Identification of motor neurons and a mechanosensitive sensory neuron in the defecation circuitry of Drosophila larvae
Figures
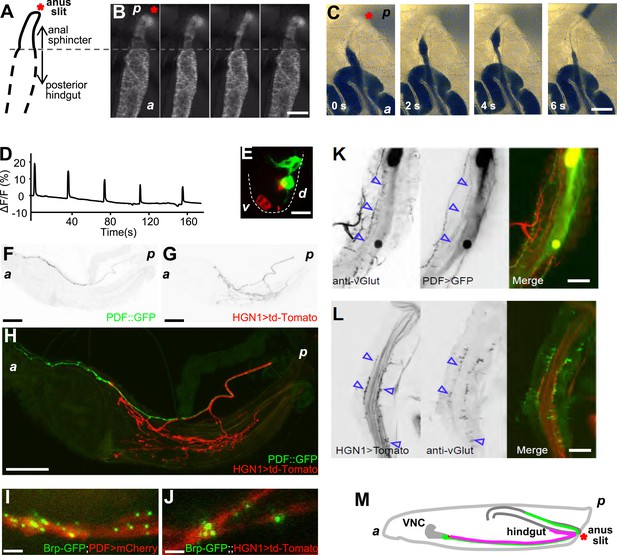
The periodical defecation process of the Drosophila larvae and the innervation of hindgut and anal sphincter by motor neurons.
(A and B) Sequential contractions of the posterior hindgut and anal sphincter. (A) Schematic representation of the posterior hindgut and anal sphincter. The posterior hindgut: dashed line; anal sphincter: solid line. (B) Posterior hindgut and anal sphincter contract sequentially in the defecation process (visualized with byn-Gal4 > GFP). From left to right: quiescent state, contraction of the posterior hindgut, contraction of the anal sphincter, and back to resting state (scale bar: 100 µm). (C) Posterior hindgut and anal sphincter contract sequentially to expel the feces out. From left to right: quiescent state, feces pushed to anus via hindgut contraction, contraction of anal sphincter, and end of defecation cycle (scale bar: 100 µm). (D) Time course of defecation cycle measured by GFP fluorescence intensity as in (B). A region of interest (ROI) was drawn on the posterior hindgut; the fluorescence intensity in ROI increased due to the tissue compression. (E) Cell bodies of PDF neurons (green, PDF-GFP) and HGN1 neurons (red, HGN1 > UAS-tdTomato) in the terminal segment of the ventral nerve cord (VNC) (scale bar: 20 µm). (F–H) Innervations in the gut of neurons labeled with PDF (green, PDF-GFP) and HGN1 (red, HGN1 > UAS-tdTomato). PDF (F) and HGN1 (G) axons (scale bar: 100 µm). (I and J) Buttons of PDF (I) and HGN1 (J) neurons labeled by Brp-GFP (green dots) along axons (red) (scale bar: 10 µm). (K and L) Anti-vGlut staining of PDF and HGN1 axons on hindgut. (K) Anti-vGlut staining on hindgut (red) in PDF-GFP larvae (green). Blue arrow heads indicate axon terminals (scale bar: 50 µm). (L) Anti-vGlut staining on anal sphincter (green) in HGN1 > tdTomato larvae (red). Blue arrow heads indicate axon terminals (scale bar: 50 µm). (M) Schematic representation of the PDF and HGN1 neurons and their innervations on gut in a whole animal lateral view.
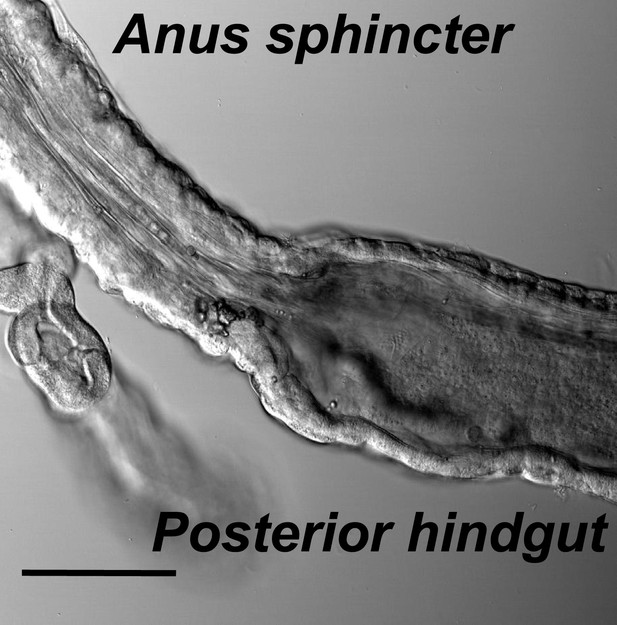
The muscle structures of hindgut and anal sphincter.
Hindgut is with a thin layer of muscles and a wide lumen, while the anal sphincter muscles are thicker and form a much narrower canal (scale bar: 100 µm).
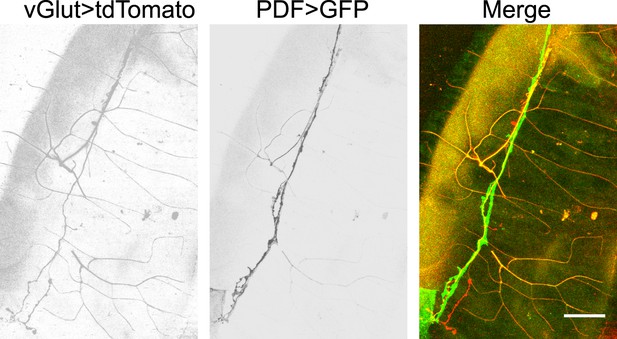
Glutamatergic innervations of motor neurons on the hindgut.
(From left to right) Motor neuron axons labelled with vGLUT-Gal4 driven tdTomato; PDF neuron specific GFP; merge of two channels (scale bar: 50 µm).
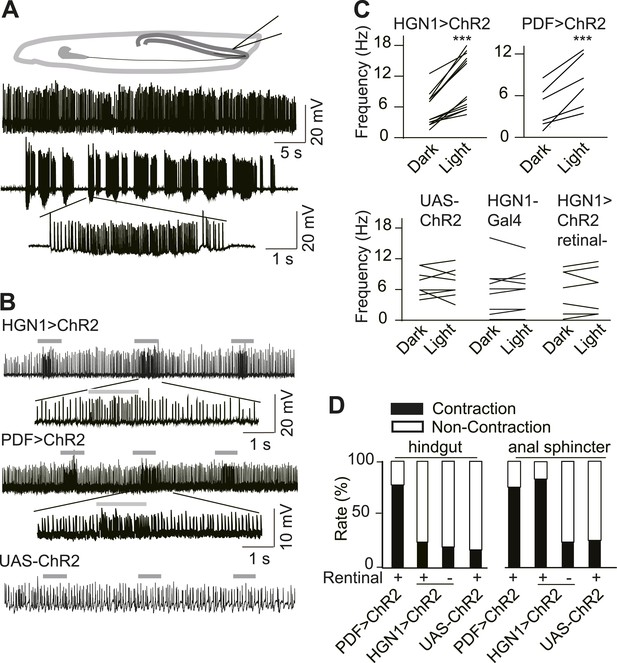
Excitatory output from VNC neurons to gut muscles.
(A) Spontaneous EJP activity of the anus sphincter muscles. Top panel: tonic input to the muscles; middle panel: burst inputs recorded in the muscles; lower panel: zoom-in of the spikes in a single burst. (B) Light induced activation in the gut muscles. Top panel: light-triggered EJPs increase in the anus sphincter muscles of HGN1 > ChR2 larva. Grey bars indicate the blue light. Lower panel: light stimulation on UAS-ChR2 larva as control. (C) Paired plot of the EJP frequency in dark and light condition (n = 15, ***p < 0.001, paired t test). (D) Light-triggered gut contractions in larvae carrying ChR2 in their PDF or HGN1 neurons.
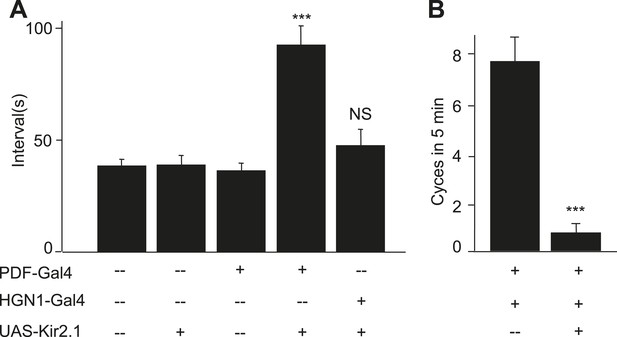
PDF and HGN1 motor neurons are essential for the defecation behavior.
(A) Silencing PDF but not HGN1 neurons increased the defecation intervals (***p < 0.001, one-way ANOVA). (B) Silencing both PDF and HGN1 neurons reduced the defecation frequency dramatically (***p < 0.001, paired t test).
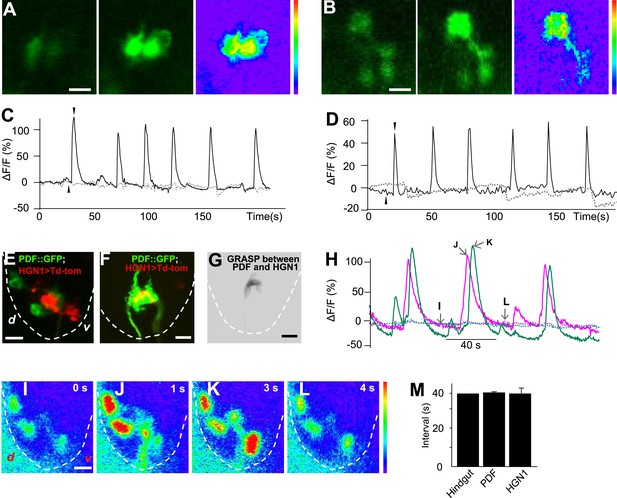
Sequential firing of the PDF and HGN1 neurons.
(A) Spontaneous Ca2+ oscillation imaged by GCaMP5 in PDF neurons in vivo. From left to right, Ca2+ signal of PDF neurons in quiescent state; peak Ca2+ signal of PDF neurons; Ca2+ intensity increase (scale bar: 20 µm). (C) Ca2+ oscillates over time. Solid line: GCaMP5; dashed line: RFP, the two black arrow heads indicate the time points of left and middle panel in A. (B) Spontaneous Ca2+ oscillation imaged by GCaMP5 in HGN1 neurons in vivo. From left to right, Ca2+ signal of HGN1 neurons in quiescent state; peak Ca2+ signal of HGN1 neurons; Ca2+ intensity increase (scale bar: 20 µm). (D) Ca2+ oscillates over time. Solid line: GCaMP5; dashed line: RFP, the two black arrow heads indicate the time points of left and middle panel in (B). (E) PDF (green) and HGN1 (red) neurons at the lateral view of the VNC. d: dorsal; v: ventral. (F) Neuropil of PDF and HGN1 neurons overlap (scale bar: 20 µm). (G) Grasp signal that resembles the location and shape of neuropil co-localization (scale bar: 20 µm). (H) Spontaneous Ca2+ oscillation imaged by GCaMP5 in PDF (purple) and HGN1 (green) neurons simultaneously in vivo. Dashed line: RFP recorded at the same time. Arrows indicate the time points of the images as shown in (I–L). (I–L), representative images from H. Color bar shows the range (1–256) (scale bar: 20 µm). (M) Form left to right; intervals of defecation and Ca2+ oscillation of PDF and HGN1 neurons (error bar: S.E.M., n = 8, 7, and 7).
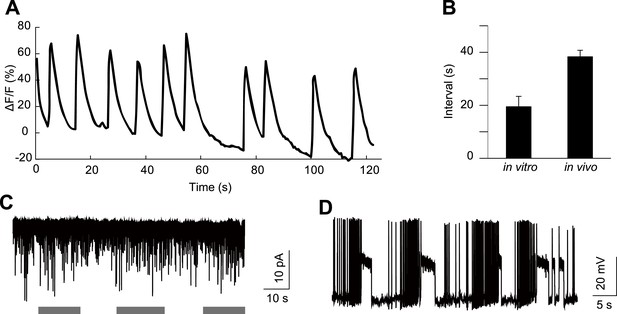
HGN1 neurons' rhythmic activity in dissected VNC.
(A) Ca2+ oscillation of HGN1 neurons in a dissected VNC. (B) Interval between Ca2+ peaks of HGN1 neurons in dissected VNC was decreased. (C) HGN1 neurons received periodical excitatory inputs (indicated by grey bars). (D) HGN1 neurons exhibited burst firing.
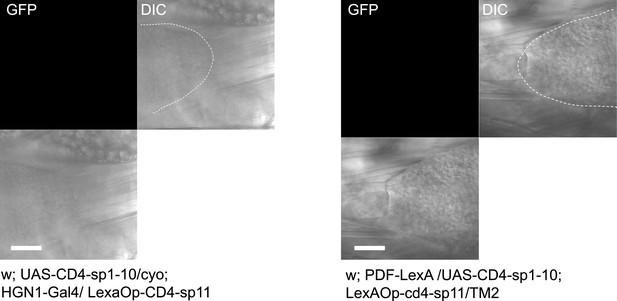
Single component of split-GFP did not show fluorescence.
https://doi.org/10.7554/eLife.03293.012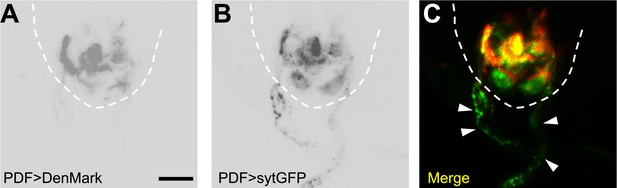
PDF neurons have axonal projections inside the VNC.
(A) PDF neurons' dendrites labelled with Denmark. (B) PDF neurons' axons visualized with sytGFP. (C) Merged image showing the intense co-localization between PDF axons and the HGN1 neuron dendritic area. Arrowheads highlighting the PDF axons out of the VNC (scale bar: 20 µm).
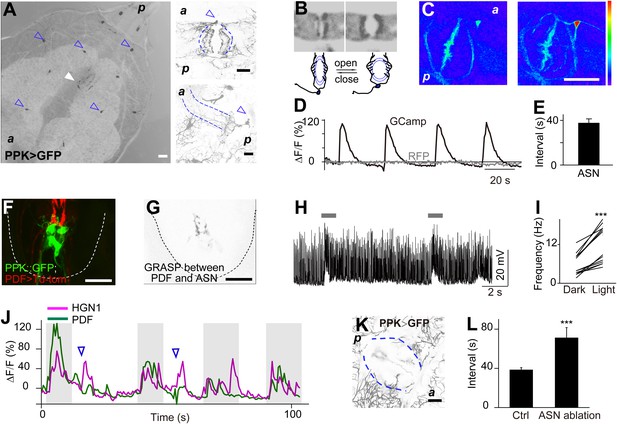
Sensory feedback from anus sensory neuron to the VNC motor neurons.
(A) Anus sensory neuron's (ASN) location and morphology. Left panel: filled arrowhead indicates the location of the ASN; open arrowheads indicate other PPK-Gal4 neurons. Upper right panel: arrowhead: cell body; lower right panel: lateral view of the dendritic extension along the anus sphincter. Dashed line indicating the border of the intestine (scale bar: 50 µm). a: anterior; p: posterior. (B) Images and schematic drawing of the anus opening, showing the stretching of the ASN dendrites. (C) Ca2+ elevates in ASN when anus opens. Left panel: resting state when the anus is closed; right panel: anus is open and the dendrites are stretched. (Color range: 1–256. Scale bar: 50 µm). (D) Plot of the Ca2+ intensity of the cell body region. Black trace: GCaMP5 signal; grey line: tomato signal as control in the same neuron. (E) Peak-to-peak interval of ASN Ca2+ activity (error bar: S.E.M., n = 7). (F) Dual labeling of PPK and PDF neurons. Red: PPK neuron axon terminals (PPK–GFP); green: PDF neuron cell bodies and dendrites (PDF-Gal4 > td-Tomato) (scale bar: 20 µm). (G) GRASP signals between ANS/PDF neurons (scale bar: 20 µm). (H) Increase of EJPs in the anus sphincter muscles when the ASN carrying ChR2 is activated by blue light (indicated by grey bars). (I) Paired plots of the EJPs frequency in dark and light condition (n = 14, ***p < 0.001, paired t test). (J) Direct ASN stimulation triggered PDF/HGN1 neurons' activity (arrow head); grey bars indicate spontaneous oscillations of PDF/HGN1 neurons. (K) Laser ablation of ASN eliminated the single neuron (ASN) while other PPK neurons remained intact. Larval genotype: HGN1-Gal4; UAS-GCaMP5/PPK-tdTomato. (L) ASN ablation increased HGN1 neurons' activity interval.
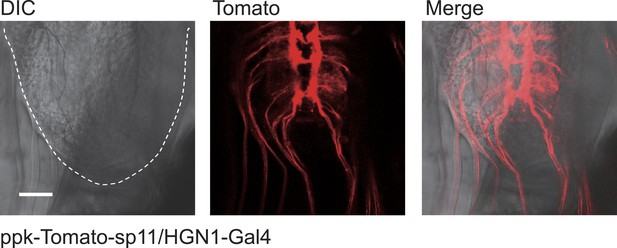
Single component of split-GFP did not show fluorescence.
https://doi.org/10.7554/eLife.03293.018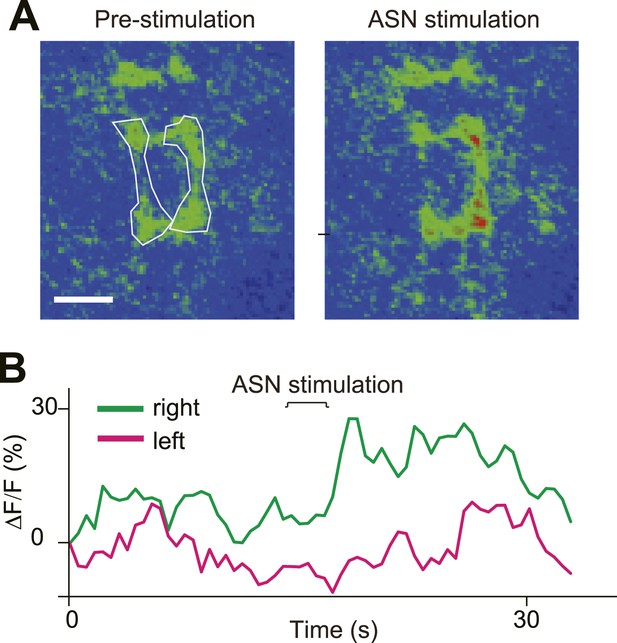
Direct ASN stimulation triggered asymmetric activation of PPK neurons' axon terminals.
(A) GCaMP intensity of ASN axon terminals before and after ASN (labelled with PPK > GCaMP) stimulation with a probe. Dashed lines outline the ROI areas. (B) GCaMP intensity of ROIs in (A) over time showing asymmetric Ca2+ elevation of ASN axon terminals.
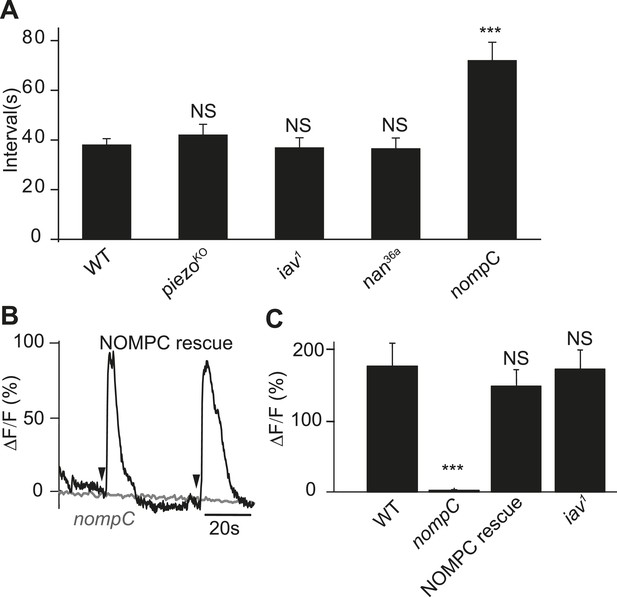
NOMPC is required for the mechanotransduction of ASN.
(A) nompC but not iav, nan, and piezo mutant affects the defecation cycle of the larvae (error bar: S.E.M., n = 6, 7, 7, and 6. ***p < 0.001, one-way ANOVA). (B) Ca2+ activity in the ASN responding to anus opening in nompC null mutant and NOMPC rescue larvae. Arrowheads indicate the anus opening for the rescue larva. (C) Group data of the Ca2+ response of ASN (error bar: S.E.M., n = 7, 10, 9, and 9. ***p < 0.001, one-way ANOVA).
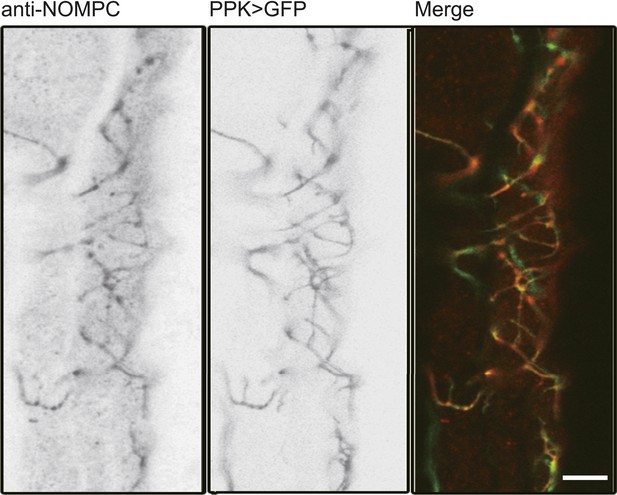
NOMPC expression over the ASN revealed by NOMPC staining.
The ASN was labelled by PPK-Gal4 driven GFP (scale bar: 10 µm).
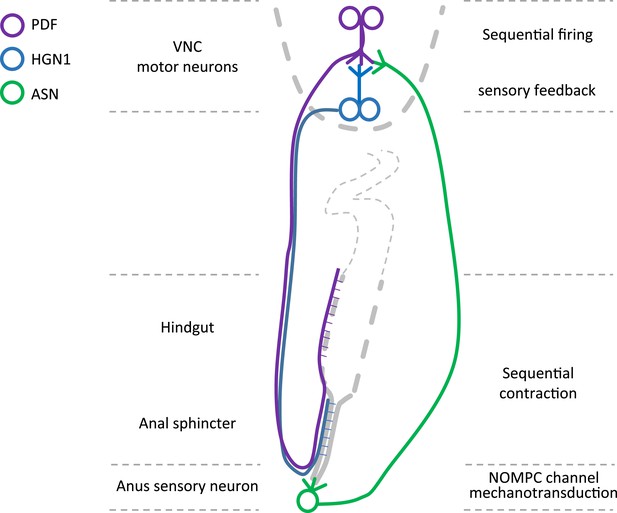
Graphic summary of the motor neurons and a mechanosensitive sensory neuron in the defecation circuitry.
https://doi.org/10.7554/eLife.03293.027Videos
Defecation behavior visualized with dyed feces.
A larva was placed lateral side up on a slide. The animal was fed with dyed food and its feces can be seen when moving along the intestine.
Light-induced defecation via activation of ChR2 in HGN1 neuron.
A larva was placed ventral side up on a slide. The defecation could be observed shortly after blue light illumination (as visualized by the auto-florescence of the internal organs).
Rhythmic activity of HGN1 motor neurons.
A larva was placed ventral side up. GCaMP5 was driven by HGN1-Gal4 to monitor the neuronal activity. The video is at 6× speed.
3-D reconstructions of PDF and HGN1 neurons in the VNC.
PDF neurons are labelled with PDF-GFP. HGN1 neurons are labelled with HGN1-Gal4 driven tdTomato. The Z-stack images were taken with 2-µm optical slice and projected along the Y-axis to get the stereo visualization of the structures.
Dual imaging of PDF and HGN1 neurons.
The larva was placed lateral side up to visualize both groups of neurons. The neurons expressed GCaMP5 under the drive of PDF and HGN1 Gal4s. Left side: ventral; right side: dorsal. A Ca2+ signal propagates from PDF neurons to HGN1 neurons.
ASN's response to anus opening in a wild-type larva.
The ASN was labelled with PPK-Gal4 driven GCaMP5. A dramatic increase of Ca2+ over dendrites and soma was observed when the anus opened.
Asymmetric activity of ASN axon projection in the VNC.
The sensory neuron axons were labelled with PPK-Gal4 driven GCaMP5. Note the last segment exhibited intense activity increase and the signal on the right side was stronger that the left side, resulted from the asymmetric projection of the ASN.
nompC mutant eliminated ASN's response to anus opening.
The ASN in the nompC mutant stayed quiescent even when it received a similar intensity of stretch. The genotype of the larva is: nompC1/nompC3; NOMPC-Gal4/UAS-GCaMP5, UAS-mCherry.
NOMPC channel rescued the nompC mutant phenotype in ASN.
A dramatic Ca2+ response could be detected when the wild-type NOMPC was expressed in the ASN with a nompC null background. The genotype of the larva is: nompC1/nompC3; NOMPC-Gal4, UAS-NOMPC/UAS-GCaMP5, UAS-mCherry.
IAV is not required for the ASN's stretch sensing.
The ASN of iav1 mutant larva exhibited similar response pattern to anus opening compared with wild-type larvae. The genotype of the larva is: iav1/y; NOMPC-Gal4/UAS-GCaMP5, UAS-mCherry.