Sub-cone visual resolution by active, adaptive sampling in the human foveola
Figures
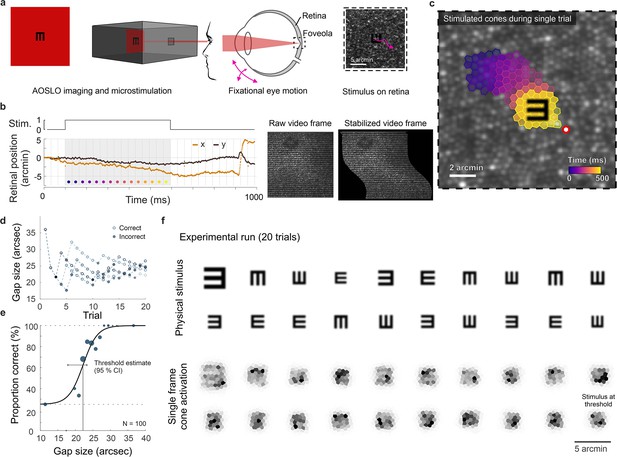
Cone-resolved adaptive optics micro-psychophysics.
(a) Schematic of cell-resolved visual acuity testing in the human foveola with an adaptive optics scanning laser ophthalmoscope (AOSLO). Stimuli were dark Snellen-E optotypes presented at variable sizes and four orientations in the center of the 788 nm AOSLO imaging raster. Participants responded by indicating stimulus orientation during natural viewing, i.e., unrestricted eye motion. (b) Exemplary single trial retinal motion trace and strip-wise image stabilization of a single AOSLO frame (shown here during a microsaccade for better visibility). Trials containing microsaccades or blinks during the 500 ms stimulus presentation (gray shaded area) were excluded. The x-axis grid represents individual video frames (33 ms). (c) Foveolar retinal cone mosaic with a exemplary single trial retinal motion across the stimulus. Time is represented by color from stimulus onset to offset (purple to yellow). The cone density centroid (CDC) is shown as a red circle with white fill. (d) Typical psychophysical data of five consecutive runs in one eye. Each run followed a QUEST procedure with 20 trials. (e) Psychometric function fit to the data (about 100 trials). Acuity thresholds were estimated at 62.5% correct responses. (f) Exemplary retinal images (upper rows) and corresponding cone activation patterns (lower rows) of one experimental run (20 trials from top left to bottom right). Cone activation patterns are shown for a representative single frame. See Videos 1 and 2 for a real-time video representation.
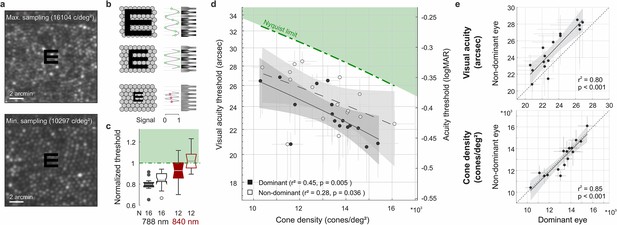
Visual acuity depends on foveolar sampling capacity.
(a) Foveolar cone mosaics of the two eyes with highest and lowest cone densities, overlayed with the physical stimulus at an average threshold size (24 arcsec). (b) Nyquist limit: critical details equaling or larger than the spacing of cones are resolvable. (c) Visual acuity thresholds measured with 788 or 840 nm infrared light, normalized to the eyes’ Nyquist limits. (d) Correlation between participant’s individual visual acuity thresholds and cone density. Thresholds exceeded the Nyquist sampling limit and were significantly lower in eyes with higher cone densities. Dominant eyes are shown as filled, and non-dominant eyes as open markers. The gray horizontal and vertical bars at each point represent standard deviations of sampling cone density and the 95% confidence intervals for acuity thresholds. The theoretical Nyquist limit is represented by a dashed green line. (e) Correlation between dominant and non-dominant eyes in visual acuity (top) and cone density (bottom). Dominant eyes reached, on average, 1.5 arcmin lower thresholds than non-dominant eyes, whereas cone density (at the retinal locations that sampled the stimulus) was very similar between fellow eyes.
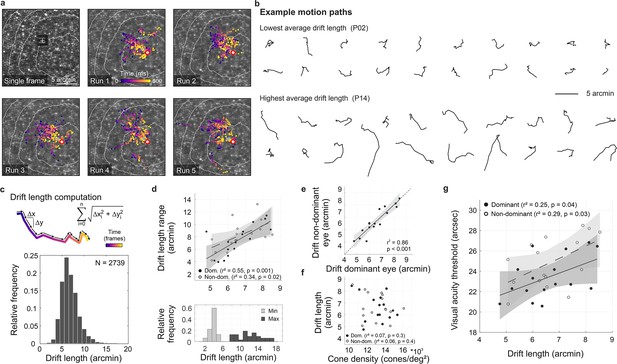
Fixational drift and the contribution to visual acuity.
(a) Ocular drift during stimulus presentation (participant 16, left eye). Single adaptive optics scanning laser ophthalmoscopy (AOSLO) frame captured during Snellen E presentation (top left) and all single stimulus positions (colored dots) of five experimental runs shown on the corresponding cone mosaic (panels 2–6). White iso-lines delimit cone density percentile areas (90th to 50th percentile visible). Time is represented by color from stimulus onset to offset (purple to yellow). Individual drift trajectories for all eyes are shown in Figure 3—figure supplement 1. (b) Individual motion traces highlighting intra- and inter-subject drift variability. Traces are from one run in the participant with the lowest (upper rows) and highest (lower rows) average drift lengths. (c) Computation of drift length as a sum of interframe motion vectors (top) and the relative frequency of occurrences among all participants and trials (bottom). (d) Median drift length and drift length range showed a moderate correlation in dominant as well as non-dominant eyes (top). The minimum drift length was similar between participants (3.8±0.8 arcmin) whereas the maximum length varied about three times as much (12.0±2.7 arcmin). (e) Drift lengths in fellow eyes had a very strong correlation. (f) Cone density and drift length did not show a significant correlation in dominant or non-dominant eyes. (g) The median drift length had a moderate correlation with visual acuity threshold in dominant as well as non-dominant eyes. Dominant eyes are indicated by filled, non-dominant eyes by open markers.
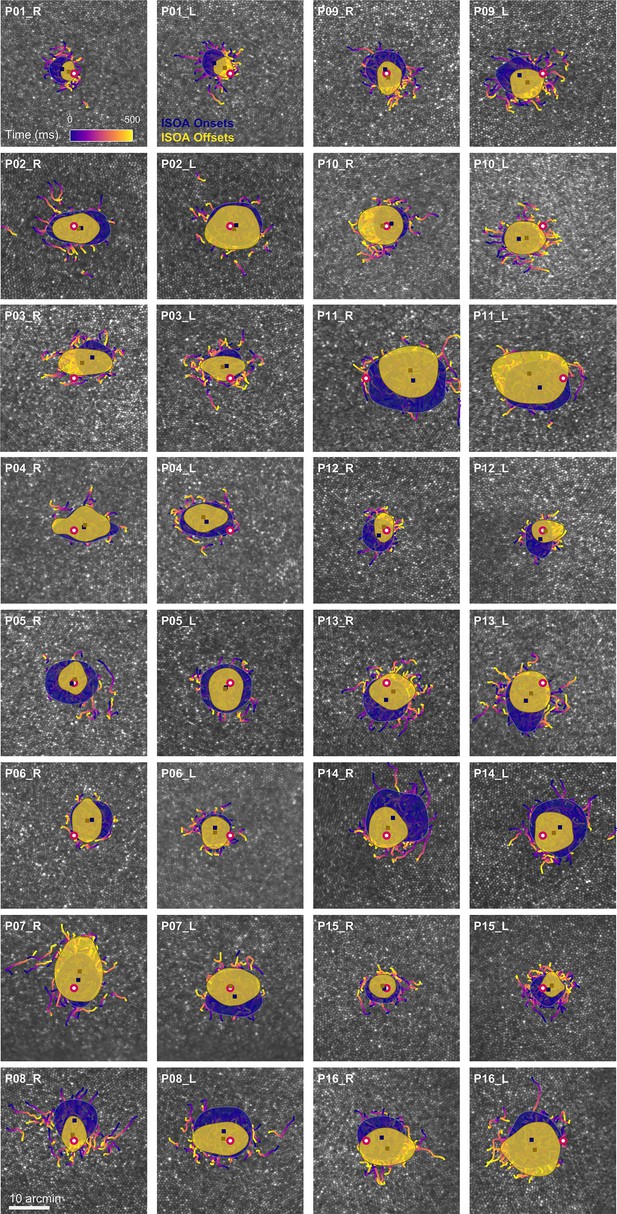
Drift trajectories on foveolar mosaics.
Participants (P01-16) are sorted according to acuity thresholds in their dominant eye (best to worst). All single trial motion traces are shown on the corresponding cone mosaic, color-coded by elapsed time. One-SD isoline areas (ISOA) are shown for all stimulus onset (blue) and offset (yellow) locations. The medians of all onset and offset locations are shown as blue and yellow squares, respectively. The cone density centroid (CDC) is indicated by a red circle with white fill.
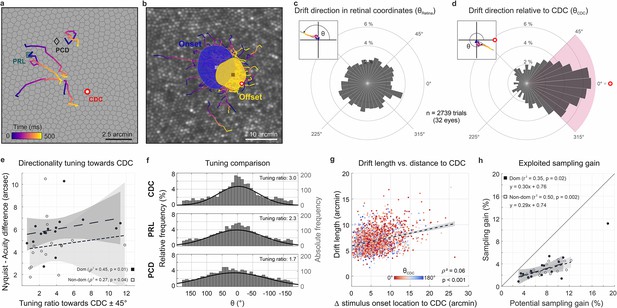
Drift moves stimuli to higher cone density areas.
(a) Five exemplary motion traces relative to cone density centroid (CDC), preferred retinal locus (PRL), and peak cone density (PCD) location on the Voronoi tessellated cone mosaic of one participant. (b) All single trial motion traces of one eye are shown on the corresponding cone mosaic (95 trials containing drift only). One-SD isoline areas (ISOA) are shown for all stimulus onset (blue) and offset (yellow) locations, indicating a trend of directional drift towards higher cone densities during 500 ms stimulus presentation. (c) Polar histogram of all individual motion traces (n=2739) shows the relative frequency of motion angles, θRetina, between the start (coordinate center) and end of motion in retinal coordinates. The inset indicates θ sign. (d) Same data as in c, where θCDC was computed relative to the line connecting drift start location and CDC, see inset. The pink quarter indicates the angular space used for the computation of the tuning ratio. For more details on the drift directionality of individual eyes, see Figure 1. (e) The difference between the acuity threshold and Nyquist limit showed a significant trend to be larger for stronger directionality tuning. The tuning ratio was computed as the ratio between the relative frequency of intra-participant drift motion towards the CDC (±45 deg) and the average of drift motion towards the remaining three quadrants. (f) Relative frequency of drift direction relative to CDC (top), PRL (middle), and PCD (bottom), respectively. For more details on the temporal progression of drift directionality, see Figure 2. (g) Across all participants and trials, drift length correlated with stimulus onset distance from CDC. There was no clear effect of stimulus onset distance on motion directionality (data color corresponding to θCDC). (h) The achieved sampling gain due to the performed drift motion is significantly correlated to the potential sampling gain in individuals. In both dominant and non-dominant eyes the potential sampling gain is on average exploited by 30%, respectively. Due to shifting the CDC towards the stimulus, participants had different PRLs for a sustained fixation task and the visual resolution task (see Figure 3).

Individual drift directionality.
Sorted according to their dominant eyes cone density centroid (CDC) to preferred retinal locus (PRL) distance (largest to smallest) eyes drift directionality is shown relative to the CDC. The CDC is indicated by a red circle with white fill. Participant numbers (P01 to P16) are assigned based on the dominant eye’s visual acuity threshold (best to worst). The upper left plot shows the angular labels exemplarily for all eyes. The tuning ratio was computed as the ratio between the relative frequency of intra-participant drift motion towards the CDC (±45 deg), indicated by the pink quarter, and the average of drift motion towards the remaining three quadrants.

Time course of drift directionality.
The angles θ cone density centroid (CDC), θ preferred retinal locus (PRL), and θ peak cone density (PCD) for all eyes were computed over time, relative to the stimulus onset, and are shown after 33 ms (one frame), 165 ms (five frames), 330 ms (10 frames), and 462 ms (14 frames). The respective tuning ratio (TR) is shown in the bottom part of each subplot. The tuning ratio was computed as the ratio between the relative frequency of inter-participant drift motion towards the CDC (±45 deg) and the average of drift motion towards the remaining three quadrants. For all cases, the circular data are not distributed uniformly around the circle (Rayleigh test, p<0.001, with increasing significance for longer durations). The distributions were compared against each other by the Kolmogorov-Smirnov test. Only CDC vs. PRL (33 ms) and PRL vs. PCD (33 ms) show no statistically significant difference. The distribution towards CDC compared to PCD is significantly different for 33 ms (p=0.002). For all other time points, all distributions are significantly different from each other, with increasing significance for longer durations (for 462 ms, all p<0.001).
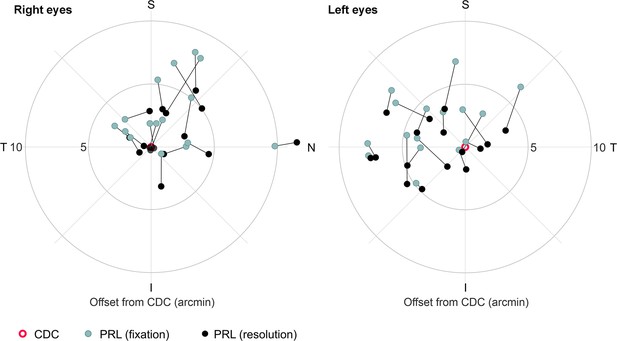
Different retinal locations used in a fixation or resolution task.
Median preferred retinal locus (PRL) locations of all participants’ right and left eyes plotted in retinal coordinates relative to the cone density centroid (CDC). Retinal locations are plotted in teal for the fixation task and in black for the resolution task. A black line connects the retinal locations of the same participant.

Drift length does not depend on stimulus sizes close to threshold.
All experimental trials were sorted by stimulus size and then grouped into percentiles for each participant (left). Additionally, 10 % of trials with stimulus sizes just above or below threshold are shown for comparison (right). For each group, median drift lengths (z-scored) are shown as box and whiskers plot. Drift length was not significantly different across groups.

Diffusion coefficient (D) and the relation to visual acuity (see Figure 3 e-g for comparison to drift length).
(a) D was strongly correlated between fellow eyes. (b) Cone density and D were not significantly correlated. (c) The median D had a moderate correlation with visual acuity thresholds in dominant as well as non-dominant eyes. Dominant eyes are indicated by filled, nondominant eyes by open markers.

Reproducibility of CDC and PCD location in comparison.
Two retinal mosaics which were recorded at two different timepoints, maximum 1 year apart from each other, were compared for 16 eyes. The retinal mosaics were carefully aligned. The retinal locations for CDC and PCD that were computed for the first timepoint were used as the spatial anchor (coordinate center), the locations plotted here as red circles (CDC) and gray diamonds (PCD) represent the deviations that were measured at the second timepoint for both metrics.
Videos
Video recordings of one experimental run in the eye with highest sampling density.
All successive single trial videos (left) and the Voronoi mosaic of cells colored with their respective amount of cone activation (right).
Video recordings of one experimental run in the eye with lowest sampling density.
All successive single trial videos (left) and the Voronoi mosaic of cells colored with their respective amount of cone activation (right).
Decrease of ISOA across trials during the 500 ms stimulus presentation.
The area of stimulus onsets between trials shows a stronger variation than the area of stimulus offsets.