APOBEC3A deaminates transiently exposed single-strand DNA during LINE-1 retrotransposition
Figures
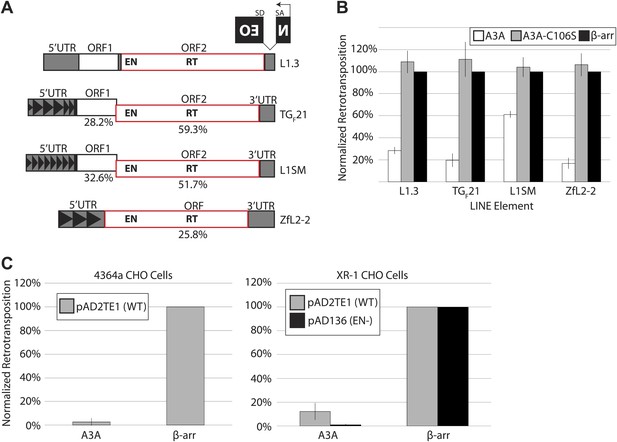
A3A suppresses retrotransposition of distinct LINEs, but does not specifically inhibit L1 endonuclease activity.
(A) LINE schematics: L1.3, TGF21, and L1SM encode two ORFs (ORF1 and ORF2, outlined in black and red, respectively). ZfL2-2 contains one ORF (outlined in red). Indicated are the endonuclease (EN) and reverse transcriptase (RT) domains in the respective ORFs. All elements are tagged in their 3’UTRs with the mneoI (NEO) indicator cassette. The percent nucleotide identity of TGF21, L1SM, and ZfL2-2 to human L1.3 is indicated below each schematic. Black arrowheads indicate repeated monomeric sequences in the 5′ UTRs of TGF21, L1SM, and ZfL2-2. (B) A3A inhibits retrotransposition of distinct LINEs: shown is the effect of wild-type A3A (white bars), deaminase-deficient A3A_C106S (gray bars), and β-arrestin (β-arr) control (black bars) on LINE retrotransposition. The x-axis indicates the LINE element. The y-axis indicates percent retrotransposition; in each case the β-arr control is set to 100%. Data were normalized using a circular NEO expression cassette as detailed in Figure 1—figure supplement 1A. Data are expressed as the mean percent retrotransposition derived from three independent experiments consisting of two technical replicates each, with error bars representing the standard deviation among all six technical replicates. (C) Retrotransposition assays in 4364a (wild-type CHO cells (left)) and XR-1 (XRCC4-deficient CHO cells (right)): the x-axis indicates experiments conducted with the A3A or β-arr expression vector. The y-axis indicates percent retrotransposition; for each reaction the β-arr control is set to 100%. Gray bars indicate retrotransposition of wild-type L1 (pAD2TE1) and black bars indicate the L1 EN mutant (pAD136). Data were normalized using the circular NEO control (Figure 1—figure supplement 1A). Data are shown as the mean percent retrotransposition derived from three independent experiments consisting of two or three technical replicates each, with error bars representing the standard deviation among all eight technical replicates.
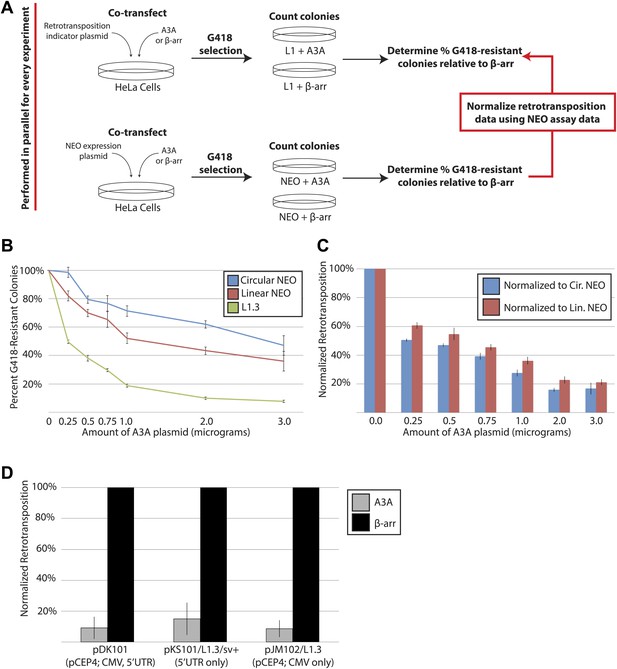
Additional Control Experiments.
(A) Strategy for A3A toxicity controls: L1 retrotransposition assays (top schematic: an engineered L1 plasmid co-transfected with either an A3A or β-arrestin expression vector) were conducted in parallel with toxicity control assays (bottom schematic: a linear or circular NEO expression vector co-transfected with either an A3A or β-arrestin expression vector). The percentages of G418-resistant colonies relative to the β-arrestin control were determined in both experiments. The results from the toxicity control experiments were used to normalize data from the corresponding L1 retrotransposition assays. (B) A3A expression inhibits L1 retrotransposition despite exhibiting some cytotoxicity: HeLa cells were transfected with 1 μg of pJM101/L1.3 or pU6i NEO expression vector, and increasing amounts of A3A expression vector (from 0.0 μg to 3.0 μg). The remaining plasmid mass included the β-arrestin expression vector. Thus, each co-transfection consisted of a total plasmid mass of 4 μg. The x-axis indicates the amount of A3A plasmid present in the co-transfection. The y-axis indicates percent of G418-resistant colonies. Shown are experiments conducted with a circular NEO expression vector (blue line), a linearized NEO plasmid (red line), or pJM101/L1.3 (green line). Data are expressed as the mean percent G418-resistant colonies derived from two independent experiments consisting of two technical replicates each, with error bars representing the standard deviation among all four technical replicates. (C) Normalization controls: the colony counts generated in the retrotransposition assay (Figure 1—figure supplement 1B) were normalized using the colony counts generated in the linear or circular NEO control toxicity experiments. The x-axis indicates the amount of A3A plasmid in the co-transfection. The y-axis indicates normalized retrotransposition values using the data with the circular NEO expression vector (blue bars) or the linearized NEO plasmid (red bars). Data are expressed as the mean percent retrotransposition derived from two independent experiments consisting of two technical replicates each, with error bars representing the standard deviation among all four technical replicates. (D) A3A inhibits the retrotransposition of human LINE-1 elements expressed from various contexts: HeLa cells were co-transfected with 0.5 μg of an A3A expression vector and 0.5 μg of the indicated L1 expression vector per well of a six-well tissue culture plate. The x-axis indicates the L1 expression vector. In pDK101, a CMV promoter augments L1.3 expression from the pCEP4 backbone. In pKS101/L1.3.sv+, the native L1 5′UTR drives transcription from a pBluescript (Stratagene) backbone. In pJM102/L1.3, the CMV promoter drives L1 expression from the pCEP4 episomal backbone; the construct lacks the L1.3 5′UTR. The y-axis indicates the normalized percent of retrotransposition in the presence of a β-arrestin (black bars) or A3A (gray bars) expression plasmid. Data were normalized relative to the linear NEO control. Data are expressed as the mean percent retrotransposition derived from two independent experiments consisting of three technical replicates each, with error bars representing the standard deviation among all six technical replicates.
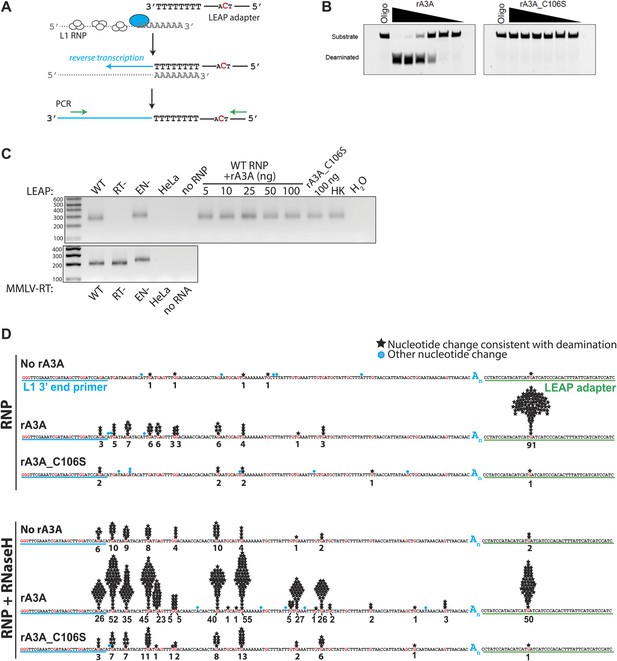
Recombinant A3A (rA3A) deaminates L1 cDNAs in vitro.
(A) LEAP assay rationale: L1 RNP preparations consisting of the L1 RNA (gray), L1 ORF1p (white ovals), and L1 ORF2p (blue oval) are incubated with a 3′ RACE primer consisting of a unique adapter sequence that contains a single cytidine (red) followed by an oligo dT sequence (black lettering). After reverse transcription (blue arrow), the resultant L1 cDNAs (blue line) are PCR amplified using primers specific to the engineered L1 and the unique adapter sequence (green arrows). (B) Recombinant A3A has deaminase activity in vitro: twofold serial dilutions (500 ng–15.62 ng) of WT rA3A (left panel) or deaminase-deficient rA3A_C106S (right panel) were incubated with a fluorescein isothiocyanate (FITC) labeled single-strand DNA oligonucleotide containing a single cytidine residue. The products were treated with recombinant uracil DNA glycosylase (UDG) and NaOH and then were resolved by gel electrophoresis. A control reaction was included without recombinant protein (marked Oligo). (C) Recombinant A3A does not inhibit L1 RT activity: control LEAP reactions with RNP preparations from HeLa cells transfected with WT (pDK101), RT- (pDK135), or EN- (pJJH230A/L1.3) human L1s (upper gel). HeLa indicates untransfected HeLa cells; no RNP indicates control reactions lacking RNPs. Increasing amounts of rA3A (ng) did not significantly affect LEAP activity. Samples containing a deaminase-deficient rA3A_C106S, a heat killed rA3A (HK), or without LEAP products (H2O) served as controls. MMLV RT reactions (lower gel) confirm the integrity of purified RNA isolated from RNP preps used in the LEAP assay. Notably, the increased size of the EN- RNP RT products is due to a higher molecular weight product generated from pJJH230A/L1.3, which contains an mblastI indicator cassette instead of an mneoI indicator cassette. Size standards (bp) are indicated at the left of the gel. (D) Sequence characterization of LEAP Products: shown is the (+) strand sequence of the LEAP product. Guanosine nucleotides are indicated in red. Black stars and numbers indicate the frequency of G-to-A mutations (corresponding to C-to-U mutations in the minus (−) strand L1 cDNA) that occurred on (+) strand L1 cDNA. Blue circles indicate other nucleotide changes. The blue An indicates the LEAP product poly (A) tail. Blue underlining indicates the L1 3′ end PCR primer. Green underlining indicates the LEAP adapter (5np1) sequence. Top panel: LEAP products generated under the following conditions: no rA3A protein, 100 ng of wild-type rA3A, 100 ng of deaminase-deficient rA3A_C106S. Bottom panel: LEAP products generated under the following conditions in the presence of RNase H: no rA3A protein, 100 ng of wild-type rA3A, 100 ng of deaminase-deficient rA3A_C106S. One hundred products were characterized for each condition.
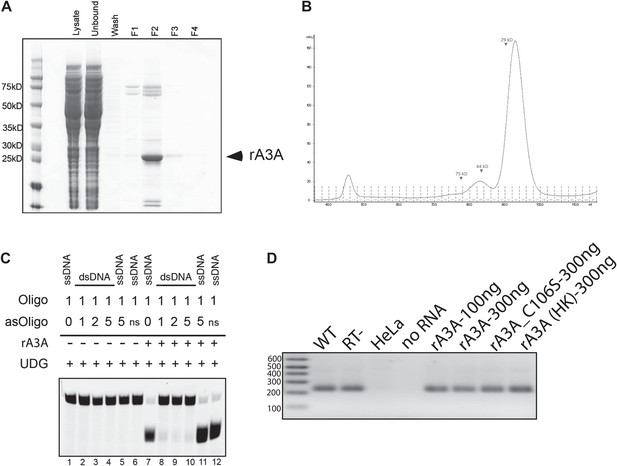
Control Experiments with Recombinant A3A.
(A) Purification of rA3A from E. coli by Ni affinity: SDS 4–12% polyacrylamide gel electrophoresis and Coomassie blue staining were used to monitor purification of the His-tagged recombinant A3A (rA3A) protein. Input bacterial lysate was loaded onto the Ni-Sepharose column (Lysate). Flow through lysate and wash fractions were collected (Unbound and Wash, respectively). Recombinant A3A was eluted from the column with lysis buffer containing 0.5M imidazole and consecutive elution fractions were collected (F1-4). Most of the His-tagged rA3A was eluted in F2 (arrow). Approximate molecular sizes (kD) are indicated at the left of the gel. (B) Purification of rA3A by gel filtration: recombinant A3A purified by Ni-Affinity was further purified by gel filtration by fast protein liquid chromatography (FPLC) on a Superdex 200 column. Arrows indicate the approximate molecular weights (kD) of the proteins. The x-axis indicates the elution volumes and fraction numbers. The y-axis indicates the UV absorbance at 280 nm. (C) Recombinant A3A does not deaminate double-stranded DNA: single-strand (lanes 1 and 7) or double-stranded DNA (lanes 2–4 and 8–10) substrates were incubated without (−) or with (+) rA3A (250 ng), were treated with UDG, and the products were resolved by gel electrophoresis on 15% polyacrylamide TBE-Urea Novex gels (Invitrogen). The relative ratios of the target (Oligo) and complementary (asOligo) oligonucleotides are indicated at the top of the figure. A non-specific oligonucleotide (ns) was also included as a control (lanes 6 and 12). As an additional control to rule out potential competition of free asOligo for UDG activity, asOligo was added after rA3A incubation with ssDNA Oligo (lanes 5 and 11). (D) Recombinant A3A does not inhibit MMLV-RT activity: from left to right: MMLV RT reactions using purified RNA isolated from pDK101 (WT) or pDK135 (RT-) RNPs, untransfected HeLa cell RNPs (HeLa), and a no RNA sample. Recombinant WT rA3A (100 ng and 300 ng), deaminase-deficient rA3A_C106S (300 ng), and ‘heat-killed’ rA3A (300 ng) were included in MMLV RT reactions. Size standards (bp) are indicated at the left of the gel image.
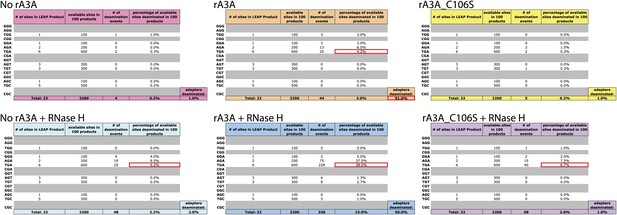
A3A Deamination Events in LEAP products.
Each chart lists all possible trinucleotide contexts with a ‘G’ as the middle nucleotide (corresponding to a ‘C’ on the first-strand LEAP cDNA). The first column shows the number of times each trinucleotide appears in the LEAP product sequence. Grayed-out rows indicate that the trinucleotide is not present in the LEAP product sequence. The second column lists how many times each trinucleotide is available in 100 LEAP products. The third column quantifies how many times a deamination event was observed within each trinucleotide. The fourth column quantifies the percent of available sites deaminated in 100 LEAP products. Colored rows below each chart indicate the overall frequency of deamination events of all ‘G’ nucleotides. An additional colored cell, below and to the right of each chart, lists the percent of sequences deaminated at the sole ‘C’ nucleotide in the single-strand oligonucleotide adapter sequence. Specific data points mentioned in the text are highlighted in red on each chart.
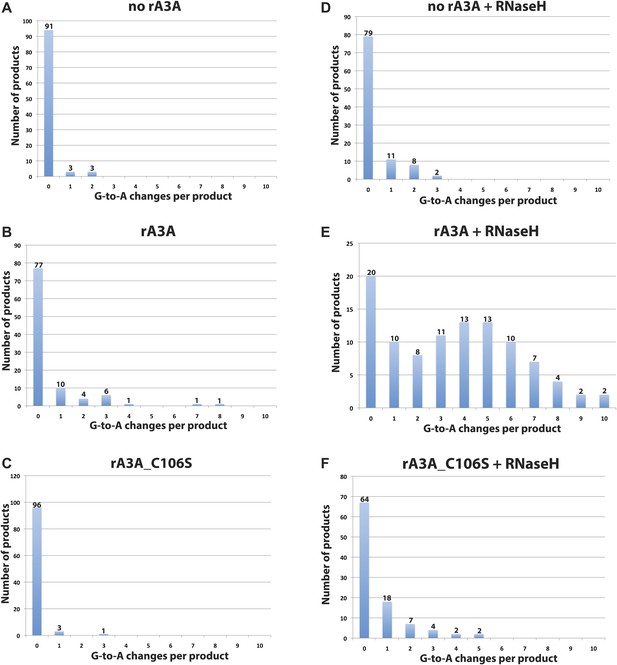
Distribution of deamination events per LEAP product.
The x-axis indicates the number of G-to-A changes; the y-axis indicates the number of LEAP products.
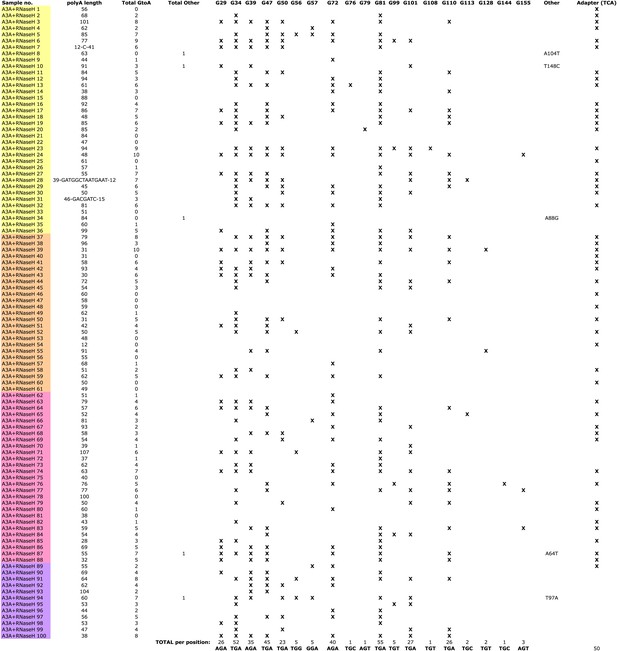
Summary of LEAP products generated in the presence of rA3A and RNase H.
Individual LEAP products (first column) were generated from four independent LEAP reactions, indicated by colored shading. For each product, poly-A tail length (second column), the total number of G-to-A changes (third column), and the total number of other nucleotide changes (fourth column) are indicated. Columns G29 through G155 represent the 19 nucleotide positions that were deaminated in WT rA3A-containing LEAP reactions. For each product, an ‘X’ indicates the specific location of a deamination event. The Adapter column indicates deamination events within the single-strand LEAP adapter.
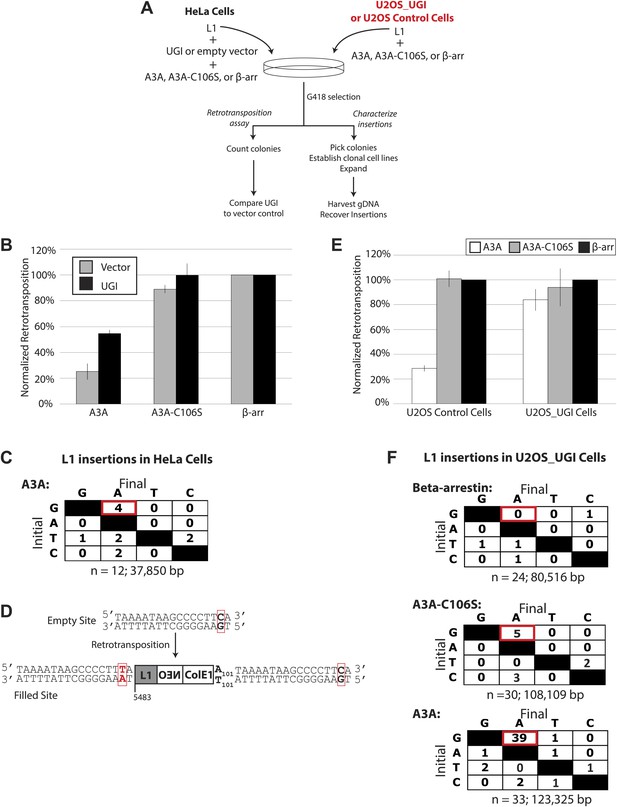
UGI expression alleviates A3A-mediated inhibition of L1 retrotransposition and allows detection of deaminated L1 retrotransposition events.
(A) Experimental strategy: cells (HeLa, upper left, or U2OS, upper right) were co-transfected with the indicated expression plasmids and assayed for L1 retrotransposition (bottom left branch) or insertion analysis (bottom right branch). (B) UGI expression alleviates A3A-mediated retrotransposition inhibition: the x-axis indicates the co-expression vector. The y-axis depicts the efficiency of L1 retrotransposition. Shown are results of experiments in the presence (black bars) or absence (gray bars) of UGI. The results were normalized as in Figure 1—figure supplement 1A. Data are expressed as the mean percent retrotransposition derived from three independent experiments consisting of two technical replicates each, with error bars representing the standard deviation among all six technical replicates. (C) DNA sequencing results: the left column indicates the engineered L1 sequence. The top row indicates sequence changes observed in recovered L1 insertions; n indicates the number of characterized retrotransposition events. The total retrotransposed sequence observed (in bp) includes the L1 sequence and the mneoI/ColE1 cassette. (D) An L1 insertion harboring mismatched TSDs: The pre- (empty) and post- (filled) L1 integration sites are shown. The truncation point (bp 5483) and structure of the engineered retrotransposed L1 are indicated. Deamination of single-strand genomic DNA in the pre-integration site (red rectangle) leads to inexact TSDs in the post-integration site (red lettering/rectangles). (E) Stable UGI expression alleviates A3A-mediated L1 inhibition: The x-axis indicates the U2OS cell line. The y-axis depicts the efficiency of L1 retrotransposition. Shown are the effects of wild-type A3A (white bars), A3A-C106S (gray bars), and β-arrestin (β-arr, black bars) control on L1 retrotransposition. Data were normalized to controls conducted with a circular NEO expression vector (Figure 3—figure supplement 1 B–C). Data are expressed as the mean percent retrotransposition derived from three independent experiments consisting of two technical replicates each, with error bars representing the standard deviation among all six technical replicates. (F) DNA sequencing results: The left column indicates the engineered L1 sequence. The top row indicates sequence changes observed in recovered L1 insertions; n indicates the numbers of characterized retrotransposition events. The total retrotransposed sequence observed (in bp) includes the L1 sequence and the mneoI/ColE1 cassette.
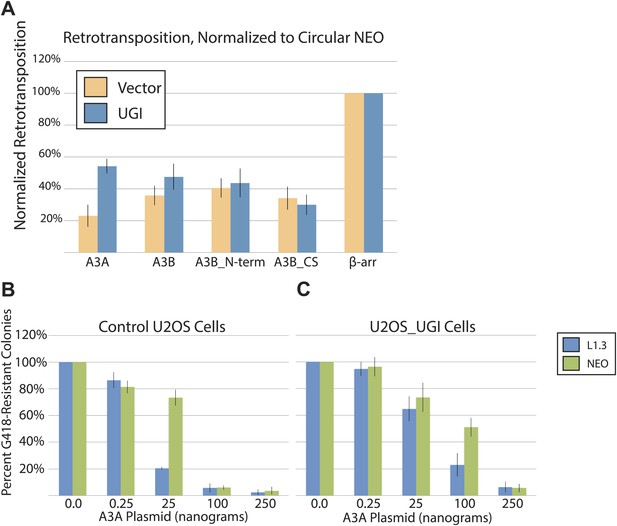
Additional Control Experiments.
(A) UGI does not alleviate L1 retrotransposition inhibition by APOBEC3B cytidine deaminase mutants (pK_A3B_Nterm and pK_A3B_CS): HeLa cells were transfected with 1 μg each of an APOBEC3 expression vector (WT pK_A3A, WT pK_A3B, deaminase-deficient pK_A3B_Nterm, deaminase-deficient pK_A3B_CS, or pK_ β-arrestin), an L1 vector (pJM101/L1.3), and pLGCX/UGI or pLGCX empty vector. The x-axis indicates the APOBEC3 expression construct. Orange bars: vector only control. The y-axis indicates percent retrotransposition, with the β-arrestin control for each reaction set to 100%. Blue bars: UGI expression. Data were normalized to circular NEO control co-transfections. Data are expressed as the mean percent retrotransposition derived from three independent experiments consisting of two technical replicates each, with error bars representing the standard deviation among all six technical replicates. Notably, UGI expression does not affect the ability of the deaminase-deficient A3B mutants (pK_A3B_Nterm and pK_A3B_CS) to inhibit L1 retrotransposition. (B) Effect of A3A expression on L1 retrotransposition and circular NEO controls in control U2OS cells: control U2OS cells were transfected with a total of 1.25 μg of DNA, including 1.0 μg of pJM101/L1.3 plasmid. For the β-arrestin control transfections, 250 ng of β-arrestin plasmid were used in the experiments. A3A transfections consisted of 0.0 ng, 0.25 ng, 25 ng, 100 ng, or 250 ng of A3A expression vector and the appropriate amount of β-arrestin plasmid to bring total plasmid mass to 1.25 μg. Blue bars indicate pJM101/L1.3; green bars indicate the pU6i NEO control. The x-axis shows the amount of A3A plasmid used in the experiment. The y-axis shows percent of G418-resistant colonies, with β-arrestin control co-transfection (0 ng A3A) set to 100%. Data are expressed as the mean percent G418-resistant colonies derived from two independent experiments consisting of two technical replicates each, with error bars representing the standard deviation among all four technical replicates. (C) Effect of A3A expression on L1 retrotransposition and circular NEO controls in U2OS_UGI cells: co-transfection experiments were carried out as described in Extended Data Figure 3B. Blue bars indicate pJM101/L1.3; green bars indicate pU6i NEO control. The x-axis shows the amount of A3A plasmid used in the experiment. The y-axis shows percent of G418-resistant colonies, with β-arrestin control co-transfection (0 ng A3A) set to 100%. Data are expressed as the mean percent G418-resistant colonies derived from two independent experiments consisting of two technical replicates each, with error bars representing the standard deviation among all four technical replicates.
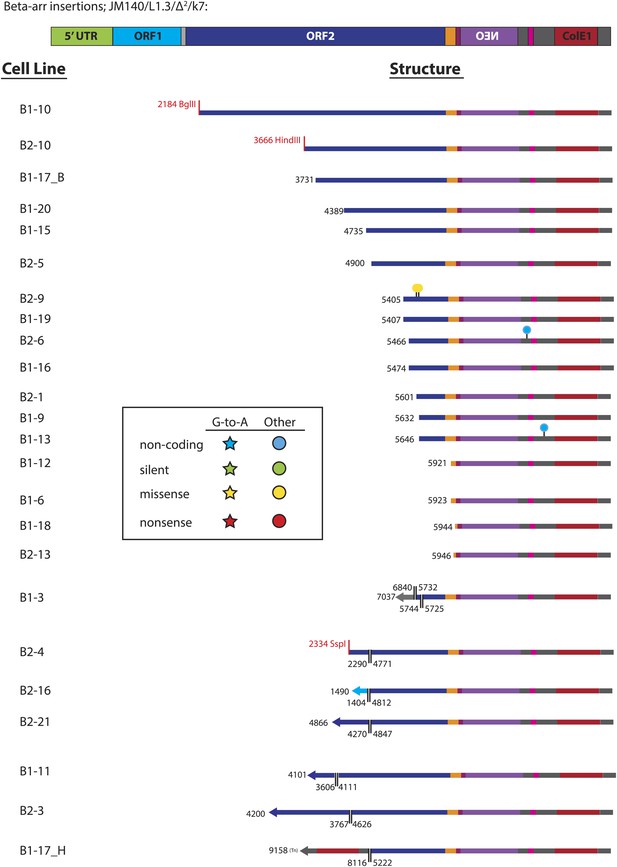
Summary of L1 retrotransposition events generated in U2OS_UGI cells in the presence of β-arrestin.
The pJM140/L1.3/Δ2/k7 retrotransposition indicator cassette is depicted at the top of the Figure. Features of the plasmid are shown to scale: 5′UTR (green), ORF1 (light blue), inter-orf spacer (light gray), ORF2 (dark blue), 3′UTR (orange), NEO cassette (purple) with HSV_tk polyA signal (maroon), SV40 promoter/ori (pink), ColE1 bacterial origin of replication (red), and plasmid backbone sequence between the above mentioned features (dark gray). The name of the cell line from which each insertion was recovered is listed at the left side. The length and structural features of characterized insertions are represented graphically at the right side. The truncation point of each insertion, relative to the pJM140/L1.3/Δ2/k7 retrotransposition indicator plasmid (Gilbert et al., 2005), is depicted in black. For inversion/deletion containing insertions, the break points are depicted in black, with a double line marking the inversion/deletion junction. The inverted sequence is depicted as a left-facing arrow. Some recovered insertions ended at the restriction site used in the recovery procedure; the sites are named in red lettering (e.g., see insertion B1-10). Nucleotide changes from the pJM140/L1.3/Δ2/k7 sequence are depicted as follows: stars indicate G-to-A changes; circles indicate any other nucleotide change. Blue shapes indicate changes in non-coding regions; green shapes indicate silent mutations; yellow shapes indicate missense mutations; red shapes indicate nonsense mutations.
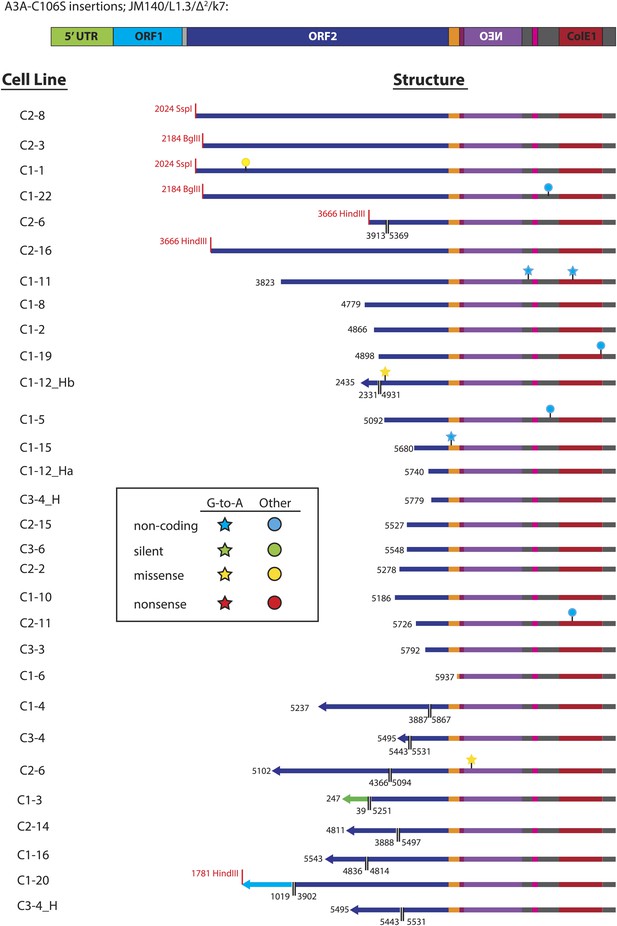
Summary of L1 retrotransposition events generated in U2OS_UGI cells in the presence of A3A_C106S.
Insertion features and nucleotide changes are depicted as described in Figure 3—figure supplement 2.
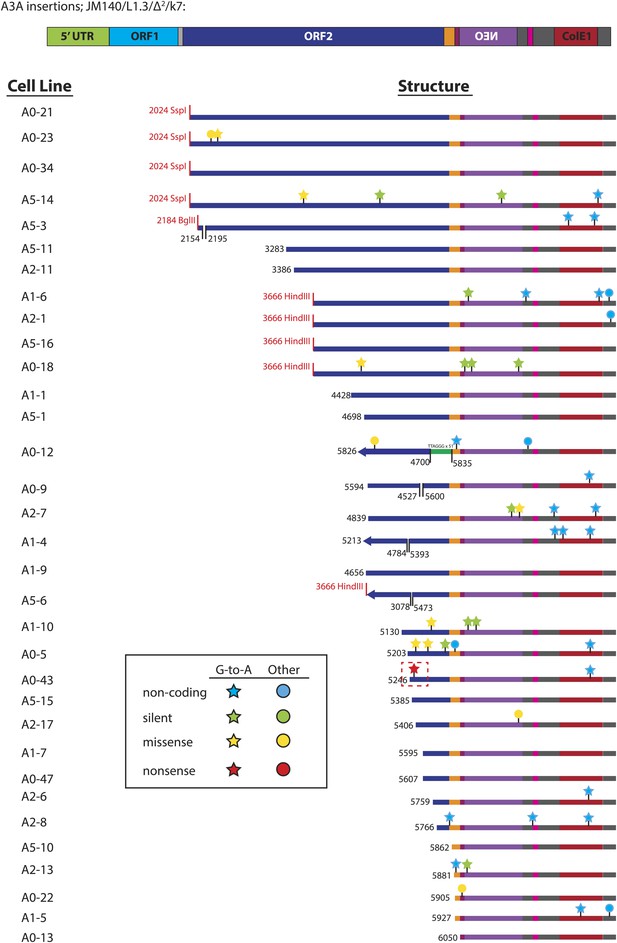
Summary of L1 retrotransposition events generated in U2OS_UGI cells in the presence of A3A.
Insertion features and nucleotide changes are depicted as described in Figure 3—figure supplement 2. The nonsense mutation G-5390-A recovered from cell line A0-43 is highlighted with a dotted red box. 51 copies of a TTAGGG telomeric repeat, highlighted by the green rectangle, were captured at the inversion/deletion junction of A0-12.
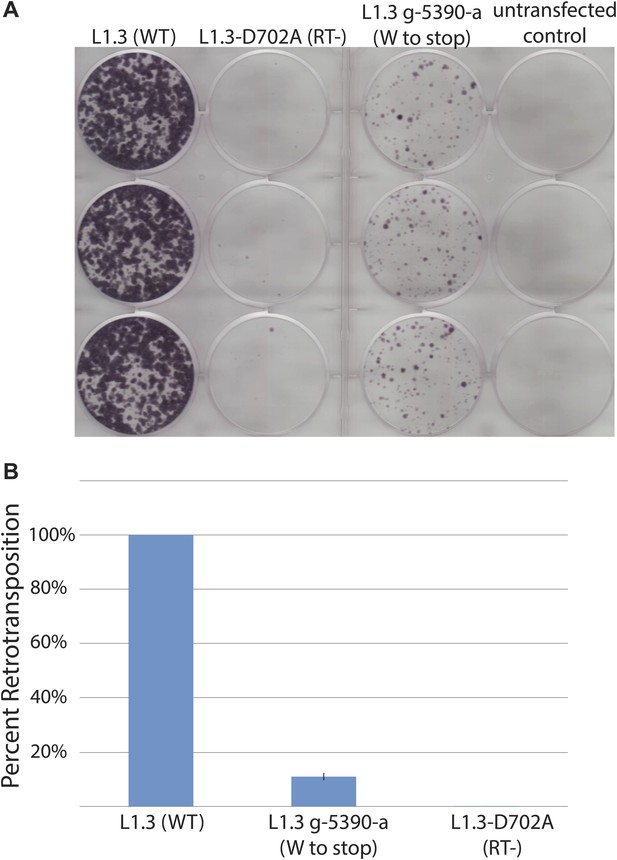
The L1.3 G-5390-A mutation decreases L1 retrotransposition efficiency.
The G-5390-A mutation was generated by site-directed mutagenesis on wild-type pJM101/L1.3. U2OS_UGI cells were transfected with 1 μg of retrotransposition indicator plasmid. (A) Representative retrotransposition assays: from left, wells contain pJM101/L1.3 (wild type), pDK135 (RT-deficient mutant), pJM101/L1.3/G-5390-A, and an untransfected control. (B) Quantification of retrotransposition efficiencies: the x-axis shows the elements used in the assay. RT- indicates the RT-deficient mutant negative control pDK135. The y-axis indicates the percent of retrotransposition relative to the pJM101/L1.3 control. Data are shown as the mean percent retrotransposition derived from two independent experiments consisting of three technical replicates each, with error bars representing the standard deviation among all six technical replicates.
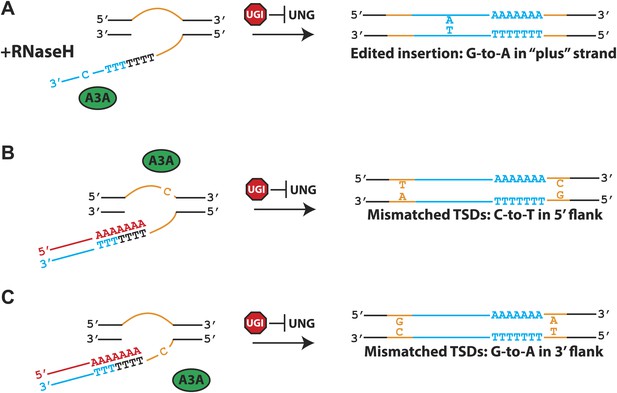
A model for A3A-mediated inhibition of L1 retrotransposition.
The left side of the Figure shows L1 integration reactions that occur via TPRT. The right side of the Figure shows the predicted structures of the resultant L1 retrotransposition events. Shown are transiently exposed single-strand genomic DNA regions that ultimately give rise to target site duplications (TSDs: orange lines), the L1 RNA (red line), the L1 cDNA (blue line), and the A3A protein (green oval). UGI expression (red stop sign) can inhibit UNG activity. (A) Deamination of the L1 cDNA: in the presence of cellular RNase H, A3A-mediated deamination of the L1 (−) strand cDNA (blue lettering) in the presence of UGI leads to C-to-T mutations on the L1 non-coding strand and G-to-A mutations on the L1 (+) coding strand. (B) Deamination of the 5′ flanking top-strand genomic DNA: in the presence of UGI, deamination of the transiently exposed single-strand 5′ flanking genomic DNA (top orange line) during TPRT results in C-to-T changes in the 5′ TSD relative to the 3′ TSD. (C) Deamination of the 3′ flanking bottom-strand genomic DNA: in the presence of UGI, deamination of transiently exposed single-strand 3′ flanking genomic DNA during TPRT, in principle, is predicted to result in a G-to-A change in the 3′ TSD relative to the 5′ TSD.