Sensory experience during locomotion promotes recovery of function in adult visual cortex
Figures
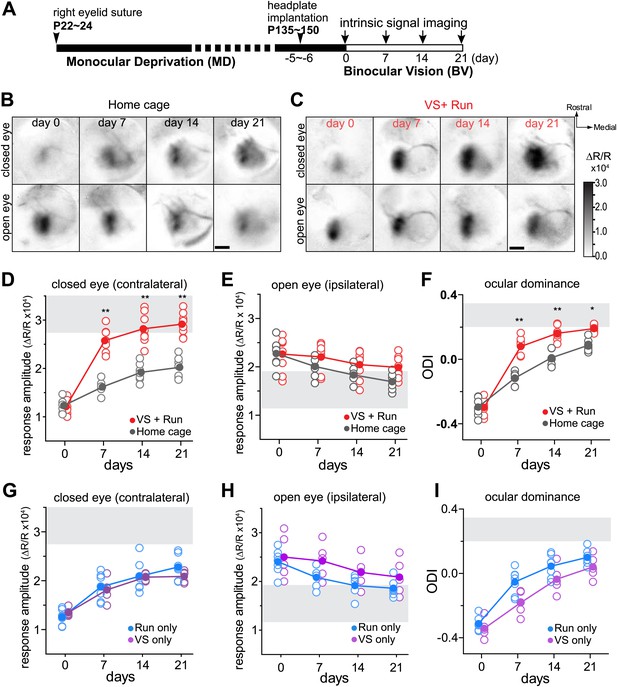
Visual stimulation during locomotion enhances recovery of cortical responses through the deprived eye after prolonged MD.
(A) Experimental schedule to examine changes in visual cortical responses over 21d-BV following prolonged MD started at postnatal day (P) 22–24. (B and C) Examples of intrinsic signal responses to the closed eye in the binocular visual cortex during 21d-BV in a home-cage control mouse (B) and in a mouse viewing contrast-modulated noise as VS during daily runs (VS+run, C). (D and E) Changes in intrinsic signal responses evoked by the noise through the closed (D) and open (E) eyes in home-cage (n = 8) and VS+run mice (n = 8). (F) Ocular dominance index (ODI) computed from response amplitude to contralateral (closed) and ipsilateral (open) eyes shown in D and E. ODI represents normalized difference in response magnitude between two eyes with 0 being equal amplitude to two eyes; the higher the number, more contralateral eye dominant. (G and H) Changes in intrinsic signal responses evoked by the noise through the closed (G) and open (H) eyes in run-only (n = 7) and VS-only mice (n = 7). (I) Ocular dominance index (ODI) computed from response amplitude to contralateral (closed) and ipsilateral (open) eyes shown in G and H. Gray area in D–I indicates the range of response amplitude or ODI in age-matched mice with normal visual experience. **p<0.01, *p<0.05, between groups.
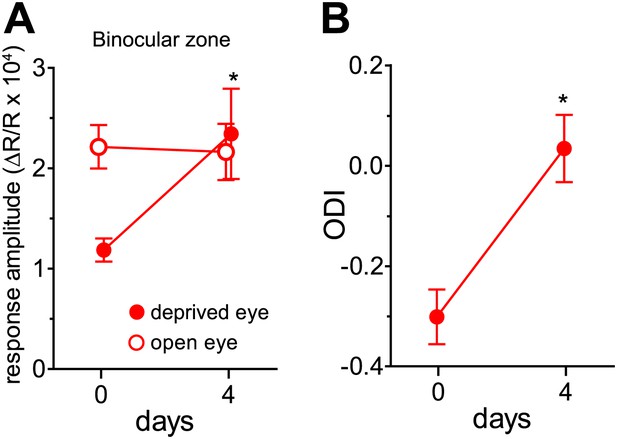
Effects of visual stimulation during locomotion after 4 days of binocular vision.
(A) Intrinsic signal responses to the noise through the deprived eye (solid circle) and open eye (open circle) in the binocular visual cortex on day 0 (before re-opening the deprived eye) and day 4 in the same individuals. Note that the animals experienced the contrast-modulated noise as VS during running daily for 4 hr on day 1, 2, and 3. (B) Ocular dominance index calculated from data shown in A. *p<0.01 compared to day 0, paired t test. n = 6.
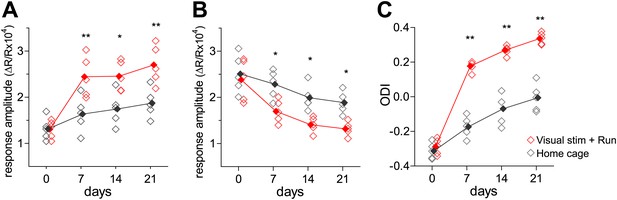
Changes in responses during reverse occlusion measured using intrinsic signal imaging.
(A) Responses to the contrast-modulated noise movie (noise) through the deprived eye in the binocular visual cortex in mice that were presented the noise as VS during daily running (VS+run: open red diamonds, n = 5) and in mice kept in the regular housing condition without VS or running (home-cage: open graygray diamonds, n = 5). (B) Responses to the contrast-modulated noise movie (noise) through the previously-open, newly-closed eye in the binocular visual cortex in VS+run mice (open red diamonds) and in home-cage mice (open graygray diamonds). (C) Ocular dominance index computed from data presented in A and B. For A–C, closed symbols indicate group average. **p<0.01, *p<0.05 between groups.
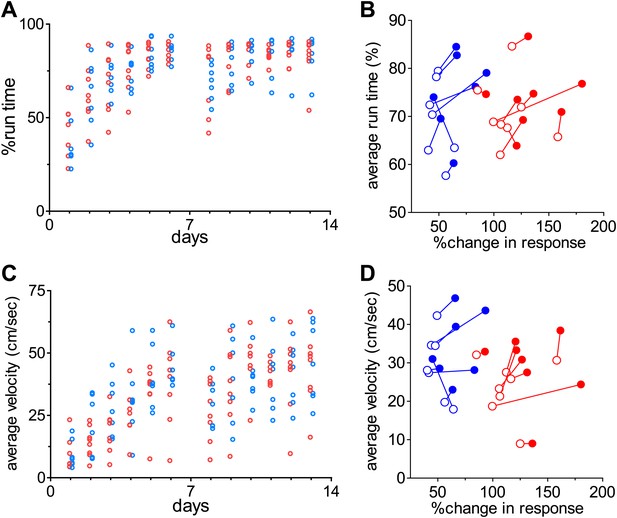
Monitoring of locomotion while the mice are on the track-ball.
(A) Percent of time during which individual animals were moving (>1 cm/s, as opposed to standing stationary) on the spherical treadmill. (B) Relationship between the change in response amplitude measured by intrinsic signal imaging and average moving time for day 1 through 6 (open circles) and day 1 through 13 (closed circles) in individual animals. Points from the same animals are connected by lines. (C) Average moving velocity of individual animals shown in A. (D) Relationship between the change in response amplitude measured by intrinsic signal imaging and average moving velocity for day 1 through 6 (open circles) and for day 1 through 13 (closed circles). Points from the same animals are connected by lines. Red circles: animals that were exposed to the visual stimuli during running (n = 8). Blue circles: animals that ran with blank screen (n = 7). Note that there was no clear correlation between the magnitude of changes in response strength and the amount of time or speed of locomotion.
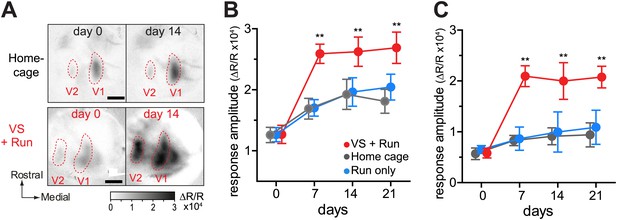
Visual stimulation during locomotion enhances recovery of cortical responses in the monocular visual cortex after prolonged MD.
(A) Examples of intrinsic signal images of monocular visual areas. (B and C) Changes in intrinsic signal magnitudes (mean ± SEM) through the closed eye in response to the noise in the monocular V1 (B) and monocular secondary visual cortex (C) (same animals shown in Figure 1D–F). **p<0.01, between groups.
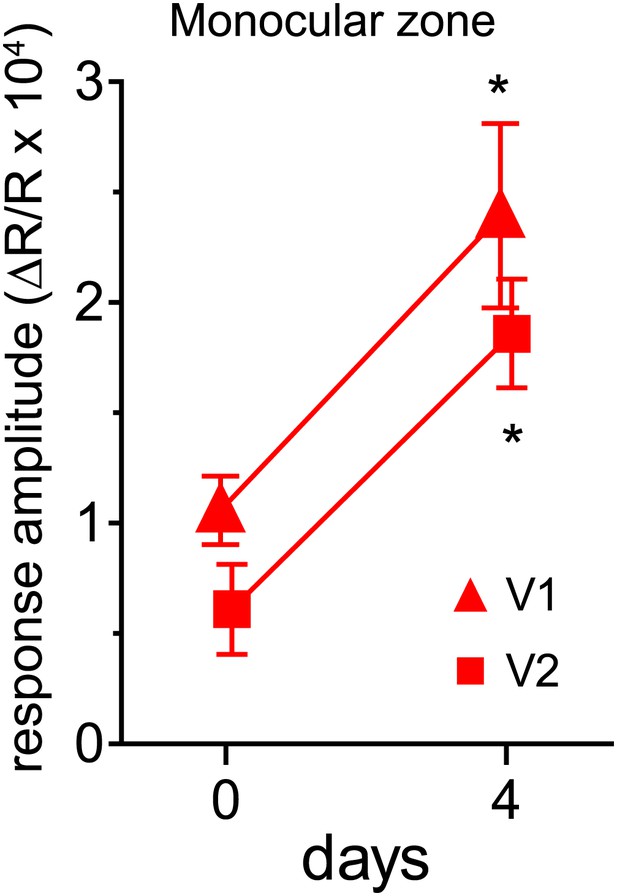
Effects of visual stimulation during locomotion on responses in the monocular zone after 4 days of binocular vision.
Intrinsic signal responses to the noise through the deprived eye in the monocular primary (V1-MZ) and monocular secondary (V2-MZ) visual cortex. *p<0.01 compared to day 0, paired t test. n = 6.
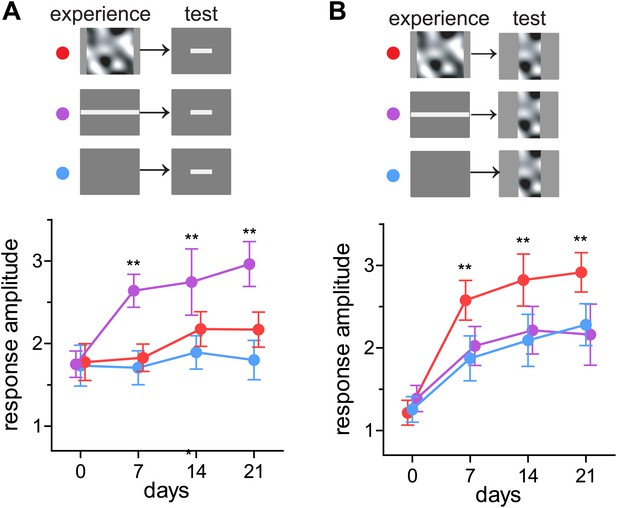
Preferential enhancement of recovery of closed-eye responses to the visual stimuli experienced during locomotion.
(A) Peak intrinsic signal amplitude in response to bar stimuli through the closed eye in mice that experienced noise (noiseVS+run, red, n = 6), drifting bars (barVS+run, n = 6), or a blank screen during running (blue, n = 6). (B) Peak intrinsic signal amplitude in response to bar stimuli in same mice as in A. Data are show mean ± SD. **p<0.01 and *p<0.05 compared with the blank-screen control (blue).
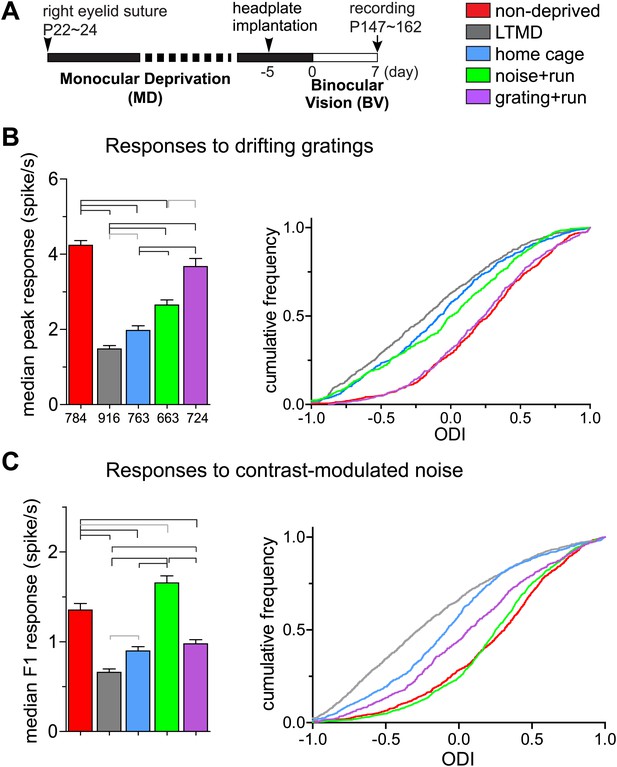
Response magnitude of individual broad-spiking cells to drifting gratings and contrast-modulated noise.
(A) Experimental schedule for single unit recording. Color-coded bars for different treatment groups apply to all panels. (B) Response magnitude to drifting gratings. Left panel: median (±s.e.) response rates to optimal drifting gratings through the deprived eye. Right panel: cumulative frequency distribution of ocular dominance index (ODI) in cells that were responsive (>2 spikes/s) through either deprived or open eye. ODI was computed from response magnitude to optimal gratings through each eye as shown in Figure 4—figure supplement 1A. (C) Response magnitude to contrast-modulated noise. Left: median F1 response (±s.e.) to the noise stimulus through the deprived eye. Right: cumulative frequency distribution of ODI calculated for each cell that were responsive to the noise (F1 response >0.2) through either eye from the data shown in Figure 4—figure supplement 1B. Data in left panels of B and C are from same populations of all cells isolated, as numbers are indicated below bars in B. Horizontal lines above bars; black: p<0.01, gray: p<0.05. Results of Kolmogorov–Smirnov tests for cumulative frequency distributions are shown in Table 1.
-
Figure 4—figure supplement 2—source data 1
This refers to Panel A. Responses of 916 broad-spiking cells to two different stimuli in spikes/sec in LTMD animals without subsequent binocular vision. Column 1 shows peak responses to the optimal grating. Column 2 shows response to the contrast modulated noise pattern at the fundamental (F1) frequency of contrast modulation.
- https://doi.org/10.7554/eLife.02798.013
-
Figure 4—figure supplement 2—source data 2
This refers to Panel B. Responses of 663 broad-spiking cells to two different stimuli in spikes/sec in run+noise mice. Columns as in Figure 4—Figure supplement 2—source data 1.
- https://doi.org/10.7554/eLife.02798.014
-
Figure 4—figure supplement 2—source data 3
This refers to Panel C. Responses of 724 broad-spiking cells to two different stimuli in spikes/sec in run+gratings mice. Columns as in Figure 4—Figure supplement 2—source data 1.
- https://doi.org/10.7554/eLife.02798.015
-
Figure 4—figure supplement 2—source code 1
Computer code using earth mover distance algorithm for analysis of fictive changes in response.
- https://doi.org/10.7554/eLife.02798.025
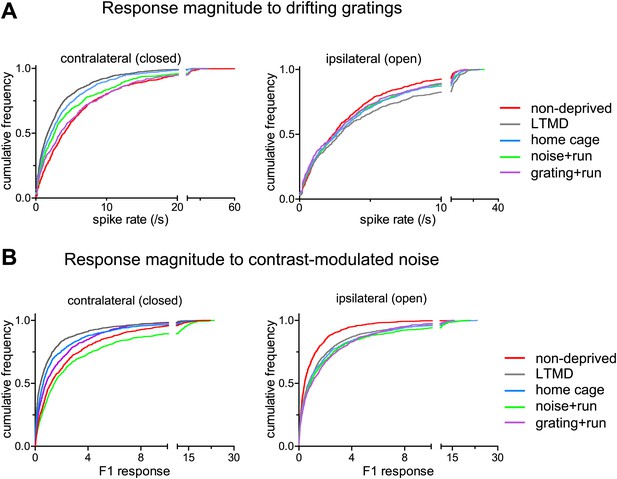
Response magnitudes of individual broad-spiking cells to drifting gratings and contrast-modulated noise.
(A) Cumulative frequency distribution of peak responses to the optimal gratings through the deprived eye (Left) and open eye (Right) of all isolated broad-spiking cells. (B) Cumulative frequency distribution of the peak response to the contrast-modulated noise through the deprived eye (Left) and the open eye (Right). The peak response of a cell was elicited at the frequency of contrast modulation (F1, the first harmonic at 0.1 Hz). Ocular Dominance Index shown in Figure 4 was calculated based on data shown here. Results of Kolmogorov–Smirnov tests for all panels are shown in Table 1.
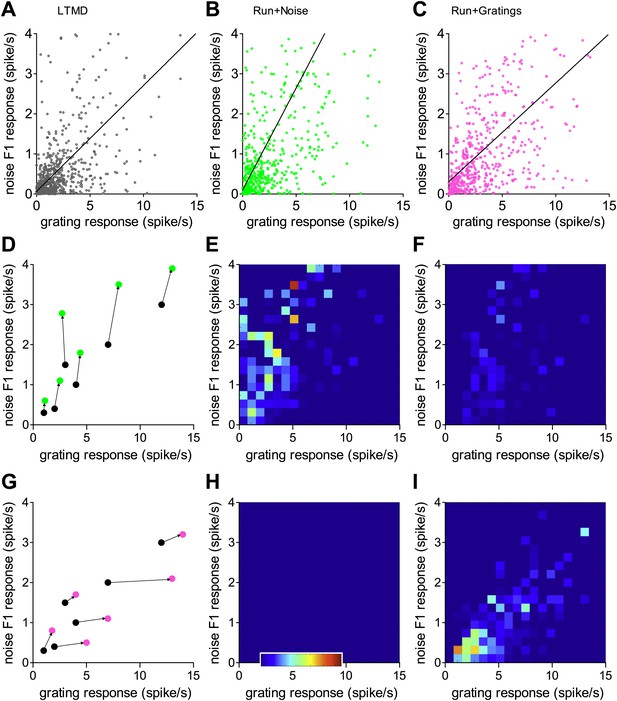
Analysis of fictive longitudinal data on the stimulus specificity of recovery during locomotion.
Scatter plots of the responses to noise and grating stimuli of three populations of broad-spiking cells, (A) before recovery and after recovery during locomotion viewing (B) noise or (C) gratings. Data from noise+run mice shown in B, D, E, H; data from grating+run mice shown in C, F, G, I. We used the earth mover distance algorithm (Y Rubner et al., A metric for distributions with applications to image databases. Proc. IEEE International Conference on Computer Vision, Bombay, India, January 1998, pp. 59–66) to calculate the fictive correspondence between the 500 most responsive individual neurons of A and either B or C as the minimum total distance required to move the points of one distribution into the other, cartooned for simple cases in D for noise and G for grating responses. The flow field of movement vectors (arrows in D and G) is plotted for each recovery condition as a heat map of the summed components along the noise response axis (E and F) or grating response axis (H and I). Color bar inset in H indicates four spikes/sec along noise F1 response axis in E and F and 15 spikes/sec along grating response axis in H and I. These heat maps show that the effect of noise stimulation was to increase the response to noise stimuli selectively in neurons that were initially more responsive to noise than to gratings, as indicated by warm spots above the diagonal in E and lack of warm spots in H. The effect of grating stimulation was to increase the response to grating stimuli selectively in neurons that were initially more responsive to gratings than to noise, as indicated by warm spots below the diagonal in I, and paucity of warm spots in F, (p<0.001 for all comparisons E vs H, F vs I, Chi-square). Computer source code used for analysis and source data are supplied in supplement.
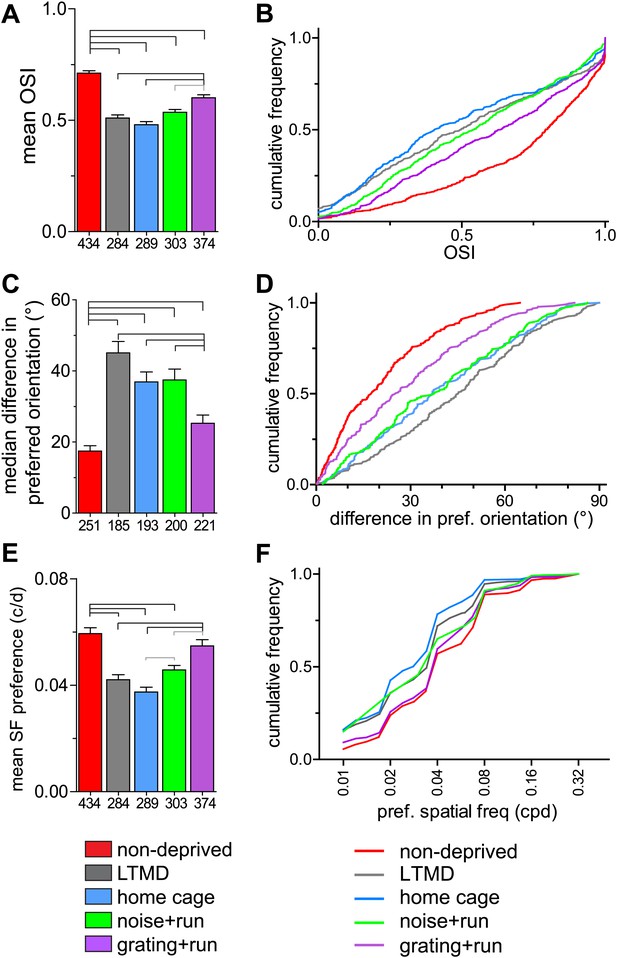
Tuning properties of individual broad-spiking cells in response to drifting gratings.
(A and B) Orientation tuning of deprived-eye responses, expressed as mean (±SEM) orientation selectivity index (OSI) (A) and cumulative frequency distribution of OSI (B). (C and D) Binocular matching of preferred orientation. Absolute differences in preferred orientation between two eyes in binocularly responsive cells are presented as the median (±s.e.) (C) and the cumulative frequency distribution (D). (E and F) Spatial frequency tuning. Preferred spatial frequencies at the preferred orientation of drifting gratings through the deprived eye are shown as mean (±SEM) (E) and cumulative frequency distribution (F). Sample sizes are indicated below bars. Horizontal lines above bars; black: p<0.01, gray: p<0.05. Results of Kolmogorov–Smirnov tests for B, D, F are shown in Table 1.
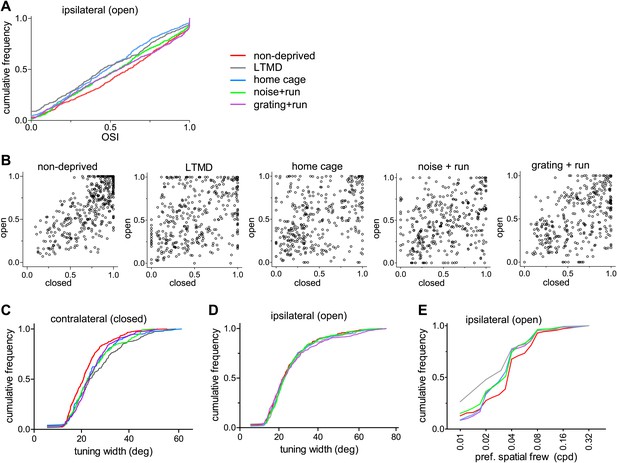
Tuning properties of broad-spiking cells examined with drifting sinusoidal gratings.
(A) Cumulative frequency distribution of orientation selectivity index (OSI) for responsive cells through the open eye. (B) Correlation of orientation selectivity index (OSI) between contralateral (closed) eye (abscissa) and ipsilateral (open) eye (ordinate). Mice with normal visual experience (non-deprived): r = 0.71, 418 pairs; after LTMD: r = 0.37, 389 pairs; after 7 days of BV in home cage: r = 0.34, 394 pairs; after 7 days of BV with the noise as VS during running (noise+run): r = 0.34, 368 pairs; and after 7 days of BV with the gratings as VS during running (grating+run): r = 0.51, 341 pairs. (C and D) Cumulative frequency distribution of the orientation tuning width of responses to the gratings through the deprived eye (C) and the open eye (D). The tuning width was defined as the half-width at half-maximum above the baseline, for orientation-selective cells (OSI >0.5). (E) Cumulative frequency distribution of preferred spatial frequency in responsive cells (>2 spikes/s) through the open eye. Results of Kolmogorov–Smirnov tests for A, and C–E are shown in Table 1.
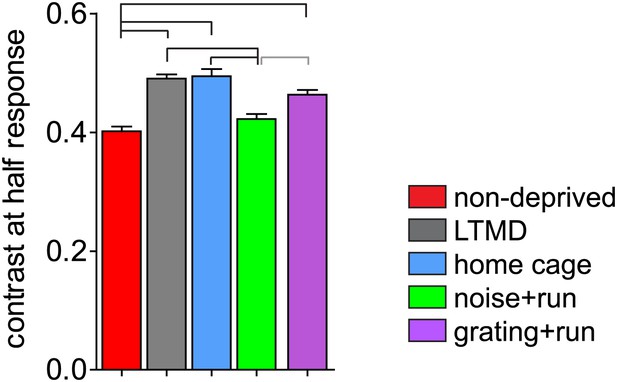
Change in contrast sensitivity in broad-spiking cells.
Average values of contrast that gives half-maximal response are shown. Horizontal lines above bars; black: p<0.01, grey: p<0.05.
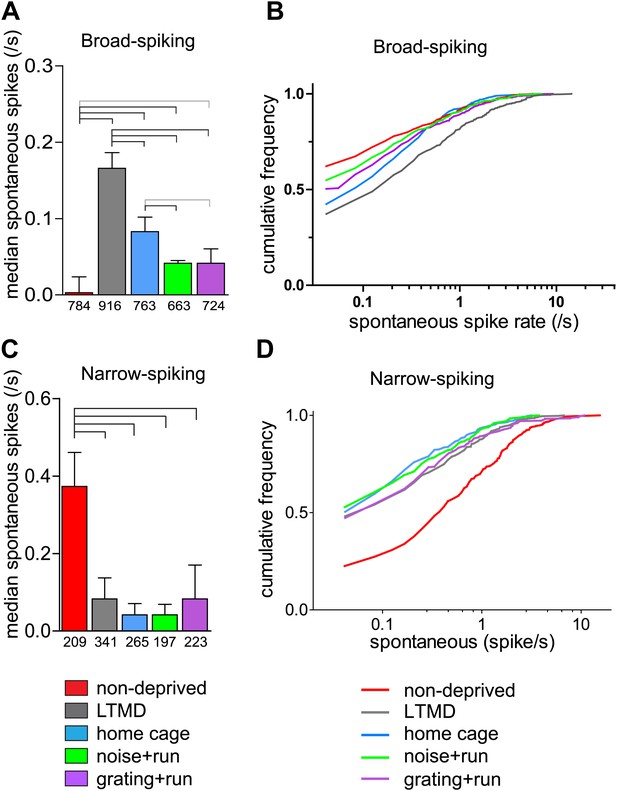
Spontaneous firing of isolated broad-spiking and narrow-spiking cells recorded simultaneously.
(A and B) The median (±s.e.) number (A) and cumulative frequency distribution (B) of spontaneous firing rates of all broad-spiking cells isolated during the presentation of blank screen randomly interspaced in the drifting grating set. (C and D) The median (±s.e.) number (C) and cumulative frequency distribution of spontaneous firing rates of all narrow-spiking cells. Sample sizes are indicated in A and C. Horizontal lines above bars; black: p<0.01, grey: p<0.05. Results of Kolmogorov–Smirnov tests for B and D are shown in Table 1.
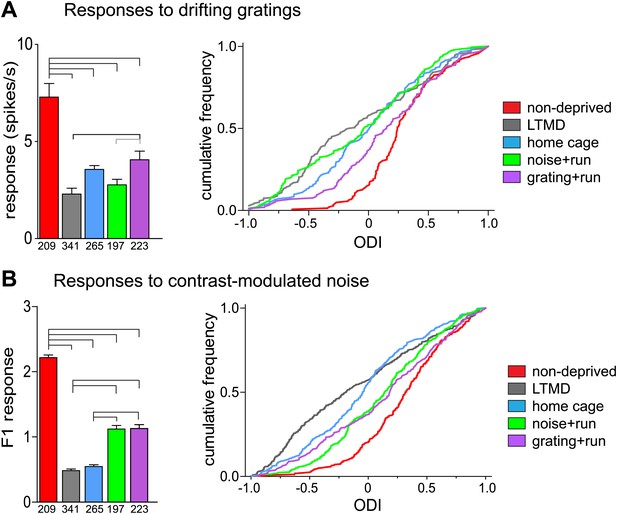
Responses of isolated narrow-spiking cells recorded simultaneously with broad-spiking cells.
(A) Responses to drifting sinusoidal gratings of all narrow-spiking cells isolated. Left: median ±s.e. of peak responses to the optimal gratings through the deprived eye. Right: cumulative frequency distribution of ocular dominance index (ODI) calculated for each cell from peak responses through the deprived eye (as shown in Figure 8—figure supplement 1A) and the open eye (Figure 8—figure supplement 1B). (B) Responses to contrast-modulated noise in all narrow-spiking cells isolated. Left: median ±s.e. of F1 responses through the deprived eye. Right: cumulative frequency distribution of ocular dominance index (ODI) calculated for each cell from F1 responses through the deprived eye (as shown in Figure 8—figure supplement 1C) and the open eye (Figure 8—figure supplement 1D). Horizontal lines above bars; black: p<0.01, grey: p<0.05. Results of Kolmogorov–Smirnov tests for cumulative frequency distributions are shown in Table 1.
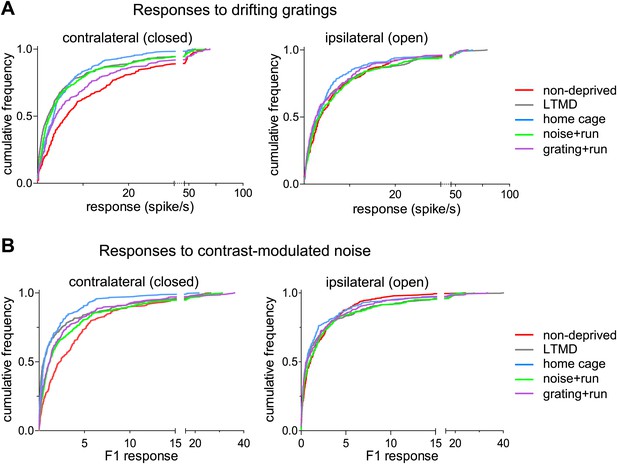
Responses of isolated narrow-spiking cells recorded simultaneously with broad-spiking cells.
(A) Responses to drifting sinusoidal gratings. Cumulative frequency distribution of peak responses to the optimal gratings through the deprived eye (left panel) and the open eye (right panel). (B) Responses to the contrast-modulated noise. Cumulative frequency distribution of F1 responses through the deprived eye (left panel) and the open eye (right panel). Results of Kolmogorov–Smirnov tests are shown in Table 1.
Tables
Results of Kolmogrov-Smirnov tests for cumulative frequency distributions
Groups compared | Non-deprived | Non-deprived | Non-deprived | Non-deprived | LTMD | LTMD | LTMD | Home cage | Home cage | noise + run |
---|---|---|---|---|---|---|---|---|---|---|
LTMD | Home cage | noise+run | grating+run | Home cage | noise+run | grating+run | noise+run | grating+run | grating+run | |
Broad-spiking cells | ||||||||||
Spontaneous firing | <0.00001 | <0.0001 | <0.001 | <0.05 | <0.01 | <0.01 | <0.001 | <0.01 | <0.05 | <0.01 |
Grating response: closed | <0.00001 | <0.00001 | <0.01 | n.s. | n.s. | <0.0001 | <0.05 | <0.05 | <0.0001 | <0.01 |
Grating response: open | <0.01 | <0.05 | <0.05 | n.s. | n.s. | n.s. | n.s. | n.s. | n.s. | n.s. |
Grating response: ODI | <0.00001 | <0.00001 | <0.0001 | n.s. | <0.01 | <0.001 | <0.00001 | <0.01 | <0.00001 | <0.001 |
|dO| | <0.00001 | <0.00001 | <0.00001 | <0.01 | n.s. | n.s. | <0.001 | n.s. | <0.001 | <0.001 |
OSI: closed | <0.00001 | <0.00001 | <0.00001 | <0.00001 | n.s. | <0.05 | <0.001 | <0.001 | <0.001 | <0.01 |
OSI: open | <0.01 | <0.01 | <0.05 | <0.05 | n.s. | n.s. | n.s. | n.s. | n.s. | n.s. |
Orientation tuning width: closed | <0.0001 | <0.05 | <0.05 | <0.05 | n.s. | n.s. | <0.05 | n.s. | n.s. | n.s. |
Orientation tuning width: open | n.s. | n.s. | n.s. | n.s. | n.s. | n.s. | n.s. | n.s. | n.s. | n.s. |
Preferred SF: closed | <0.001 | <0.00001 | <0.001 | n.s. | n.s. | n.s. | <0.05 | <0.05 | 0.001 | <0.05 |
Preferred SF: open | <0.00001 | <0.001 | <0.001 | <0.001 | <0.01 | <0.01 | <0.01 | n.s. | n.s. | n.s. |
Noise response (F1): closed | <0.00001 | <0.0001 | <0.01 | <0.001 | <0.05 | <0.00001 | <0.001 | <0.00001 | <0.05 | <0.0001 |
Noise response (F1): open | <0.0001 | <0.0001 | <0.0001 | <0.00001 | n.s. | n.s. | n.s. | n.s. | n.s. | n.s. |
Noise response: ODI | <0.00001 | <0.00001 | n.s. | <0.0001 | <0.0001 | <0.00001 | <0.0001 | <0.00001 | <0.001 | <0.001 |
Narrow-spiking cells | ||||||||||
Spontaneous firing | <0.00001 | <0.00001 | <0.00001 | <0.00001 | n.s. | n.s. | n.s. | n.s. | n.s. | n.s. |
Grating response: closed | <0.00001 | <0.0001 | <0.0001 | <0.001 | n.s. | n.s. | <0.01 | n.s. | <0.05 | <0.05 |
Grating response: open | n.s. | n.s. | n.s. | n.s. | n.s. | n.s. | n.s. | n.s. | n.s. | n.s. |
Grating response: ODI | <0.00001 | <0.001 | <0.0001 | <0.05 | <0.01 | n.s. | <0.001 | n.s. | n.s. | <0.05 |
OSI: closed | <0.001 | <0.0001 | n.s. | n.s. | n.s. | n.s. | n.s. | <0.01 | <0.01 | n.s. |
OSI: open | <0.01 | <0.01 | <0.01 | <0.01 | n.s. | n.s. | n.s. | n.s. | n.s. | n.s. |
Noise response (F1): closed | <0.001 | <0.001 | <0.01 | <0.01 | <0.05 | <0.01 | <0.05 | <0.01 | n.s. | n.s. |
Noise response (F1): open | n.s. | n.s. | n.s. | n.s. | n.s. | n.s. | n.s. | n.s. | n.s. | n.s. |
Noise response: ODI | <0.00001 | <0.00001 | <0.001 | <0.01 | <0.001 | <0.0001 | <0.0001 | <0.001 | <0.001 | <0.05 |
-
Statistical significance levels are indicated after adjusting p-values using Bonferroni correction for multiple comparisons. n.s.:p>0.05. closed: measures through deprived eye, open: measures through open eye. ODI: ocular dominance index. |dO|: absolute difference in preferred orientation between left and right eyes. OSI: orientation selectivity index.