An adaptable, reusable, and light implant for chronic Neuropixels probes
Figures
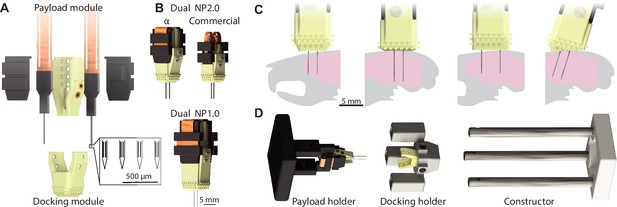
The Apollo implant and its flexible design.
(A) Exploded view of the implant showing the two modules: the payload module, which accommodates up to two Neuropixels probes (protected by two lids), and the docking module. Zoom-in: scaled illustration of the tip of a 4-shank Neuropixels 2.0α probe. Each shank is 75 μm wide, with 250 μm center–center distance between shanks and 15/32 μm vertical/horizonal distance between electrode sites on each shank. (B) Assembled view of the implant, for 2.0α and 2.0 (top) and 1.0 (bottom) probes. (C) Illustration of implant flexibility. Compared with the standard model (left), the length of exposed probes (middle-left), spacing between probes (middle-right), and implantation angle (right) can all be adjusted with preset parameter changes in the software files (Video 1). (D) Constructor for the assembly of the payload and docking modules. The docking holder slides along the posts of the constructor, and optimally aligns with the payload module being held by the payload holder. This effectively eliminates the risk of breaking the shanks when combining modules.
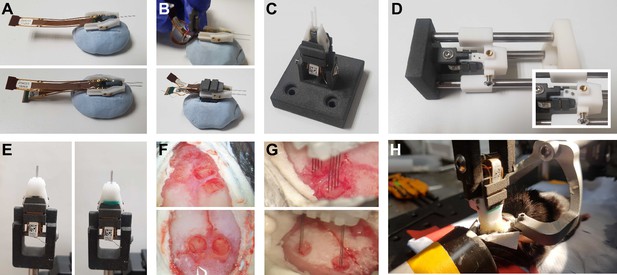
Assembly and implantation.
(A) Initial stage of payload assembly. The payload module is stabilized on Blue Tack while the first (top) and second (bottom, optional) probes are secured with epoxy. (B) Each flex cable is first folded into a cavity in the lid (top) before the lid is glued in place (bottom). (C) The completed payload module fixed in its holder before being attached to the constructor. (D) The combination of payload and docking modules in the constructor. Inset: after the screws have been added to combine the modules. (E) Before (left) and after (right) residual gaps were filled with Kwik-Cast. (F) Example of dual craniotomies performed with a drill (top – premotor cortex and striatum) or biopsy punch (bottom – bilateral superior colliculus). (G) Dual 4-shank probes at the initial stage of insertion into craniotomies performed with drill (top) or biopsy punch (bottom). (H) Finalized implant in anesthetized animal, after the docking module has been cemented to the skull.
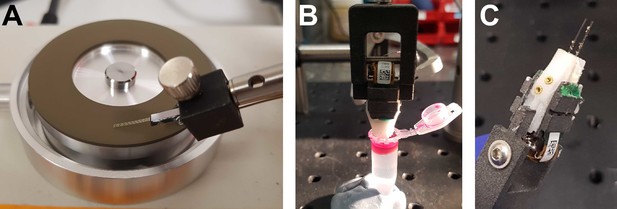
Additional protocol steps.
(A) Probes were sharpened before assembling the implant using a microgrinder (Narishige EG-45). (B) Before most implantations, probes were coated with a fluorescent marker (Vybrant V22888 or V22885, Thermo Fisher) by dipping them directly into the solution. (C) Example of detritus that can adhere to the probe shanks during explantation. This should be cleaned before reusing the implant (see Methods).
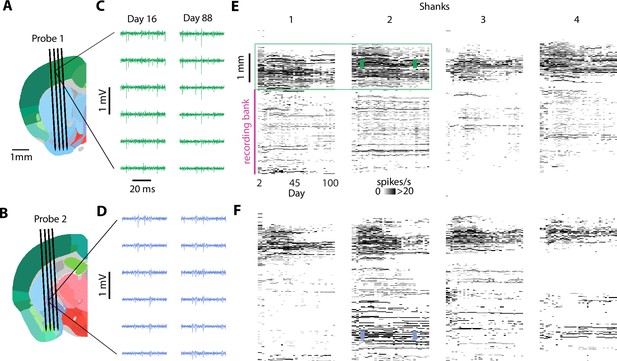
Dual implant providing months of recordings.
(A, B) Insertion trajectories of two simultaneously implanted 4-shank Neuropixels 2.0α probes, with respect to brain anatomy (Allen Mouse Brain Atlas, Wang et al., 2020). (C, D) Raw signal (bandpass filtered between 400 Hz and 9 kHz) across six channels, on day 16 and 88 post-implantation. (E, F) Number of spikes per second versus depth along the probe (y-axis) and days from implantation (x-axis) for the same implantation shown in A–D. The total number of spikes per second (across all detected units) is binned across depths for each day (20 µm bins). This mouse was recorded while head-fixed.
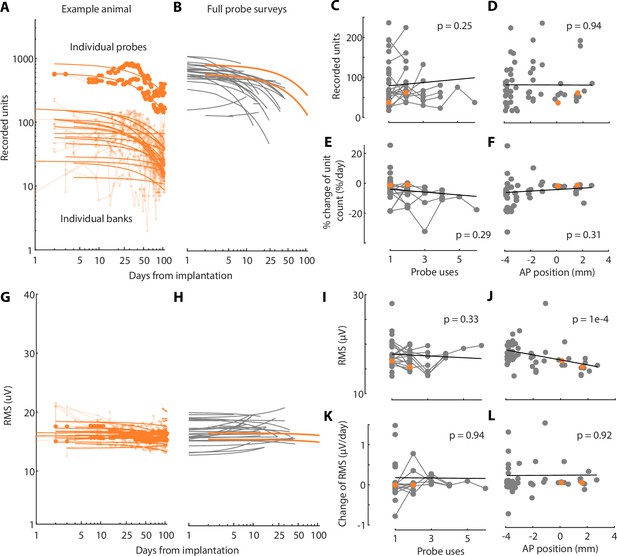
Recording stability and implant reuse.
(A) Total number of recorded units across days for individual channel banks (thin lines), and across each probe (thick lines), for the same implantation as in Figure 3. Lines: logarithmic fits. (B) Logarithmic fits across all implantations where a full survey of the probe was regularly performed (orange, implantation from Figure 3). Full probe surveys were performed only in the primary lab (head-fixed conditions). (C) Unit count versus number of implantations. Connected dots represent single probes, reused up to six times. No criteria were used to select probes for reuse, and this decision was based solely on probe availability and experimental need. Slopes were quantified on individual banks and averaged for each probe before applying a linear mixed-effects model (thick line). (D) Unit count versus antero-posterior position of the insertion, relative to bregma. (E, F) Same as (C, D) but for the slope of the unit count decay. (G–L) Same as (A–F) but for the root-mean-square (RMS) value of the raw signal. For C–F and I–L, all mice are used and shown (head-fixed and freely moving conditions). Rats were excluded because their insertion coordinates cannot be matched with the mice, but their individual results are shown in Figure 4—figure supplement 1. All p-values shown come from a linear mixed-effects model.
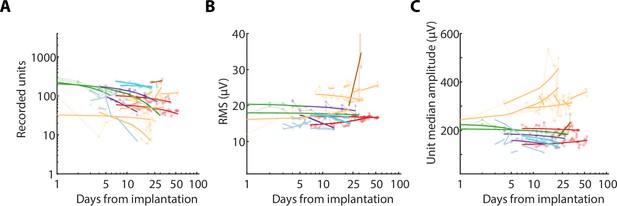
The amplitude of the recorded neurons is stable across days and probe reuses.
(A) Number of recorded units as a function of days from implantation, for all the secondary labs. Each color corresponds to a different lab, and each line is a different bank for a specific probe and animal. (B) Root-mean-square (RMS) values as a function of days from implantation, for all the secondary labs. (C) Median unit amplitude as a function of days from implantation, for all the secondary labs. The high amplitude values (yellow) come from the recordings in rats. All secondary labs used this implant as part of their own study, explaining the diversity of length and types of protocols.
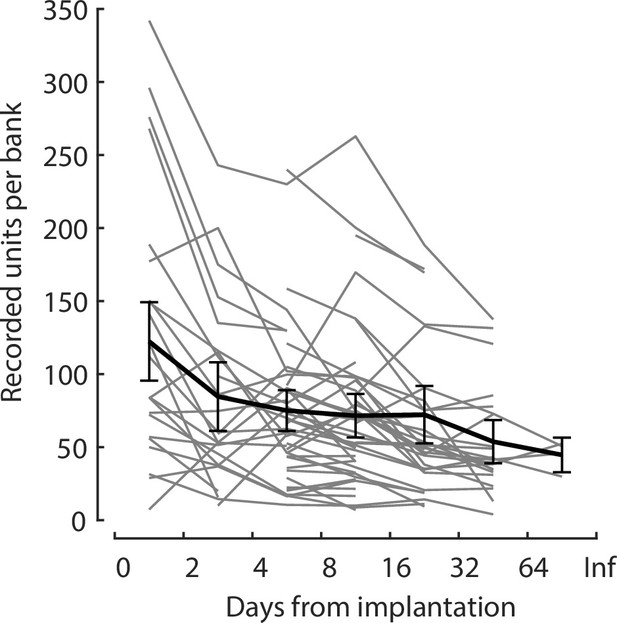
Stability of unit count across days.
Number of recorded units per bank as a function of days, averaged across all banks for each animal (gray) or averaged across animals (black, mean ± SEM, n changing across bins).
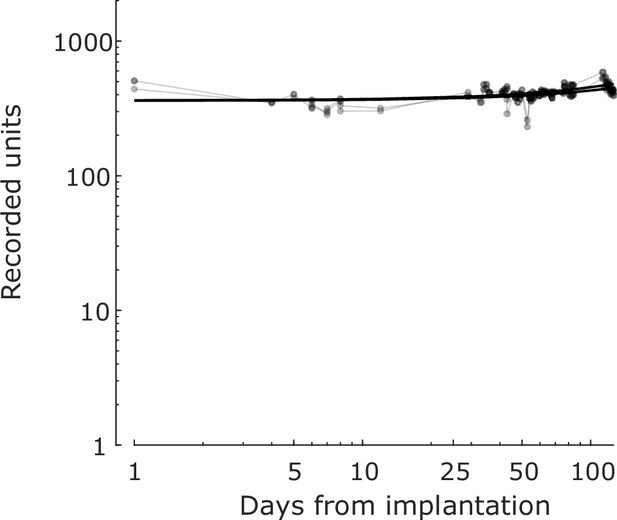
Recording stability on the mouse where the craniotomy was covered with silicon.
As in Melin et al., 2024, a drop of silicon (Kwik-Sil) was added to cover the craniotomy before closing the implant with cement. Although anecdotal, the stability of recorded units in this mouse was excellent, suggesting that silicon may improve implant stability.
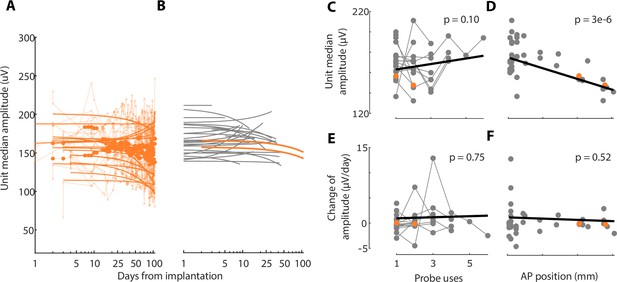
The amplitude of the recorded neurons is stable across days and probe reuses.
(A) Median unit amplitude across days for individual channel banks (thin lines), and across each probe (thick lines), for the same implantation as in Figure 3. Lines: logarithmic fits. (B) Logarithmic fits across all implantations where a full survey of the probe was regularly performed (orange, implantation from Figure 4). (C) Median unit amplitude versus number of implantations. Connected dots represent single probes, reused up to six times. The slope is quantified with a linear mixed-effects model (thick line). (D) Median unit amplitude versus antero-posterior position of the insertion, relative to bregma. (E, F) Same as (C, D) but for the change in amplitude over days.
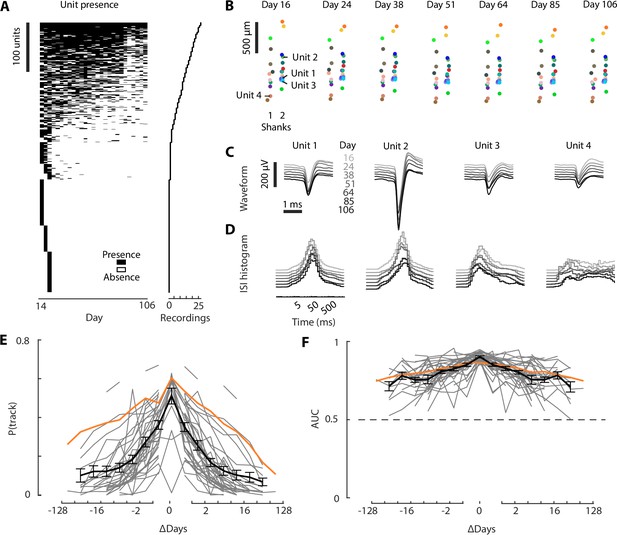
Neurons recorded across days and months.
(A) Unit presence across all recordings (left). Only the units present in at least one of the first 3 days are shown for ease of visualization. Units are ordered by the number of recordings in which their presence was detected (right). (B) Spatial layout of the population of neurons tracked across days. In this example, the recording sites were spanning two shanks of a 4-shank 2.0α probe (green rectangle in Figure 3E). (C) Average waveforms of four example tracked units, computed for 7 days across a 13-week period. (D) As in C, but showing the inter-spike interval (ISI) histogram of each unit on each day. (E) Probability of tracking a unit as a function of days between recordings, for individual mice (gray), including example from A to D (orange), or the average across all mice (black, mean ± SEM across datasets). (F) The average AUC values when comparing the ISI histogram correlations of tracked versus non-tracked neurons. Colors same as (E).
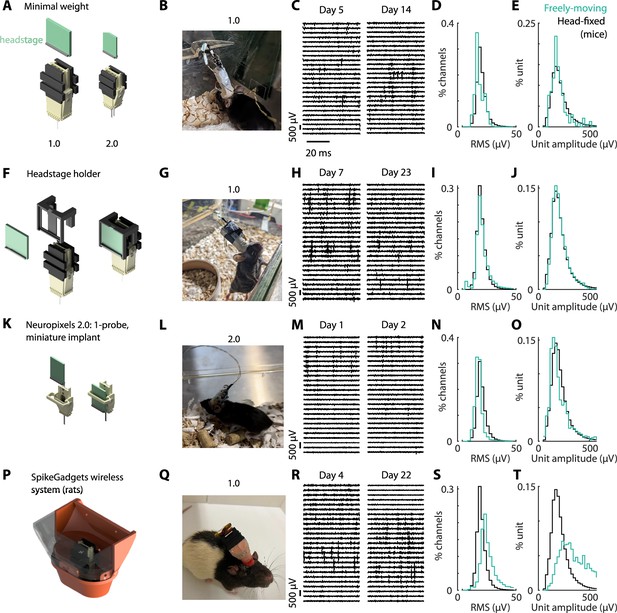
Use in freely behaving animals.
(A) Neuropixels 1.0 and 2.0α were used with freely moving mice. The headstage was suspended by the wire above the implant. (B) Animal freely moving with the 1.0 version of the implant, with headstage attached. (C) Raw signal (bandpass filtered between 400 Hz and 9 kHz) across multiple channels of increasing depth, on 2 days post-implantation. (D) Mean distribution of the root-mean-square (RMS) value across channels, averaged across all recordings in head-fixed mice (black, n = 2 mice) and freely moving (cyan, n = 35 mice). (E) Same as (D), but for the distribution of the units’ amplitude. (F) As in (A), but with an additional headstage holder for Neuropixels 1.0 (n = 4 mice). (G–J) As in (B–E) but for recordings with the headstage holder from (D). (K) Miniature, 1-probe implant for Neuropixels 2.0, with a headstage holder (n = 8 mice with both 2.0 and 2.0α probes). (L–O) As in (B–E) but using the modified design from (G). (P) Configuration for rats, with a casing to protect the implant (SpikeGadgets – without the lid, n = 3 rats). The final configuration comprises the wireless recording system. (Q–T) As in (B–E) but with the apparatus from (P), recorded in rats. Not that the reference head-fixed data is from mice.
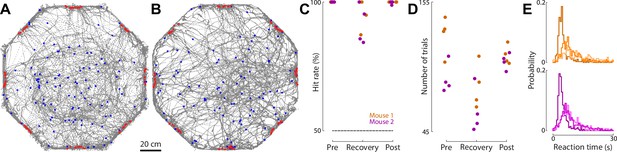
Effects of implantation on performance in a freely moving task.
(A) Trajectories (gray) of single mouse in an example session prior to implantation. Blue and red dots indicate mouse position at the start and end of each trial. (B) As in (A), but for an example session post-implantation. (C) The hit rate (percentage of correctly completed choices) made by two mice (magenta and brown) in the four sessions immediately before implantation (Pre), immediately after implantation (Recovery), and the subsequent four sessions after this recovery period (Post). n = 2 mice, 4 sessions per mouse at each time period. (D) As in (C), but for the number of trials per session. (E) The probability of each reaction time for the same sessions in A and B, separated by mouse.
Videos
Guide to altering the Apollo parts to a particular implantation.
This video guide demonstrates how to change the shape of the implant using parameters in Autodesk Inventor software—facilitating changes in inter-probe distance, penetration depth, and angle of implantation.
Tables
Implant weight depends on probe version and material.
The weight for each implant version. We find these to vary with each print (5%), and with different services (10–15%). For consistency, these weights are calculated from part volume and the material density. The ‘Standard’ implant comprises PA12-Lids and Rigid4000-Payload/Docking modules. This is the most common implant used by experimenters, but the PA12-only implant has been used to reduce weight further. The total weights do not include the cement (0.2 g) to fix the probes to the payload module.
Weights of implants (g) | ||||||
---|---|---|---|---|---|---|
NP 1.0 | NP 2.0-Alpha | NP 2.0-Commercial | ||||
Nylon PA12 | Rigid4000 Resin | Nylon PA12 | Rigid4000 Resin | Nylon PA12 | Rigid4000 Resin | |
Payload | 0.36 | 0.46 | 0.29 | 0.38 | 0.18 | 0.23 |
Docking | 0.35 | 0.45 | 0.26 | 0.34 | 0.22 | 0.29 |
Lid (x2) | 0.61 | 0.79 | 0.41 | 0.52 | 0.25 | 0.32 |
Probe (x2) | 0.80 | 0.38 | 0.33 | |||
Screws (x4) | 0.09 | 0.09 | 0.09 | |||
Threads (x4) | 0.08 | 0.08 | 0.08 | |||
PA12 total | 2.29 | 1.51 | 1.15 | |||
Standard total | 2.50 | 1.67 | 1.26 |
Additional files
-
MDAR checklist
- https://cdn.elifesciences.org/articles/98522/elife-98522-mdarchecklist1-v1.pdf
-
Supplementary file 1
Table of all animals used in the study.
This table provides extensive information on the animals used in the study, such as the implant date, probe number and type, number of recordings, or whether an issue was encountered during the implantation period.
- https://cdn.elifesciences.org/articles/98522/elife-98522-supp1-v1.xlsx