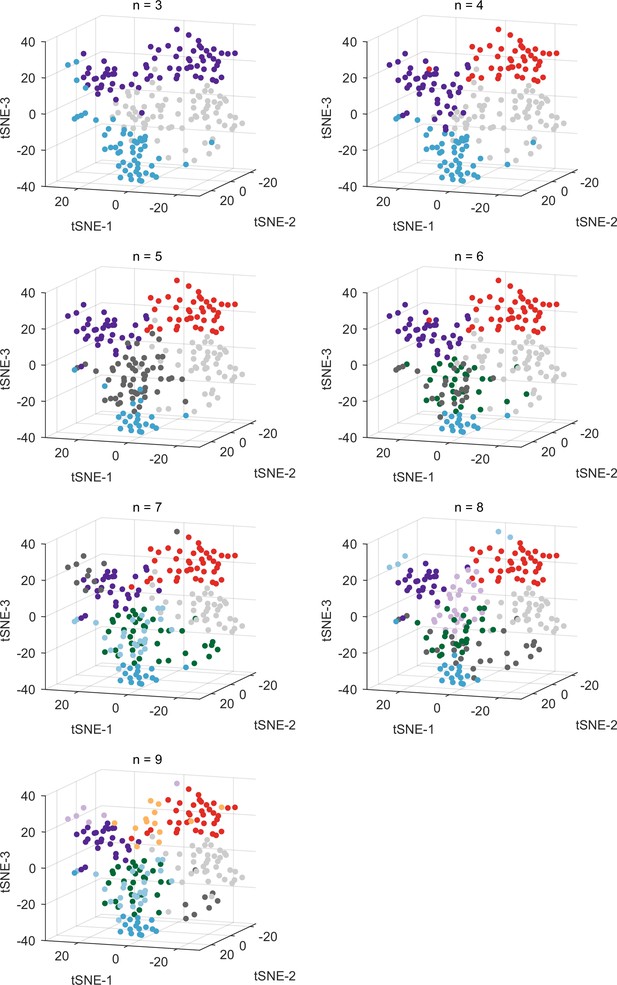
Clustering results using alternative numbers of clusters, visualized in t-distributed stochastic neighbor embedding (t-SNE) space.
Same format as Figure 3E.
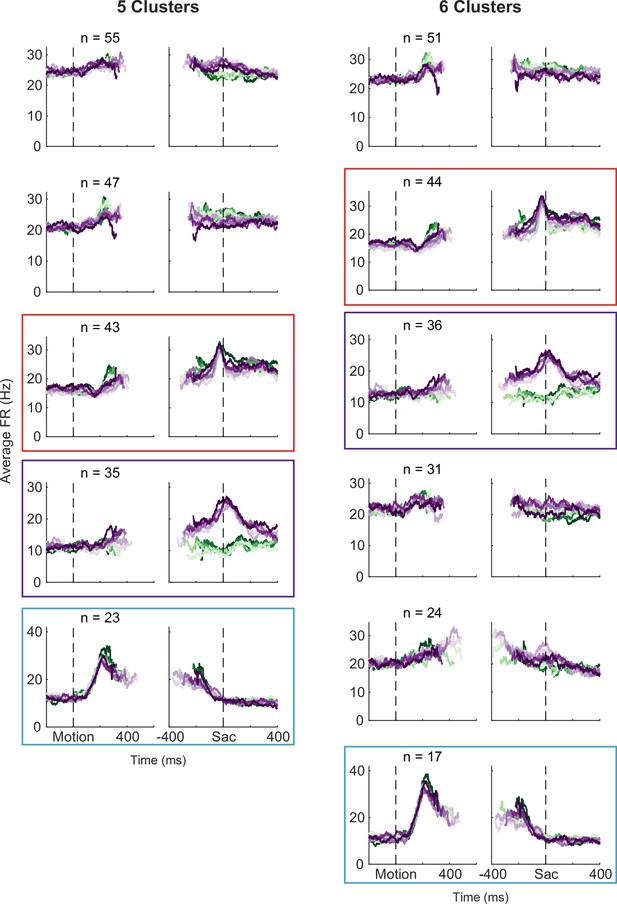
Clustering results using alternative numbers of clusters, visualized as average firing rates for each cluster.
Same format as Figure 3B and D. Colored boxes highlight the clusters corresponding to those identified in Figure 3.
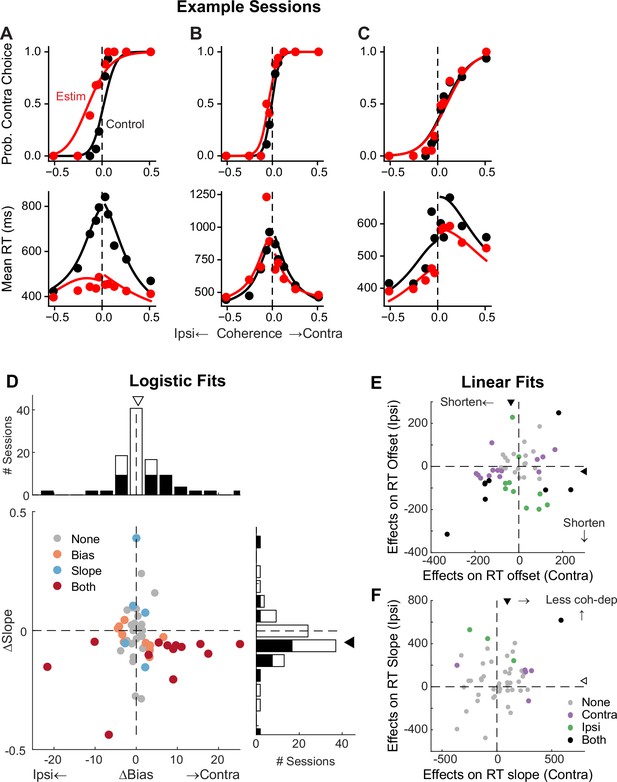
Subthalamic nucleus (STN) microstimulation affects monkeys’ choice and reaction time (RT).
(A–C) Monkey’s choice (top) and RT (bottom) performance for trials with (red) and without (black) microstimulation for three example sessions (A,B: two sites in monkey C; C: monkey F). Lines: drift-diffusion model (DDM) fits. (D) Distributions of microstimulation effects on bias and slope terms of the logistic function. Filled bars in histograms indicate sessions with significant modulation of the specific term (bootstrap method). Triangles indicate the median values. Filled triangle: Wilcoxon signed-rank test for H0: median = 0, p<0.05. (E and F) Summary of microstimulation effects on the offset (E) and slope (F) terms of a linear regression fit to RT data. Two separate linear regressions were performed for the two choices (Ipsi/Contra, as indicated). Triangles indicate the median values. Filled triangles: Wilcoxon signed-rank test, p<0.05.
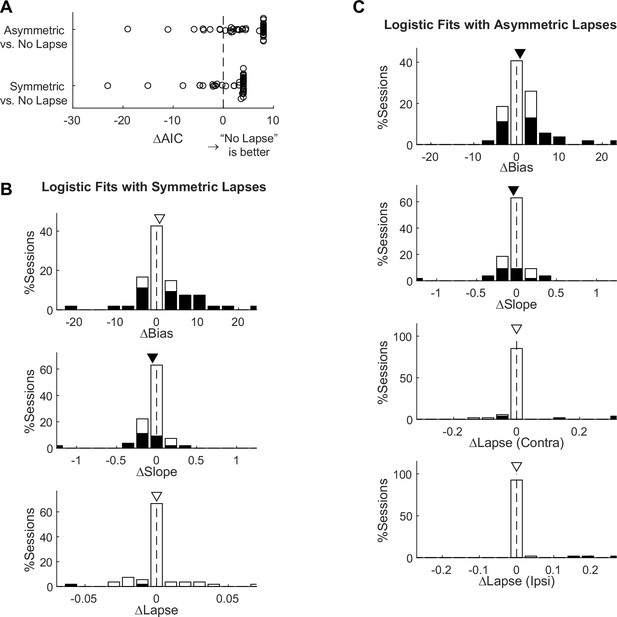
Comparison of different logistic models.
(A) The No Lapse model was associated with the lowest Akaike information criterion (AIC) for most sessions. The Symmetric Lapse model was associated with lower AICs for 12 sessions. The Asymmetric Lapse model was associated with lower AICs for 8 sessions. (B) Histograms of microstimulation effects on bias, slope, and lapse terms in the Symmetric Lapse model. (C) Histograms of microstimulation effects on bias, slope, and two lapse (for each choice) terms in the Asymmetric Lapse model. Same format as the histograms in Figure 5D.

Subthalamic nucleus (STN) microstimulation affected multiple computational components in the drift-diffusion model (DDM).
(A) Illustration of the DDM. Red/black lines represent across-trial mean/single-trial example of the evidence (top) and drift rate (bottom). Blue lines represent the collapsing decision bounds. (B) Distribution of the difference in Akaike information criterion (AIC) between the None and Full models. Red dashed line indicates the criterion for choosing the Full model: AIC difference = 3. (C) Histograms of microstimulation effects on DDM parameters. Each histogram included only sessions in which the Full model outperformed the corresponding reduced model (e.g. the histogram for parameter a included only sessions in which AICNoA – AICFull >3 and AICNone – AICFull >3). Triangles indicate median values. Filled triangles: Wilcoxon signed-rank test, p<0.05. (D) Summary of microstimulation effects on all parameters, for sessions in which at least one significant effect was present. Sessions were sorted by the prevalence and sign of the effects.
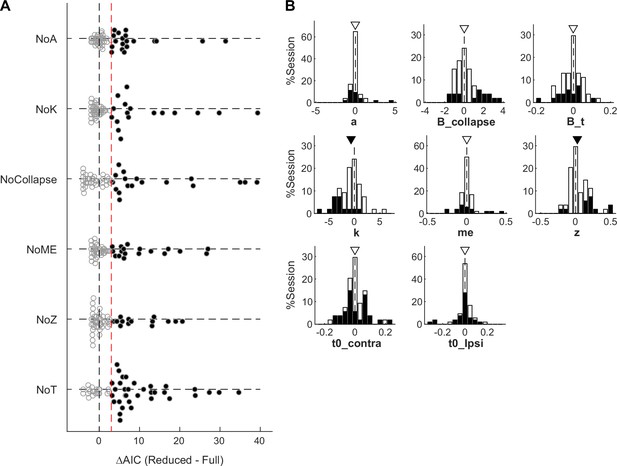
Supplemental DDM fitting results.
(A) Differences in Akaike information criterion (AIC) between reduced and Full models. Filled circles indicate sessions for which AICReduced – AICFull >3 (red line). Note that for three sessions, the Full model outperformed the None model but not any of the reduced models. (B) Histograms of difference in drift-diffusion model (DDM) parameters between trials with and without microstimulation. Filled bars represent sessions considered to show significant microstimulation effects on the given parameter, based on AIC comparisons. Triangles indicate median values. Filled triangles: Wilcoxon signed-rank test, p<0.05.

Different subthalamic nucleus (STN) subpopulations are intermingled.
Locations of STN neurons, color-coded by clusters based on random-seed clustering (same as Figure 3D). The medial-lateral (ML) values were jittered for better visualization of neurons recorded along the same track and at similar depths. Anterior-posterior (AP) levels were relative to the anterior commissure. ML and depth levels were relative to the center of the recording chambers.

Subthalamic nucleus (STN) microstimulation effects depend partially on neural clusters.
(A) Average activity at stimulation sites, grouped by four clusters based on the clusters in Figure 3D. (B) Fractions of significant microstimulation effects for sites with the presence of each neuron cluster. Significance was based on Akaike information criterion (AIC) comparison between reduced and Full models. (C) Microstimulation effects grouped by neuron cluster. Same format as Figure 6D.

Firing rates of a single STN neuron did not substantially influence decision-related DDM parameters.
(A) Differences in AIC between DDM variants that included firing rate-dependent terms and the standard DDM. Red dahsed line: difference = -3. Each column represents results from one unit. (B) Fitted coefficients for firing rate-related terms were near zero. Note the range of y axis. Values for the top and bottomw panels were obtained from "DV"- and "Bound"-type models, respectively. See text for more details.
Tables
Reagent type (species) or resource | Designation | Source or reference | Identifiers | Additional information |
---|---|---|---|---|
Software, algorithm | Matlab | Matlab | RRID:SCR_001622 | |
Software, algorithm | Python | Python | RRID: SCR_008394 |
Additional files
-
Supplementary file 1
Indices of motivational state did not correlate with microstimulation effects.
p-Values are raw values from Pearson correlation, not corrected for multiple testing.
- https://cdn.elifesciences.org/articles/98345/elife-98345-supp1-v1.docx
-
MDAR checklist
- https://cdn.elifesciences.org/articles/98345/elife-98345-mdarchecklist1-v1.pdf