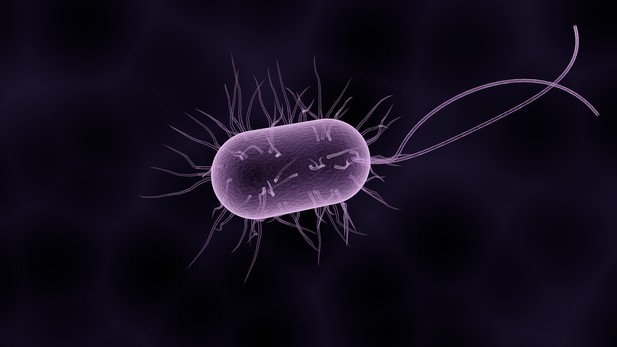
To be able to move in their environment, bacteria often carry one or several propellers-like molecular motors that include a long external filament. Image credit: Public domain
Bacteria are so small that for them, making their way through water is like swimming in roofing tar for us. In response, these organisms have evolved a molecular machine that helps them move in their environment. Named the bacterial flagellum, this complex assemblage of molecules is formed of three main parts: a motor that spans the inner and outer membranes of the cell, and then a ‘hook’ that connects to a long filament which extends outside the bacterium. More precisely, the motor is formed of the stator, an ion pump that stays still, and of a rotor that can spin. Different rings can also be present in the space between the inner and outer membranes (the periplasm) and surround these components. The stator uses ions to generate the energy that makes the rotor whirl. In turn, this movement sets the filament in motion, propelling the bacterium. Depending on where the bacteria live, the stator can use different types of ions. In addition, while many species have a single stator system per motor, some may have several stator systems for one motor: this may help the microorganisms move in different conditions.
As microbes colonize environments with a different pH or viscosity, they constantly evolve new versions of the motor which are more suitable to their new surroundings. However, a part of the motor remains the same across species. Overall, it is still unclear how bacterial flagella evolve, but examining the structure of new motors can shed light upon this process. Here, Kaplan et al. combine a bioinformatics approach with an imaging technique known as electron cryo-tomography to dissect the structure of the flagellar motor of three species of bacteria with different stator systems, and compare these to known motors of the same class.
The results reveal a correlation between the nature of the stator system and the presence of certain elements. Stators that use sodium ions, or both sodium and hydrogen ions, are associated with two periplasmic rings surrounding the conserved motor structure. These rings do not exist in motors with single hydrogen-driven stators. Motors with dual hydrogen-driven stators are, to some extent, an ‘intermediate state’, with only one of those rings present. As all the studied species currently exist, it is difficult to know which version of the motor is the most ancient, and which one has evolved more recently.
Capturing the diversity of bacterial motors gives us insight into the evolutionary forces that shape complex molecular structures, which is essential to understand how life evolved on Earth. More practically, this knowledge may also help us design better nanomachines to power microscopic robots.