Selection of chromosomal DNA libraries using a multiplex CRISPR system
Figures
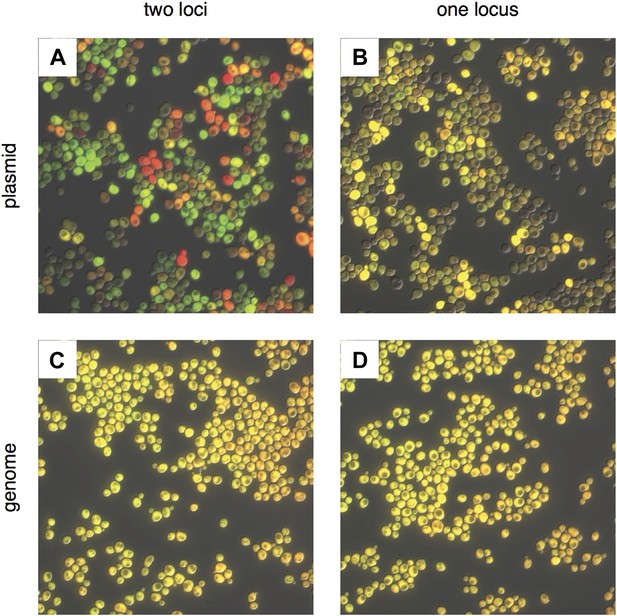
Comparing noise in plasmid and genomic expression of two fluorescent proteins.
Proteins mRuby2 (false-colored red) and Venus (false-colored green) were expressed in the same cell with a strong, PTDH3 promoter. (A) Fluorescent proteins expressed on two separate CEN plasmids; (B) in tandem on a single CEN plasmid; (C) integrated into two different loci (LEU2 and URA3) in the S. cerevisiae genome; and (D) integrated in tandem at a single locus (URA3) in the genome. Each image was auto-exposed for both red and green channels, with yellow showing co-expression of mRuby2 and Venus. The variability in the relative expression of the two fluorescent proteins is reduced by moving from two plasmids to one, and the variability in total expression is reduced by moving to the genome. The difference between integrating into one or two loci in the genome is minimal.
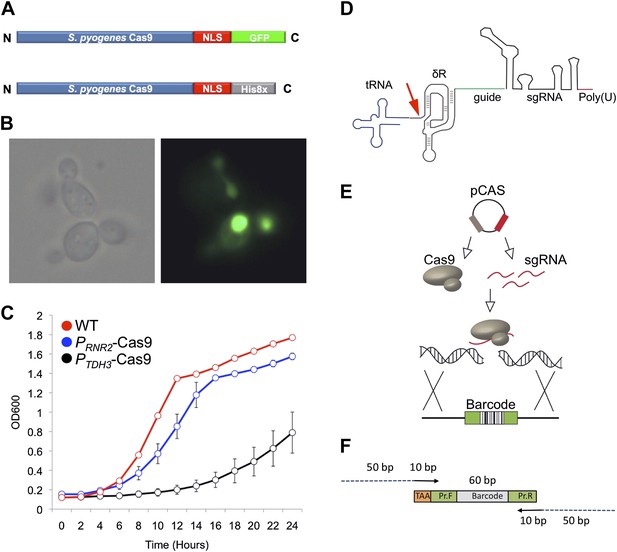
The engineered CRISPRm platform.
(A) Cas9 construct used for nuclear localization experiments. The S. pyogenes Cas9 protein was tagged with a C-terminal nuclear localization motif and a green fluorescent protein (GFP) (green). For genome editing experiments, the S. pyogenes Cas9 protein was tagged with a C-terminal nuclear localization motif and a Histidine affinity tag (gray). (B) Cellular localization of Cas9-GFP in exponentially growing S. cerevisiae cells. The Cas9-GFP protein was expressed from the TDH3 promoter in this experiment. Left, bright field image; right fluorescence microscopy. (C) Growth profiles of yeast expressing Cas9 from a strong promoter PTDH3 (black) or a weaker promoter PRNR2 (blue) relative to wild type (red). (D) The mature sgRNA contains a 5′ hepatitis delta virus (HDV) ribozyme (δR, brown), 20mer target sequence (green), sgRNA (black) and RNA polymerase III terminator (red). The RNA polymerase III promoter tRNA (blue) is catalytically removed by the HDV ribozyme (red arrow). (E) Schematic of yeast Cas9 targeting. Cas9 and sgRNA are expressed from a single plasmid, form a complex, and cleave targeted genomic DNA, which is repaired using a barcoded oligonucleotide. (F) The linear barcoded repair DNA molecule. Each repair DNA contains a 5′ TAA stop codon (gold), a forward primer sequence (green), a unique molecular barcode (gray), and a 3′ reverse primer (green) (Giaever et al., 2002). Barcodes are amplified using a forward primer that contains 50 bp of homology (blue) to the genome targeting site and a reverse primer that contains 50 bp of homology to the genome targeting site. The 50 bp of genomic targeting sequence are each 10 bp proximal to the PAM motif, resulting in a 20 nt deletion and barcode oligonucleotide integration.
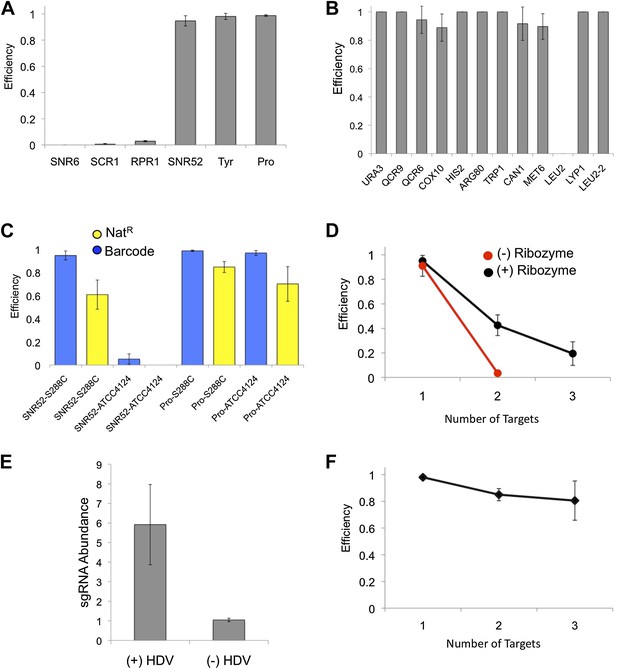
CRISPRm barcode insertion in yeast cells.
(A) Targeting efficiency at the URA3 locus in diploid S288C yeast using RNA polymerase III promoters (x axis) to drive the expression of the sgRNA. (B) Targeting efficiency measured across 11 loci in 10 genes in diploid cells of yeast strain S288C, using the tRNATyr promoter. (C) Single fragment barcode integration (blue) and three-fragment NatR cassette integration (yellow) efficiency in S288C diploid and ATCC4124 polyploid strains. For each experiment the promoter and strain are indicated as promoter-strain (e.g., SNR52-S288C). (D) Efficiency of multiplex insertion of barcoded DNA in diploid yeast cells with the 5′ HDV ribozyme (black) and without the 5′ HDV ribozyme (red). Triplex targeting without the 5′ HDV ribozyme was not tested. The tRNATyr promoter was used in these experiments. (E) The addition of a 5′ HDV ribozyme increases the intracellular levels of sgRNA by sixfold. The RT-qPCR experiments were carried out in biological triplicate, with the mean and standard deviation shown. (F) Efficiency of targeting one (URA3), two (URA3, LYP1) and three (URA3, LYP1 and COX10) in haploid S288C yeast cells. The tRNATyr promoter was used for sgRNA expression.
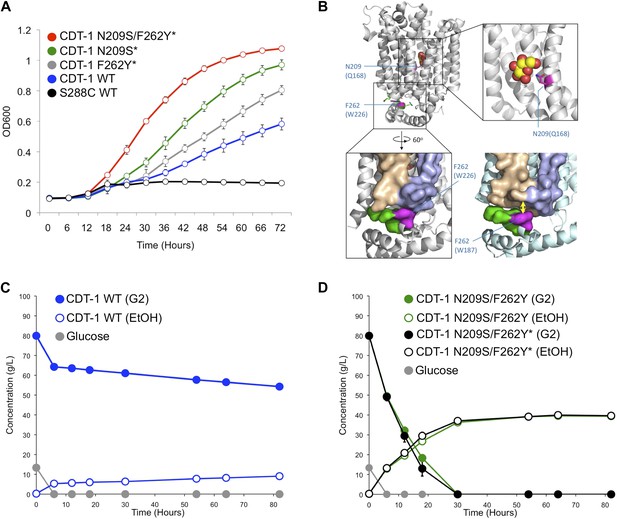
CRISPRm mediated insertion and evolution of chromosomal DNA libraries for in vivo protein engineering.
(A). Utilization of cellobiose in CRISPRm-engineered diploid yeast strains. S288C wild type cdt-1− (black), cdt-1 (blue), cdt-1Q45H/F262Y/F533Y (indicated as cdt-1F262Y* on the figure, gray), cdt-1N209S/I354N/S360P/T406S/W531L (indicated as cdt-1N209S* on the figure, green) and cdt-1N209S+Q45H/F262Y/F533Y (indicated as cdt-1N209S+F262Y* on the figure, red). (B) Location of mutations at conserved sites in the evolved CDT-1 transporter structure. The mutated residues are colored magenta in the top figure, and are mapped onto the E. coli XylE transporter in the outward-facing configuration bound to glucose (upper panels and lower left, PDB entry 4GBZ), and onto the S. epidermidis GlcP transporter in the inward-facing configuration (lower right, PDB entry 4LDS). Amino acids in parentheses are the sequences in XylE or GlcP. (C) Fermentation of cellobiose by wild-type cdt-1 expressed from a chromosomally-integrated copy in diploid S288C yeast. Cellobiose is indicated as G2, ethanol as EtOH. The rate of cellobiose consumption was 0.13 g L−1 hr−1. (D) Fermentation of cellobiose by mutant versions of cdt-1 expressed from chromosomally-integrated copies. Glucose consumption (gray) was identical in both strains. The rate of cellobiose consumption for both strains was over 2.0 g L−1 hr−1. In (C and D), values and error bars represent the means and standard deviations of three independent biological replicates.
Additional files
-
Supplementary file 1
(A) CRISPR-Cas screening results. (B) Potential off-target mutations identified by whole genome sequencing. (C) RNA polymerase III promoter sequences used to express sgRNAs. (D) Guide sequences used in this study.
- https://doi.org/10.7554/eLife.03703.007
-
Supplementary file 2
(A) pCAS—Tyrosine—sgRNA-LYP1. (B) pOR1.1. (C) sgRNA without HDV ribozyme. (D) sgRNA with HDV ribozyme.
- https://doi.org/10.7554/eLife.03703.008
-
Supplementary file 3
Custom perl script for Cas9 target identification in S288C genome.
- https://doi.org/10.7554/eLife.03703.009
-
Supplementary file 4
Custom R script for yeast genome analysis.
- https://doi.org/10.7554/eLife.03703.010