Metagenomic chromosome conformation capture (meta3C) unveils the diversity of chromosome organization in microorganisms
Figures
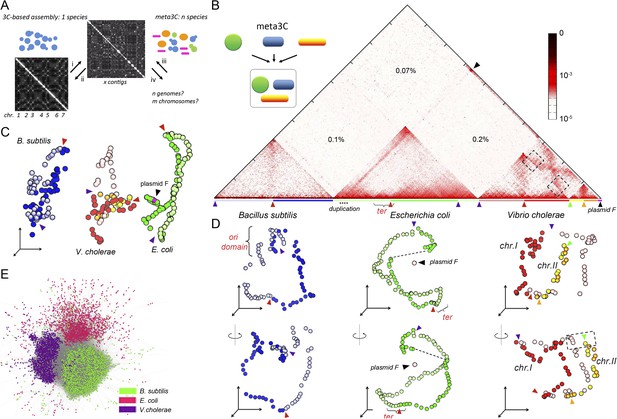
meta3C experiment on a controlled mix of bacterial species.
(A) Schematic representation of the principle of a meta3C experiment. For a single species, one can (i) generate a genome-wide contact map but also (ii) use the genomic contact data to reorder the contact matrix of a poorly assembled genome (top) in order to scaffold its contigs into a more likely structure (bottom left). For a mixture of species, one can similarly generate a contact map directly from the mix (iii) and then use it to characterize the genomes of the species contained in the mixture (iv). (B) Chromosomal contact map of a mixture of three bacteria. The darker the shade in the matrix, the higher the contact frequency (color scale in log). Blue and green arrowheads: origins of replication of the chromosomes. Red and orange arrowheads: termination of replication. Each chromosome arm is represented with a color code. The percentages of interactions that occurred between different species are indicated within the matrix. We detected frequent interactions between a F plasmid sequence (black arrowhead) and a large duplicated region of the E. coli chromosome (dotted line). (C) 3D reconstruction of the entire contact matrix. Each replichore of the four chromosomes is represented with a different color, with the colored arrowheads indicating Ori positions. Black arrowhead: F’ plasmid and E. coli chromosome when contacts with the duplication are taken into account; see Figure 1—figure supplement 2 for more details. (D) Two different views of the 3D reconstruction of each of the three bacteria taken individually. Ter and Ori are indicated with colored arrowheads (see above). E. coli and B. subtilis domains are also shown. The F’ plasmid is positioned according to its 3D contacts with the E. coli genome, without taking into account the duplication (dotted line within the E. coli genome). (E) Networks of interactions between the different contigs for the mix of three bacteria represented with a force-directed graph-drawing algorithm. Each node represents a contig from the de novo assembly. Each link in gray represents at least one 3C contact. Each color corresponds to one community detected by the Louvain algorithm.
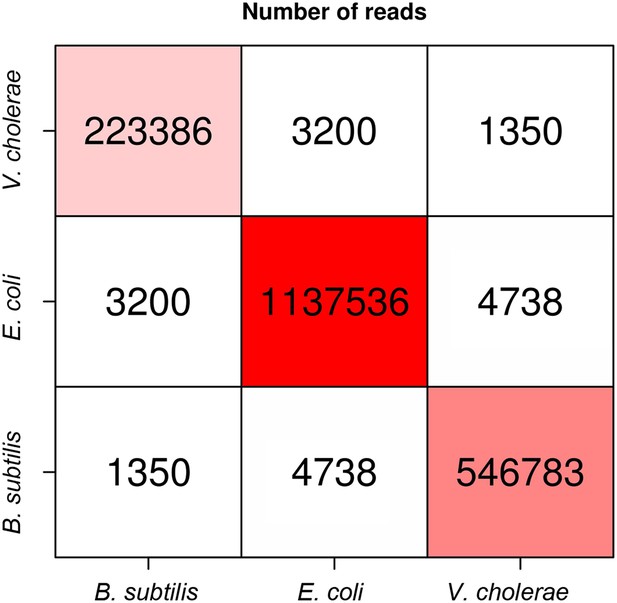
Numbers of intra-specific and inter-specific (chimeric) pairs of reads from the meta3C experiment performed on the bacterial mix.
https://doi.org/10.7554/eLife.03318.004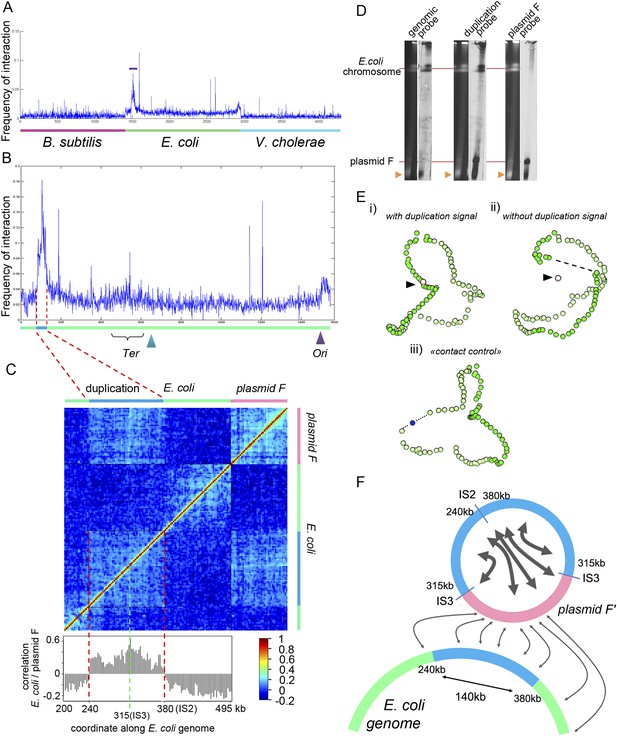
Analysis of the organization of the F’ plasmid in the E. coli strain used in this study.
(A) Frequencies of interaction between the F’ plasmid and the three bacterial genomes. (B) Contact frequencies between the F’ plasmid and the E. coli genome, normalized by the read coverage to take into account the replication. The dotted region encompassing a duplication with frequent contacts with the F’ plasmid corresponds to the region of interest analyzed in C. (C) Correlation analysis of the contact frequency between the F’ plasmid (in pink) and the duplicated region (in blue) along the E. coli genome (in green). A strong shift in the correlation score occurred for a region indicated with the red dotted lines, indicating that the plasmid F’ is in frequent contact with this region but not beyond it (and therefore must be carrying one copy of the duplicated region). This analysis allowed to position duplication breakpoints at coordinates ∼240,000:380,000 along the chromosome. (D) PFGE analysis of the E. coli genome and corresponding Southern blots hybridized with probes from the genome (duplicated and non-duplicated region) and from the F’ plasmid (see Table 2 for the coordinates of the probe). Orange triangle: migration front of the degraded DNAs of both E. coli genome and plasmid. (E) 3D reconstruction of genome-wide contact maps of the E. coli genome when (i) the duplicated region is considered as a single copy region of E. coli genome, (ii) the duplicated region is removed from the analysis, but not the plasmid F’, and (iii) a region of a size similar to the duplicated region is removed from the analysis and replaced with a small DNA segment of the same size as the F’ plasmid. (F) Schematic representation of the contacts between the F plasmid sequence (pink segment) and duplicated segments (blue) of the E. coli genome (green) that accounts for all the observations in panels A–E.
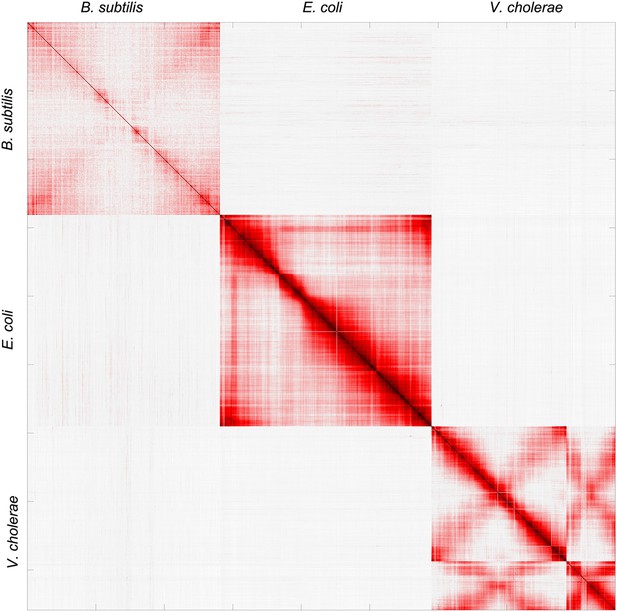
Pearson correlation matrix of the meta3C bacterial experiment.
https://doi.org/10.7554/eLife.03318.006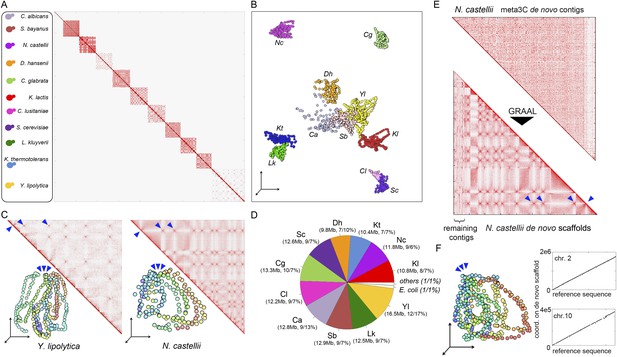
meta3C experiment on a controlled mix of yeasts species.
(A) meta3C contact map of the mix of eleven species. (B) 2D projection of the 3D reconstruction of the entire meta3C contact matrix. Each genome occupies an isolated position in space (the 2D projection induces a visual overlap for some species). The color code is the same as for the schematic yeasts on the left panel in (A). (C) Close-up on the contact maps of three species, with the 3D representation of the matrix in vis-à-vis. (D) Quantification of the assembly performed using meta3C reads. The first number indicates the amount of total DNA in the community. The two numbers that follow indicates the proportion of the contigs of each community in regards to the total assembly (% of total kb, % of total number of contigs). (E) Top: contact map of the contigs present within the community containing mostly sequences from N. castellii. The bottom contact map corresponds to the maps recovered following the GRAAL scaffolding. 11 large scaffolds were retrieved, in near-perfect agreement with the known number of chromosomes of this species. Blue triangles: inter-scaffold signal corresponding most likely to the 10 centromeric interactions (Marie-Nelly et al., 2014b). (F) Corresponding 3D structure of the N. castellii de novo meta3C assembly combined with GRAAL processing. The collinearity between two of the resulting superscaffolds and their corresponding reference chromosome sequences is represented in the right panels.
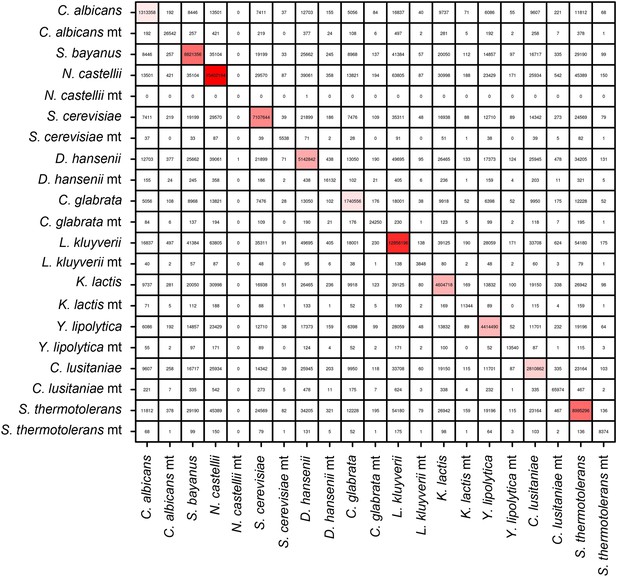
Number of intra-specific and inter-specific (chimeric) pairs of reads in the meta3C contact map.
Mitochondrial genomes behaved as separate entities.
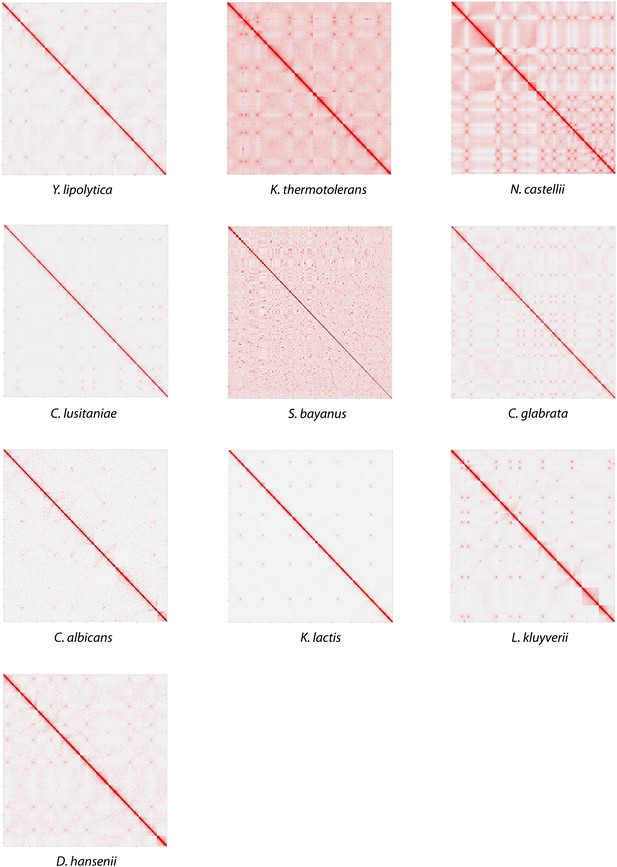
Contact matrices of the genomes of Y. lipolytica, K. thermotolerans, N. castellii, C. lusitaniae, S. bayanus, C. glabrata, C. albicans, K. lactis, L. kluyveri, and D. hansenii.
https://doi.org/10.7554/eLife.03318.015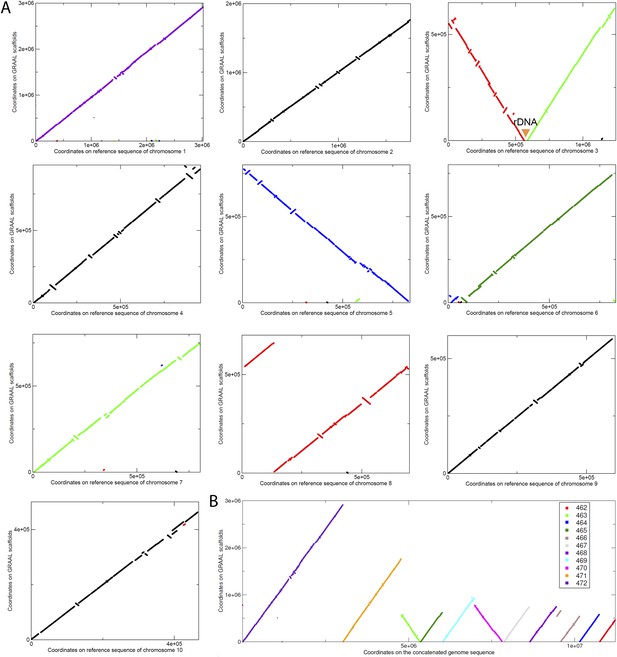
Scaffolding using GRAAL of N. castellii meta3C contigs.
(A) Comparison between the scaffolds generated by GRAAL and the reference sequences of the N. castellii chromosomes: y-axis: coordinates along the new scaffolds. x-axis: coordinates along the reference chromosome. The position of the rDNA cluster is indicated. (B) The eleven largest scaffolds are aligned against the concatenated reference genome, covering 94.5% of the total sequence.
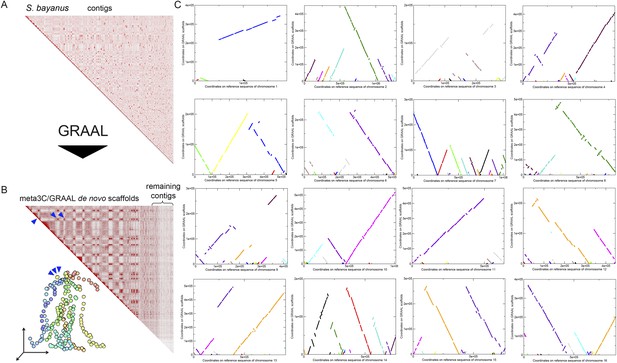
Scaffolding using GRAAL of S. bayanus meta3C contigs.
(A) Contact map obtained from a draft genome assembly of S. bayanus. (B) Contact map and corresponding 3D structure of the S. bayanus de novo meta3C assembly combined with GRAAL processing. Blue triangles: inter-scaffold signal corresponding most likely to the 10 centromeric interactions. (C) Comparison between the scaffolds generated by GRAAL and the reference sequences of S. bayanus chromosomes. The main scaffold is indicated in bold, whereas the other ones correspond to other scaffolds and are shown here for information only.
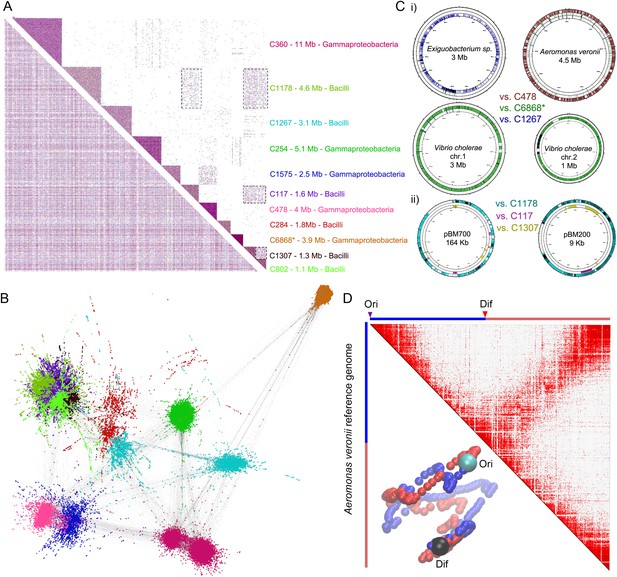
meta3C analysis of a complex environmental sample.
(A) meta3C contact map of the largest 11 communities of contigs in the matrix before (bottom left) and after (upper right) clustering. Each square corresponds to a community grouping contigs that exhibit significantly more contact with each other than with other communities. For each community, an index, the sum of the sequences, and candidate classes are indicated on the right side of the matrix. Dotted square underlines inter-community contact enrichments. C6868*: V. cholerae. (B) Illustration of the interactions between the 11 largest communities of contigs using a force-directed graph drawing algorithm Force Atlas 2 (Jacomy et al., 2014). Each node corresponds to a contig (or a chunk of a contig) and each link represents at least one meta3C interaction. The colors correspond to the communities identified by the Louvain algorithm and described in A. (C) i: For three communities, the contigs were mapped against the closest reference genomes identified (Exiguobacterium sp. AT1b, A. veronii B565, V. cholerae N16961, plasmids pBM700 and pBM200). To illustrate the specificity of the approach, each community was mapped against each of those genomes (the color code is the same as in A and the order from the outer circle to the inner circle is indicated in the middle). ii: Similarly, the three Bacilli communities highlighted in color were mapped against two plasmid sequences. (D) Genomic contact map of an unknown species (most likely A. veronii or a close relative) generated by mapping the reads present in the community 478 against the genome of A. veronii (bin size = 10 kb). The corresponding 3D structure is indicated next to the contact map.
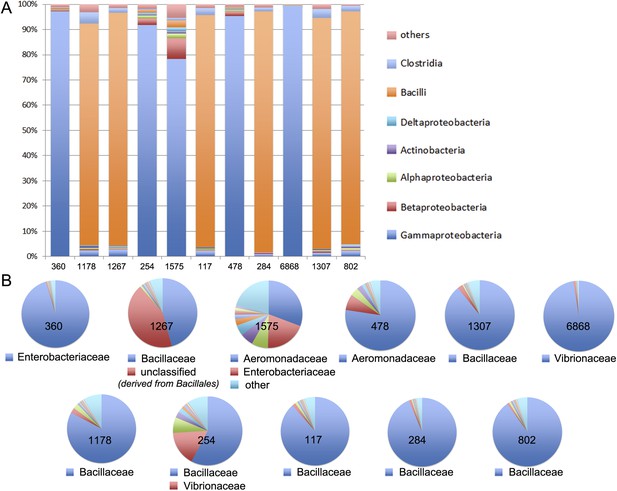
MG-RAST analysis of the 11 largest meta3C communities.
The similarity between the sequences present in these communities and those of known species was to annotate them. (A) Homogeneity regarding class-level annotations; (B) homogeneity regarding family-level taxonomy.
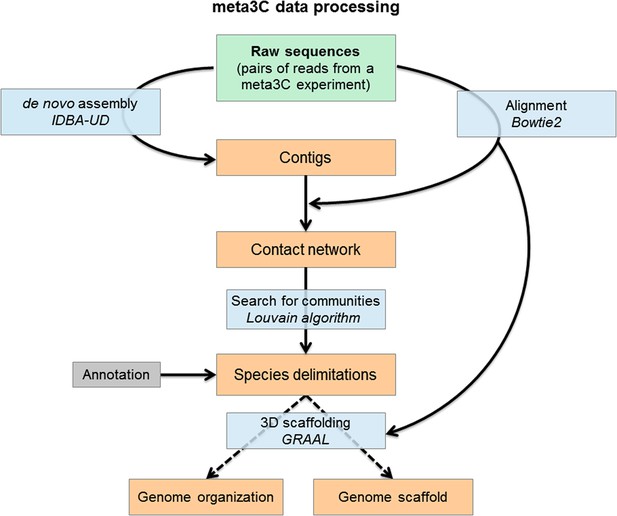
Flowchart representing the computational analysis steps of a meta3C experiment.
First, the reads from the sequenced meta3C library are assembled into contigs. The meta3C contact information between the contigs is then used to generate a network of the contacts of all contigs against each other. This contact network is searched for so-called ‘communities’ (by analogy with social network analysis) using the Louvain algorithm. The significant communities can be annotated by NCBI and correspond principally to sequences from individual species. Ultimately, the contact information can be used to reorder the DNA segments within each community and thus generate the 3D contact map of the corresponding species. In this process contigs can be reassembled by software such as GRAAL, thereby decreasing the percentage of chimeric fragments in the assembly and improving its continuity. DNA segments originating from other species are put aside automatically during this process given their lack of 3C contacts with the rest of the genome under reassembly.
Videos
3D reconstruction of the bacterial meta3C contact matrix.
https://doi.org/10.7554/eLife.03318.0073D reconstruction of the E. coli genome with a plasmid F’ carrying a 140 kb segmental duplication.
The contacts made by the duplicated region are taken into account. Because of the frequent contacts of one copy of this region with the chromosome and the other copy with the plasmid and of the impossibility to distinguish those two copies, the plasmid is now artificially dragged into the vicinity of the E. coli chromosome.
3D reconstruction of the E. coli genome with a plasmid F’.
The contacts made by the 140 kb duplication are not taken into account, so the position of the plasmid depends only on its contacts with the rest of the genome.
3D reconstruction of the B. subtilis genome.
https://doi.org/10.7554/eLife.03318.0103D reconstruction of the V. cholerae genome.
https://doi.org/10.7554/eLife.03318.0113D reconstruction of the V. cholerae genome.
The color scale now represents the read coverage along the genome, hence displaying the differential replication timing of the two chromosomes, from early (over-covered) replicated regions (in blue) to terminal regions (in red).
3D reconstruction of the 11 yeasts meta3C contact matrix.
https://doi.org/10.7554/eLife.03318.0183D reconstruction of the Y. lipolytica genome.
https://doi.org/10.7554/eLife.03318.0193D reconstruction of the N. castellii genome.
https://doi.org/10.7554/eLife.03318.0203D reconstruction of the N. castellii genome after meta3C de novo assembly and GRAAL scaffolding.
https://doi.org/10.7554/eLife.03318.0213D reconstruction of the S. bayanus genome after meta3C de novo assembly and GRAAL scaffolding.
https://doi.org/10.7554/eLife.03318.0223D reconstruction of a genome closely related to A. veronii.
Blue and black beads: Ter and Ori regions, according to the reference genome of A. veronii.
Tables
List of the custom-made Illumina adapters used in this study
Oligos | Sequence | Library |
---|---|---|
MM70 | GTANNNNNNAGATCGGAAGAGCGGTTCAGCAGGAATGCCGAG | Mix of 11 yeasts |
MM71 | ACACTCTTTCCCTACACGACGCTCTTCCGATCTNNNNNNTACT | |
MM76 | CAGNNNNNNAGATCGGAAGAGCGGTTCAGCAGGAATGCCGAG | Mix of 3 bacteria |
MM77 | ACACTCTTTCCCTACACGACGCTCTTCCGATCTNNNNNNCTGT | |
MM70 | GTANNNNNNAGATCGGAAGAGCGGTTCAGCAGGAATGCCGAG | River sediment |
MM71 | ACACTCTTTCCCTACACGACGCTCTTCCGATCTNNNNNNTACT |
List of the oligonucleotides used to PCR amplify probes for the Southern blot of the PFGE (Figure 1—figure supplement 2)
Oligos | Sequence | Coordinates | Probe |
---|---|---|---|
Meta1 | CATCCCGTGAGAAATAATGGTCG | 2,256,273 (MG1655) | Genomic probe |
Meta2 | TGTGCATCCCGTCACAAATTC | 2,257,545 (MG1655) | |
Meta3 | TTGAGCTTATCAAAGTCGTCGGAG | 323,607 (MG1655) | Duplication probe |
Meta4 | TGATGTGAACTAACGCAGGAAC | 324,796 (MG1655) | |
Meta5 | TTTACCTCTGATACTGGCTCTGG | 79,024 (plasmid F) | Plasmid F probe |
Meta6 | ACGTGGCATATTCATGCAGAC | 80,159 (plasmid F) |