Multiple interfaces between a serine recombinase and an enhancer control site-specific DNA inversion
Figures
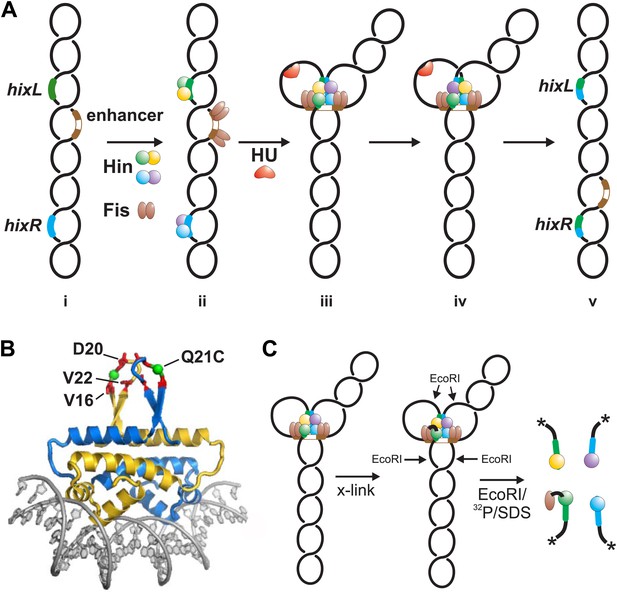
Hin-catalyzed DNA inversion reaction and outline of Fis-Hin crosslinking experiments.
(A) The Hin reaction pathway proceeds by Hin dimers binding to hixL and hixR and two Fis dimers binding to the enhancer element (brown) (ii). The DNA bending protein HU aids in formation of the small (∼100 bp) loop during assembly of the invertasome (iii). Each of the four Hin subunits cleaves the hix DNA by forming phosphoserine linkages with the 5′ ends, and the DNA strands are exchanged by rotation of the purple and yellow subunits relative to the green and blue subunits (iv). The DNA is ligated by Hin in the recombinant orientation (v). (B) Fis-DNA crystal structure (PDB ID: 3IV5) highlighting the sole cysteine at the crosslinking target residue 21 (green spheres) in the protruding β-hairpin arms where surrounding residues that contact Hin are shown as red sticks. (C) Crosslinking is performed after incubating Fis and Hin under conditions stabilizing DNA-cleaved invertasomes. pRJ2372 (Figure 1—figure supplement 1) contains EcoRI sites flanking the hix sites such that each Hin subunit can be 32P-labeled by DNA polymerase and radiolabeled dNTPs after digestion with EcoR1, and the crosslinked Fis-Hin-(32P)DNA product is then detected by SDS-PAGE.
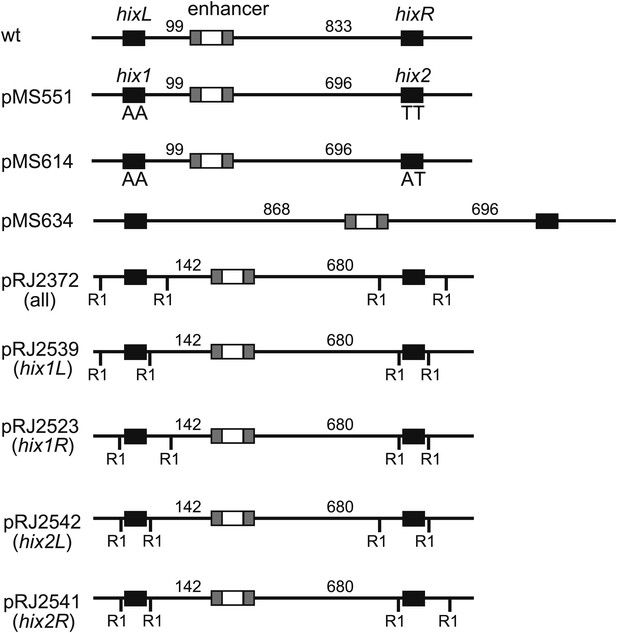
Plasmid substrate design.
Wild type (wt) shows the spacing and layout of the elements in the invertible segment on the Salmonella enterica serovar Typhimurium chromosome. All plasmids used for Hin reactions in this work contain two copies of the Hin recombination site hixL called hix1 and hix2. For crosslinking substrates, EcoRI sites are shown as RI and are located either 14 bp or 50 bp from the position of Hin cleavage at the center of the hix site. pMS551, pMS614, and pMS634 are from Johnson and Simon (1985), and pRJ2372 is from Dhar et al. (2009a).
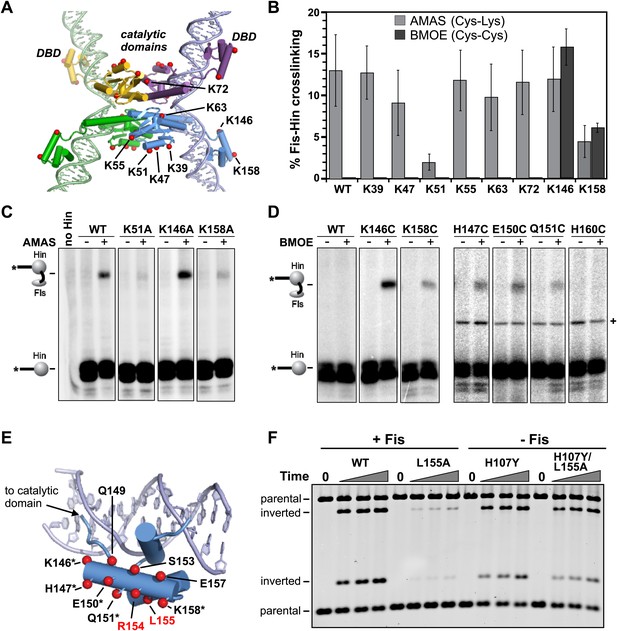
Identification of the Fis contact region on the Hin DBD.
(A) DNA-cleaved tetramer model of Hin with the locations of eight lysines on each subunit shown as red spheres. (B) Fis-Hin AMAS or BMOE crosslinking efficiencies (mean and standard deviation from ≥three experiments) between Fis-Q21C and eight lysine to alanine (AMAS, 4.4 Å spacer, gray bars) or cysteine (BMOE, 8 Å spacer, dark bars) Hin mutants. Percent Fis-Hin crosslinks are relative to the Hin-(32P)DNA cleavage product. (C and D) Representative phosphorimages of AMAS or BMOE crosslinking experiments, respectively. A Hin- and crosslinker-independent contaminant band, which sometimes originates from an incompletely digested DNA fragment, is marked with a (+). (E) Hin DBD—hixL crystal structure (PDB ID: 1IJW) with residues evaluated for crosslinking or Fis-activated DNA inversion (Table 1) shown as spheres (Cβ atoms). Asterisks signify residues exhibiting BMOE crosslinking with Fis-Q21C when replaced with cysteine; red highlights the two key residues proposed to directly contact Fis. (F) DNA inversion activity of Hin-L155A in the absence or presence of the H107Y hyperactivating mutation enabling Fis/enhancer-independent inversion. Hin was added to reactions containing pMS551, HU, and Fis, as designated, and aliquots were taken at 0, 1, 2, and 5 min. Digestion with HindIII and PstI allows the inverted and parental DNA orientations to be distinguished. Hin-wt does not generate detectable inversions under these no-Fis conditions.
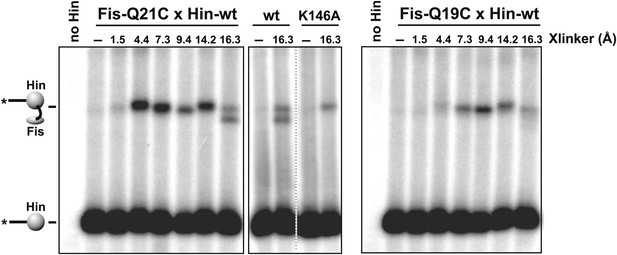
Heterobifunctional crosslinking using different length crosslinkers targeting either Cys21 or Cys19 on Fis and a primary amine on Hin.
Fis-Q21C exhibits efficient crosslinking with Hin beginning at 4.4 Å, whereas Fis-Q19C exhibits less efficient crosslinking beginning at 7.3 Å. The additional product at 16.3 Å using Fis-Q21C is absent with Hin-K146A, suggesting this product represents a crosslink between these two residues. Fis-wt and Fis-R71C (cysteine near DNA binding domain) do not generate Hin crosslinks (Dhar et al., 2009a). Crosslinkers are: SIA (1.5 Å), AMAS (4.4 Å), GMBS (7.3 Å), EMCS (9.4 Å), SMPH (14.2 Å), and KMUS (16.3 Å) (Pierce-Thermo Scientific).
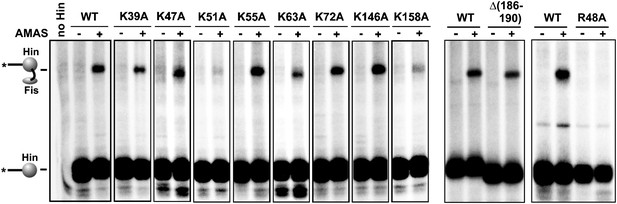
Complete set of AMAS crosslinking data between Cys21 on Fis and a primary amine on Hin.
Hin-wt and the eight lysine to alanine mutants are from one experiment and the C-terminal deletion (Δ(186–190), which includes Lys186 and Lys187,) and Hin-R48A are from separate experiments.
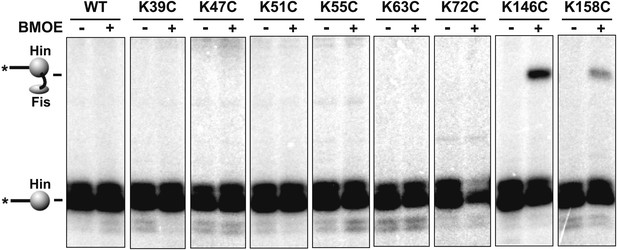
BMOE crosslinking data between Cys21 on Fis and cysteines introduced at eight lysine residues in Hin.
Hin-wt contains a single cysteine at residue 28, which does not crosslink with Fis-Q21C (left panel).
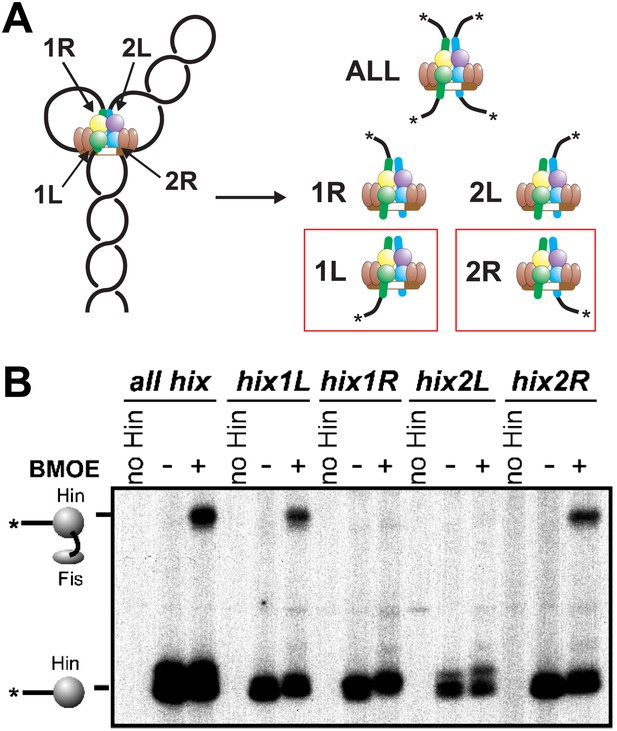
Fis contacts the two Hin subunits bound to half-sites hix1L and hix2R that are positioned at the base of the invertasome.
(A) Plasmid substrates used to determine which Hin subunits contact Fis (see also Figure 1—figure supplement 1). The 3′ DNA ends from each of the four Hin-DNA covalent complexes are labeled using the standard substrate pRJ2372 upon digestion with EcoRI and DNA polymerase fill-in (all hix). Four additional substrates were employed in which the DNA from only one Hin-DNA cleavage complex can be labeled due to the locations of the EcoR1 sites. DNA-labeled crosslinks between Fis-Q21C and the Hin protomers bound to the left half-site of hix1 (hix1L) and the right half-site of hix2 (hix2R) are observed (red outlines). (B) Fis-Hin crosslinking between Fis-Q21C and Hin-K146C on the different DNA substrates using BMOE.
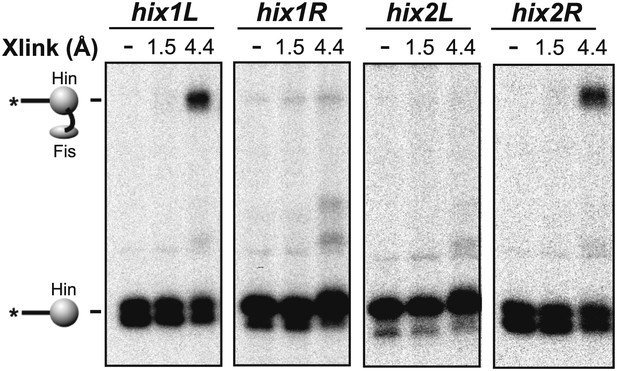
Heterobifunctional crosslinking (cysteine-lysine) between Fis-Q21C and Hin-wt was performed using N-succinimidyl iodoacetate (1.5 Å, no crosslinks obtained) or AMAS (4.4 Å).
As observed with the BMOE (cysteine-cysteine) crosslinking, Fis-Hin crosslinks by AMAS only occurred with Hin subunits bound to hix1L and hix2R.
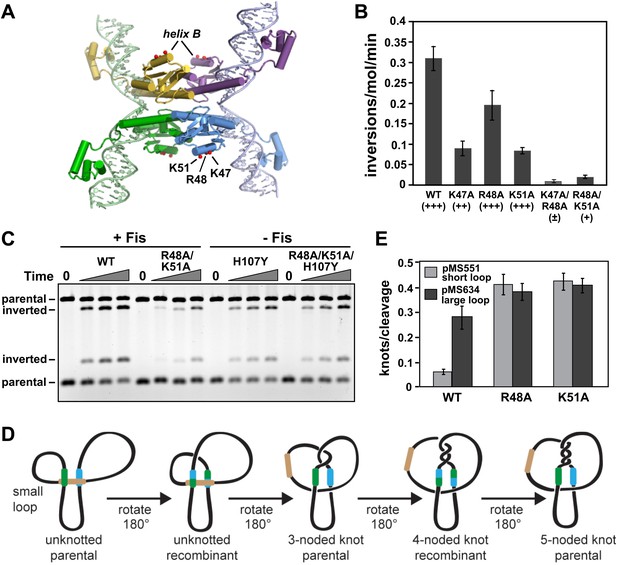
Residues within Hin helix-B function in Fis/enhancer-dependent Hin activation and control of subunit rotation.
(A) DNA-cleaved Hin tetramer model with the locations of basic residues in the helix-B region highlighted with red spheres. (B) Fis-activated DNA inversion rates of helix-B Hin mutants reported as inversions/molecule/minute (mean and standard deviation from ≥three experiments). In vivo rates are given in parentheses; see Table 1 for legend. (C) DNA inversion kinetics of a Hin helix-B double mutant. Inversion reactions were performed in the presence and absence of Fis for 0, 1, 2, and 5 min. Hin-R48A/K51A exhibits 10-fold slower kinetics than Hin-wt under Fis-activating conditions, but is fully competent for Fis-independent inversion when coupled to the hyperactivating mutation Hin-H107Y. (D) Schematic representation of topological changes during Hin recombination. Normally Hin ligates the DNA after a single DNA exchange by subunit rotation due to the small loop between the hix site and the enhancer, resulting in an unknotted inverted product. If the loop is large or the enhancer is released (as shown), multiple rounds of subunit rotations can occur resulting in DNA knots with increasing numbers of nodes. (E) Quantitation of DNA knotted forms relative to the amount of initially Hin-cleaved plasmid (mean and standard deviation from ≥three experiments) from single-round knotting experiments (see Figure 4—figure supplement 3). Hin-wt efficiently forms knots only on pMS634 containing the long spacer, whereas Hin-R48A and K51A are insensitive to spacer length, reflecting an unstable interaction with the enhancer.
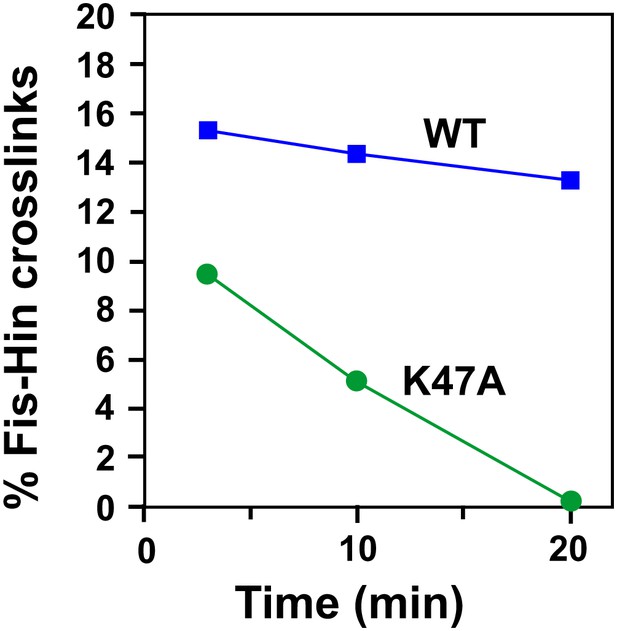
Hin helix-B mutation K47A destabilizes association of the Fis/enhancer with the active Hin tetramer.
Invertasomes were assembled with Fis-Q21C and Hin-wt or Hin-K47A in Mg2+-free ethylene glycol buffer. At 2.5, 10, and 20 min after addition of proteins, the reaction was subjected to AMAS crosslinking for 30 s. Fis-Hin crosslinked products were quantified relative to the total Hin-(32P-DNA) products at that time point and plotted with respect to time of incubation. Note that the percent of Hin-K47A—Fis crosslinked products relative to Hin-cleaved DNA are relatively high initially but decrease with time, whereas Hin-wt—Fis crosslinks remain high over the 20 min time period.
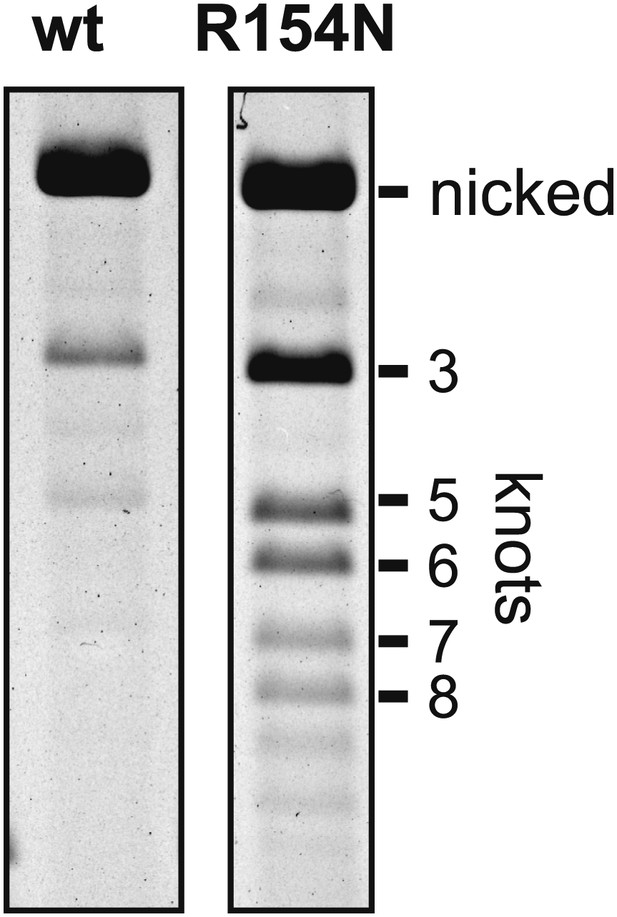
Partial disruption of Fis-Hin interactions leads to DNA knotting by processive subunit rotations.
Fis, HU, and Hin-wt or Hin-R154N were incubated for 10 min under inversion conditions with pMS614, which contains AT dinucleotides at the hix2 crossover region that prevents ligation in the recombinant orientation (Figure 1—figure supplement 1; Merickel and Johnson, 2004). The reaction was quenched, ethanol precipitated, digested with the nicking enzyme Nt.BsmAI, and the product topologies were resolved on an agarose gel. Hin R154N generates 50% knotted products whereas Hin-wt generates 2%, despite a twofold decrease in inversion rate by Hin-R154N.
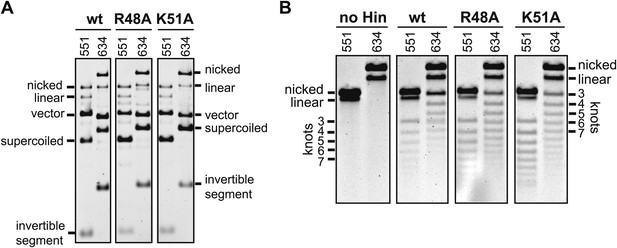
Primary data for single round knotting experiments summarized in Figure 4E.
(A) pMS551 or pMS634, which have 99 bp or 696 bp between the enhancer and closest hix site, respectively, were incubated with Hin, Fis, and HU for 5 min under ethylene glycol Mg2+-free cleavage conditions, and an aliquot was quenched and electrophoresed in an agarose gel to assess the amount of DNA cleavage. Vector and invertible segment bands represent double strand cleavages at both hix sites. (B) The remainder of the reaction from panel A was diluted with buffer containing no ethylene glycol and 10 mM Mg2+ to enable ligation. The DNA was then nicked and electrophoresed in an agarose gel to resolve knotted forms. The identities of the bands, including the number of nodes in each knotted form for pMS551 and pMS634 are shown on the left and right, respectively. The percent of knotted ligation products per Hin-cleaved substrate is given in Figure 4E.
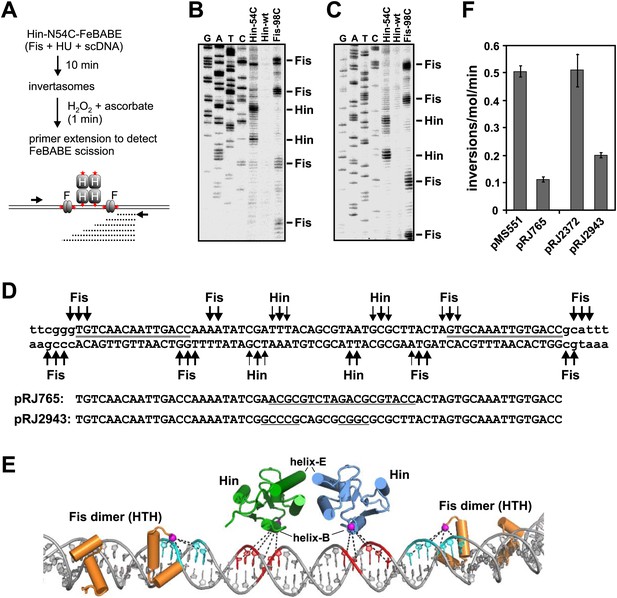
Localization of proteins on the hin enhancer DNA by site-specifically tethered FeBABE-mediated DNA scission.
(A) Experimental approach for mapping enhancer DNA contacts by the Hin helix-B region. Red stars denote sites of FeBABE coupling. (B and C) Sequencing gels resolving primer extension products of DNA scission by FeBABE coupled to Hin-N54C, Hin-wt (control), and Fis-N98C next to dideoxy sequencing reactions. Top strand primer in B; bottom strand primer in C. (D) Sequence of enhancer DNA with primary Fis-N98C-FeBABE and Hin-N54C-FeBABE scission sites denoted. Thickness of arrows approximates scission efficiency based on several gels. Gray lines designate the 15 bp core Fis binding sites. Below are the sequences of two mutant enhancers used in panel F; underlined sequences are changes from the wild type. (E) Molecular model of enhancer segment within the invertasome structure (‘Discussion’, ‘Material and methods’, and Figure 7C). Shown are the helix-turn-helix regions of the Fis dimers (orange) and the catalytic domains of the two enhancer-proximal Hin subunits (green and blue). The Hin domains are rotated 40° about the y-axis relative to Figure 2A. Residues 98 on Fis and 54 on Hin have been replaced with cysteine and the Sγ atoms where FeBABE (12 Å to the Fe that generates hydroxyl radicals) is coupled are highlighted by magenta spheres. DNA scission sites generated by Hin-N54C-FeBABE are in red and by Fis-N98C-FeBABE are in cyan. (F) Inversion rates on mutant enhancers contained on pRJ765 and pRJ2943 relative to their wild-type enhancer parent substrates pMS551 and pRJ2372, respectively (Figure 1—figure supplement 1). Inversion rates are reduced by the mutations but are much greater than no-Fis reactions (<0.001 inversions/molecule/min).
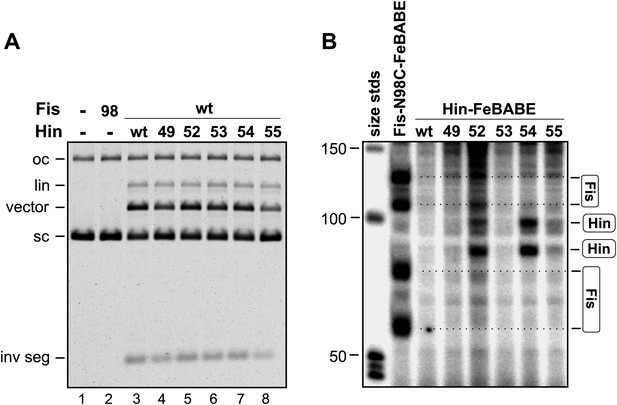
Activities and scission over the enhancer region by Hin mutants coupled to FeBABE.
(A) Invertasome were assembled on pRJ2372 with Hin-wt (lane 3) or Hin mutants containing cysteine substitutions at the designated positions (lanes 4–8) that had been subjected to FeBABE coupling. Formation of DNA-cleaved invertasomes was measured by addition of SDS after a 10 min incubation and electrophoresis on an agarose gel after digestion with proteinase K. Lane 1 is the plasmid control, and lane 2 is incubation with Fis-N98C that had been coupled with FeBABE. Hin-catalyzed DNA cleavage generates the vector backbone and the invertible segment (inv seg). (B) Na ascorbate and H2O2 were added to the reactions in panel A to initiate DNA scission by FeBABE for 30 s. Scission over the enhancer segment was probed by primer extension using a 32P-labeled oligonucleotide that hybridizes 66 nts downstream from the closest Fis binding site (see Figure 5A), and the products were electrophoresed on an 8% denaturing polyacrylamide-urea gel. Size standards (nts) are on the left. High resolution (sequencing) gels of the scission products by Hin-N54C-FeBABE and Fis-N98C-FeBABE are shown in Figures 5B,C.
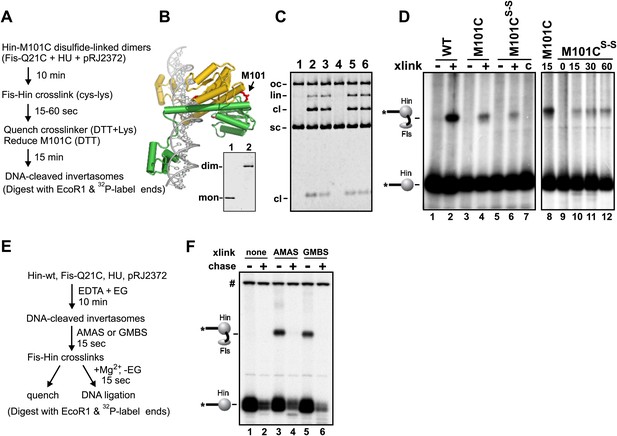
Fis-Hin connections at early and late steps in the DNA inversion reaction.
(A–D) Hin dimers covalently linked to Fis proceed to tetramers active for DNA cleavage. (A) Outline of the experiment. (B) Hin dimer model highlighting Met101. Hin-M101C forms disulfide-linked dimers; insert shows non-reducing SDS-PAGE of reduced Hin-M101C (lane 1) and purified disulfide-linked dimeric Hin-M101C (lane 2). (C) Hin cleavage reactions with Fis-Q21C (10 min) displayed on an agarose gel. Lane 1, unreacted DNA; lane 2, Hin-wt reaction; lane 3, reduced Hin-M101C reaction; lane 4, disulfide-linked Hin-M101Cs–s reaction; lane 5, disulfide-linked Hin-M101Cs–s reaction then incubated 10 min with 10 mM DTT; lane 6, disulfide-linked Hin-M101Cs–s reaction, crosslinked with GMBS (7.3 Å spacer), then incubated 10 min with 10 mM DTT, which inactivates the crosslinker and reduces the disulfide bond. (D) Fis-Hin crosslinking products displayed on an SDS gel. Lanes 1–6 are Hin-wt and reduced or disulfide-linked M101C crosslinked with Fis-Q21C for 30 s with GMBS as designated (+), the crosslinker was quenched, and the reaction incubated an additional 10 min under reducing conditions to form DNA-cleaved invertasomes. The presence of a Fis-Hin-(32P)DNA crosslinked product in lane 6, as well as in lanes 10–12 where crosslinking times (s) were varied, demonstrates that covalently crosslinked Fis-Hin dimers can transition into Hin tetramers competent to cleave DNA. In lane 7, the DTT + lysine quench was added immediately prior to the crosslinker, demonstrating that the crosslinking in lanes 6 and 10–12 could not have occurred after reduction of the dimer. (E and F) DNA ligation by Hin proceeds when Hin is covalently crosslinked to Fis. (E) Outline of the experiment. (F) Control and crosslinked DNA cleavage reactions were either quenched directly (−) or chased by addition of 10 mM Mg2+ and dilution of the ethylene glycol to induce DNA ligation. The amount of cleaved DNA remaining after switching to ligation conditions is assessed by the levels of Fis-Hin-(32P)DNA or Hin-(32P)DNA complex. Over 85% of the Hin-DNA and >95% of the Fis-crosslinked Hin-DNA covalent product is lost, demonstrating Fis-Hin association does not inhibit DNA ligation. The band designated # is a labeled DNA fragment from the substrate.
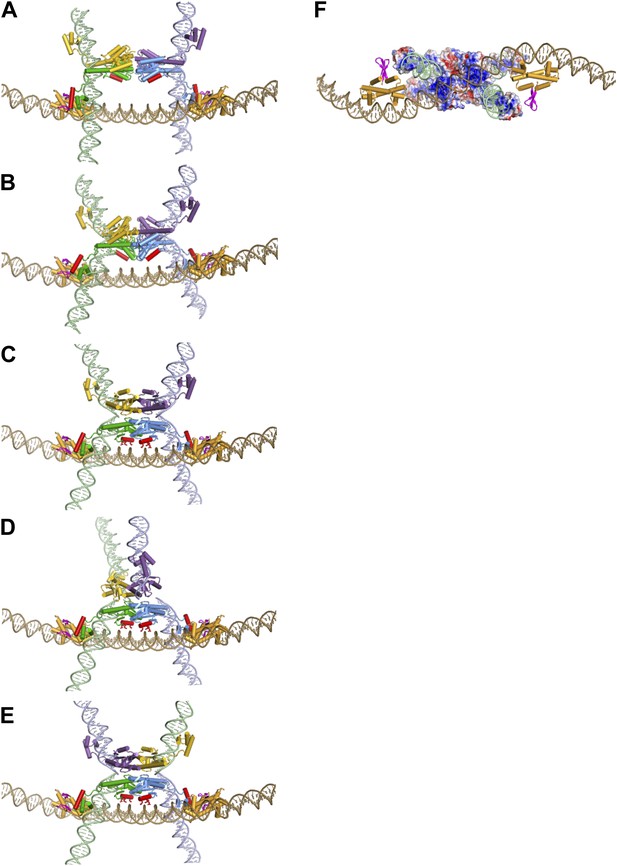
Assembly of the Hin invertasome.
(A) Two Hin dimers bound to hix sites docked onto the enhancer (brown) with helix-B of the catalytic domain and helix-1 of the DBD highlighted in red. Fis dimers are gold with their mobile β-hairpin arms colored magenta. The hix DNA segments cross the enhancer to form 2 (−) nodes, consistent with a branch on negatively supercoiled DNA. (B) The pre-cleaved Hin tetramer model docked onto the enhancer. (C) DNA-cleaved Hin tetramer model docked onto the enhancer. In this conformation, basic residues within helix-B of the Hin catalytic domain (Lys47, Arg48, and Lys51, displayed as sticks) are close to the enhancer DNA, and the flat interface enabling subunit rotation has formed. (D) Rotation (50°) of the yellow and purple subunits relative to the green and blue subunits that remain bound to the Fis/enhancer element. (E) Complete subunit exchange positions the cleaved DNA ends into the recombinant configuration. See Videos 1 and 2. (F) DNA-cleaved Hin tetramer model rotated 90° about the x-axis relative to panel C with the electrostatic surface potential (± 4 kT e−1) displayed. Two distinct basic regions (blue) surrounding helix-B on the bottom two Hin subunits are positioned adjacent to the enhancer DNA.
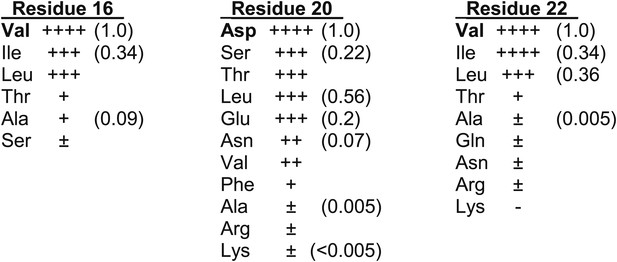
Chemical properties of Fis residues proposed to contact the Hin DBD.
A previous cysteine scan of residues throughout the β-hairpin arm of Fis identified the prime importance of Val16, Asp20, and Val22 located near the tip (see Figure 1B) for activation of Hin-catalyzed DNA inversion (Safo et al., 1997). The results of further mutagenesis of these residues are presented here. Fis mutants were evaluated for activation of Hin-catalyzed DNA inversion in vivo using the same lacZ reporter system as utilized for the Hin mutants, except that the Fis mutant gene on a plasmid was introduced into the cell containing Hin-wt (see Safo et al., 1997 for experimental details). For each residue the order of amino acid residues (top to bottom) reflects their relative in vivo activities. (++++) indicates wild-type activity where red colonies developed on lactose MacConkey agar plates within 27 hr. (+++) indicates red colonies developed between 28–30 hr; (++) indicates red colonies between 32–34 hr, (+) indicates red colonies after 36–38 hr, (±) indicates evidence of very low inversion after 40 hr, (−) indicates no detectable inversion after 48 hr like the no Fis control. Selected Fis mutants were partially purified and assayed for activation of Hin-catalyzed inversion in vitro. Numbers in parentheses reflect inversion rates relative to Fis-wt (set to 1.0). The data indicate that Fis residues 16 and 22 are most active when hydrophobic (Val, Ile, Leu). Polar substitutions (Ser, Thr, Glu) at Asp20 exhibit moderate activity, and a leucine substitution has surprisingly high activity. These Fis residues are proposed to specifically interact with Hin DBD residues Arg154, Leu155, and possibly Gln151 during invertasome assembly.
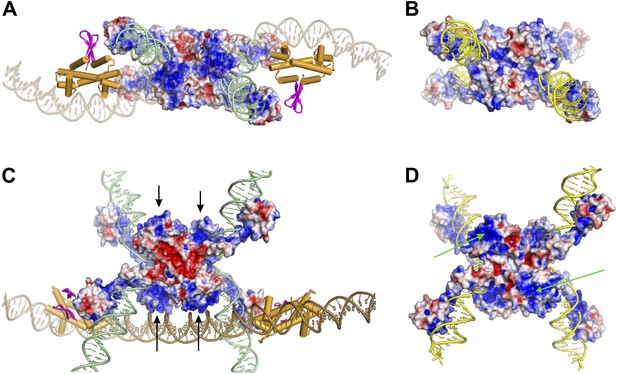
Electrostatic surface potentials of Hin and γδ resolvase (± 4.0 kT e−1 for all images).
(A) Hin invertasome model in a view similar to Figure 7F, except that the enhancer DNA is partially transparent to more easily visualize the basic regions (blue) surrounding the B-helices. (B) γδ resolvase tetramer (PDB ID: 1ZR4) in a similar orientation as the Hin tetramer in panel A. Note the absence of the prominent basic patches that are present in Hin. (C) View of the Hin invertasome in a similar orientation as in Figure 7C. Arrows denote the basic regions surrounding the B-helices. (D) γδ resolvase tetramer in a similar orientation as the Hin tetramer in panel C. Arrows point to a basic region containing resolvase residues Lys29, Arg32, and Lys54; in wild-type resolvase Arg2 would also be located within the basic patch. Arg2, Arg32, Lys54, and Glu56 comprise the 2–3′ crystallographic interface between dimers that is important for assembly of the active synaptic complex (Hughes et al., 1990; Murley and Grindley, 1998; Burke et al., 2004; Olorunniji et al., 2008). The Sin resolvase regulatory residues F52 and R54, which also participate in a crystallographic dimer-dimer interface, are located within this region as well (Mouw et al., 2008).
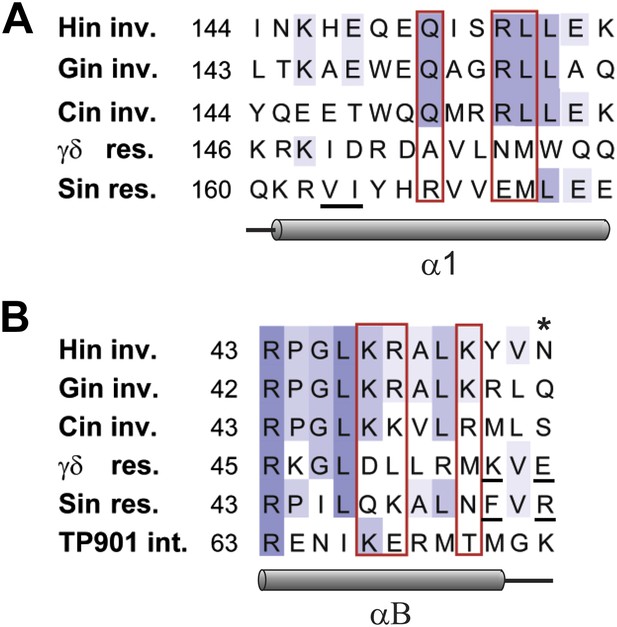
Sequence alignments of select serine recombinases over the regions that Hin contacts the Fis/enhancer.
(A) Sequence alignment over the DBD helix-1 region of members of the Fis/enhancer-dependent DNA invertase family (Hin, Gin, Cin) and Fis/enhancer-independent resolvase family (γδ, Sin). Secondary structure is from Hin (PDB ID: 1IJW); the lengths of helix-1 in resolvases vary. Solvent-exposed residues Gln151, Arg154, and Leu155, which are proposed to directly or indirectly interact with Fis, are uniquely conserved among DNA invertases. Sin residues Val163 and Ile164 (underlined) mediate synapsis of regulatory subunits during formation of the Sin synaptic complex (Mouw et al., 2008). (B) Sequence alignment over the helix-B region of DNA invertases (Hin, Gin, Cin), resolvases (γδ, Sin), and a large serine recombinase (TP901 integrase). Secondary structure is from the Hin model; the helix-B boundaries are similar in most resolvase and Sin structures. Hin residue Asn54, the site of FeBABE coupling, is marked with an asterisk. The basic character of Hin residues 47, 48, and 51 are uniquely conserved among DNA invertases. Mutations at the conserved residue Arg43, which functions in folding or catalysis in other serine recombinases (Olorunniji and Stark, 2009; Keenholz et al., 2011), also inactivate Hin. γδ resolvase residues Lys54 and Glu56 and Sin residues Phe52 and Arg54 (underlined) participate in protein-protein interactions between regulatory and catalytic subunits in these resolution reactions (Hughes et al., 1990; Murley and Grindley, 1998; Burke et al., 2004; Mouw et al., 2008; Olorunniji et al., 2008; Rowland et al., 2009).
Videos
Different views of the Hin invertasome model shown in Figure 7C.
Assembly of the Hin invertasome and DNA exchange by subunit rotation within the invertasome structure (Figure 7A–E).
Tables
Fis-activated DNA inversion activities of Hin DBD helix 1 mutants
https://doi.org/10.7554/eLife.01211.009Mutant | DNA inversion in vivo* | DNA inversion in vitro† |
---|---|---|
WT | +++ | 0.31 ± 0.02 |
WT (−Fis) | – | <0.001 |
K146A | +++ | 0.30 ± 0.03 |
H147A | +++ | 0.38 ± 0.02 |
E148A | +++ | 0.19 ± 0.03 |
Q149A | +++ | 0.37 ± 0.04 |
E150A | +++ | 0.30 ± 0.05 |
Q151A | ++ | 0.08 ± 0.01 |
S153A | +++ | 0.17 ± 0.01 |
R154A | + | 0.08 ± 0.02 |
L155A | ± | <0.005 |
E157A | +++ | 0.29 ± 0.03 |
K158A | +++ | 0.17 ± 0.02 |
R154S | + | 0.13 ± 0.03 |
R154N | ++ | 0.17 ± 0.02 |
R154C | + | 0.06 ± 0.01 |
R154D | + | 0.02 ± 0.01 |
L155V | ± | 0.03 ± 0.01 |
L155G | ± | <0.01 |
L155S | ± | <0.02 |
L155T | ± | <0.01 |
L155K | ± | <0.01 |
L155R | ± | <0.02 |
-
*
In vivo DNA inversion rates as measured by color development on lactose MacConkey media. +++ indicates red colonies developed within 24 hr, ++ red colonies between 25–29 hr, + red colonies between 30–34 hr, ± some red or papillations after 36 hr, and -no evidence of inversion (red) after 48 hr, as observed for no Hin or no Fis experiments.
-
†
In vitro recombination rates (DNA inversions/molecule/minute) obtained with purified proteins (mean and standard deviation from at least three determinations).