Neuron-wide RNA transport combines with netrin-mediated local translation to spatially regulate the synaptic proteome
Figures
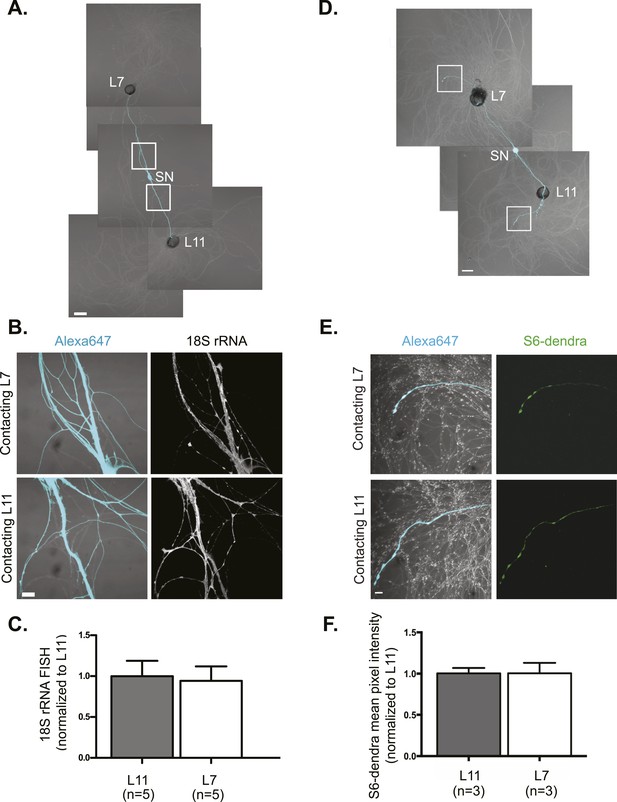
rRNA targets equally well to SN branches contacting target and non-target MNs.
A bifurcated SN was cultured with an L7 target MN and an L11 non-target MN (A) for 3 days. Alexa647 (cyan) was microinjected into the SN before imaging to visualize SN processes. Cultures were processed for Fluorescent In Situ Hybridization (FISH) for 18S rRNA (B). Areas in proximal SN neurites outlined with white squares in (A) were imaged at high magnification in (B); left panels show merged images of DIC and Alexa fluorescence, and right panels show FISH signals. The Alexa647 served as a volume control to analyze FISH RNA intensity. Group data show that 18S rRNA (C) is evenly distributed in neurites contacting L7 target and L11 non-target MNs. Parallel experiments showing FISH for 28S rRNA are shown in Figure 1—figure supplement 1. A bifurcated SN was cultured with an L7 target MN and an L11 non-target MN (D) for 1 day and a plasmid expressing ApS6 tagged with dendra2 was microinjected together with Alexa647 (cyan) into the SN. Live imaging (D) was performed on day 3; shown in (E) are high magnification images of DIC and Alexa fluorescence on the left and ApS6-dendra2 on the right. The Alexa647 served as a volume control to analyze FISH RNA intensity. Group data show that ApS6 (F) is evenly distributed in neurites contacting L7 target and L11 non-target MNs. Error bars represent SEM. None of the differences were significant as determined by a Student's paired t-test. Scale bar in (A and D) =100 μm; in (B and E) =20 μm.
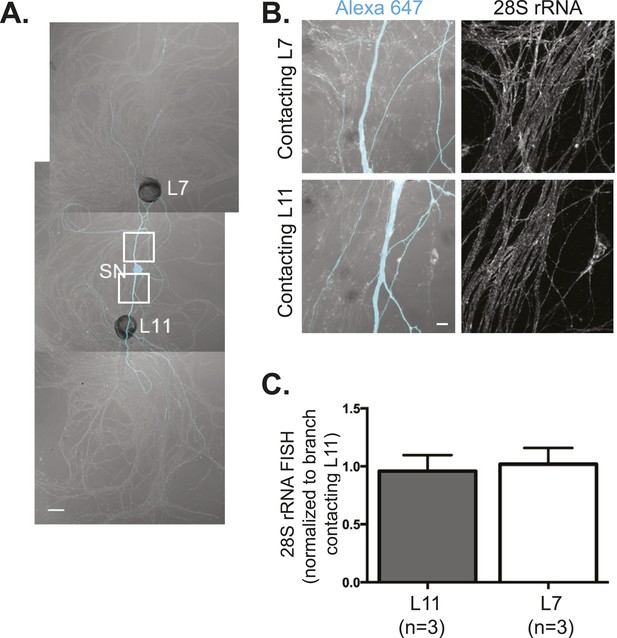
28S rRNA targets equally well to SN branches contacting target and non-target MNs.
A bifurcated SN was cultured with an L7 target MN and an L11 non-target MN (A) for 3 days. Alexa647 (cyan) was microinjected into the SN before imaging to visualize SN processes. Cultures were processed for Fluorescent In Situ Hybridization (FISH) for 28S rRNA (B). Areas in proximal neurites outlined with white squares in (A) were imaged at high magnification in (B); left panels show merged images of DIC and Alexa fluorescence, and right panels show FISH signals. The Alexa647 served as a volume control to analyze FISH RNA intensity. Group data show that 28S rRNA (C) is evenly distributed in neurites contacting L7 target and L11 non-target MNs. Scale bar in (A) =100 μm; in (B) =20 μm.
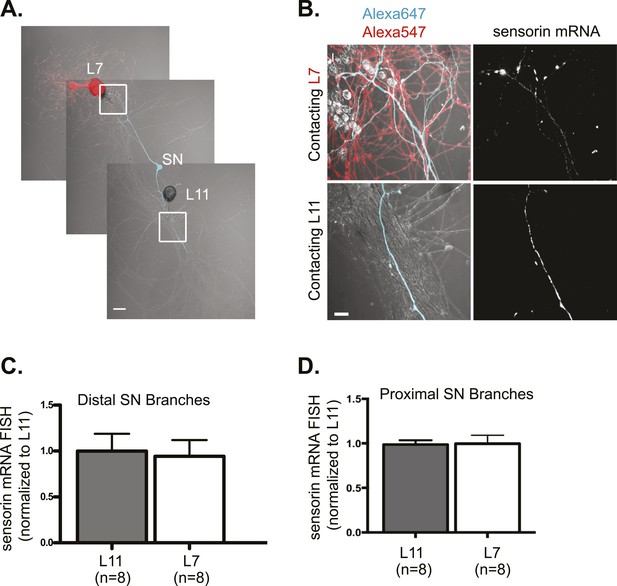
Sensorin mRNA targets equally well to SN branches contacting target and non-target MNs.
A bifurcated SN was cultured with an L7 target MN and a L11 non-target MN (A) for 3 days. Alexa647 (cyan) was microinjected into the SN before imaging to visualize SN processes; in (A), Alexa 546 (red) was also microinjected into the L7 MN before imaging to visualize the L7 MN processes. Cultures were processed for Fluorescent In Situ Hybridization (FISH) for sensorin. Areas outlined with white squares in (A) was imaged at high-magnification to visualize mRNA in distal (B) SN neurites contacting L7 and L11 MNs, respectively; left panels show merged images of DIC and Alexa fluorescence, and right panels show sensorin mRNA FISH signals. The Alexa647 served as a volume control to analyze FISH RNA intensity. Group data show that sensorin mRNA (C and D) is evenly distributed in proximal and distal SN neurites contacting L7 target and L11 non-target MNs. Error bars represent SEM. None of the differences were significant as determined by a Student's paired t-test. The pattern of sensorin mRNA distribution differs in distal branches contacting L7 and L11 MNs, as shown in Figure 2—figure supplement 1A, because sensorin mRNA concentrates at synapses (Lyles et al 2006). Scale bar in (A) =100 μm; in (B) =20 μm.
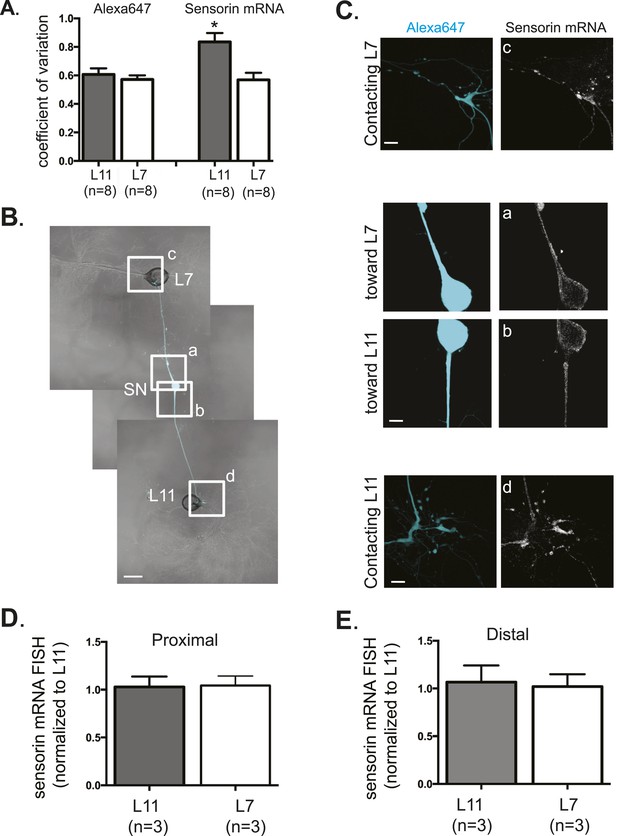
Sensorin mRNA distribution in DIV3 SN-MN cultures and localization in DIV1 SN-MN cultures.
The coefficient of variation for sensorin mRNA (A) indicates that the pattern of sensorin mRNA distribution is distinct in distal SN branches contacting L7 target MN, as previously described in Lyles et al. 2006. To analyze sensorin mRNA distribution at earlier time points, a bifurcated SN was cultured with two target MNs for 1 day (B). Alexa647 (cyan) was microinjected into the SN before imaging to visualize SN processes. Cultures were processed for Fluorescent In Situ Hybridization (FISH) for sensorin mRNA. Areas in proximal and distal (C) SN neurites outlined with lettered white squares in (B) were imaged at high magnification in (C); left panels show images of Alexa fluorescence in SN (cyan), and right panels show sensorin FISH signals. The Alexa647 served as a volume control to analyze FISH RNA intensity. Group data show that sensorin rRNA is evenly distributed in proximal (D) and distal (E) SN neurites contacting L7 target and L11 non-target MNs. Error bars represent SEM. None of the differences were significant as determined by a Student's paired t-test. Scale bar in (B) =100 μm; in (C) =20 μm.
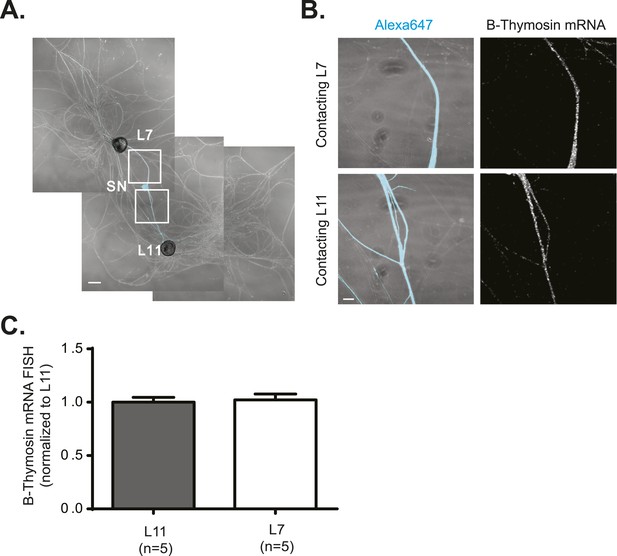
β-Thymosin mRNA localizes equally well to SN branches contacting target and non-target MNs.
A bifurcated SN was cultured with an L7 target MN and an L11 non-target MN (A) for 3 days. Alexa647 (cyan) was microinjected into the SN before imaging to visualize SN processes. Cultures were processed for Fluorescent In Situ Hybridization (FISH) for β-thymosin mRNA (B). Areas in proximal neurites outlined with white squares in (A) were imaged at high magnification in (B); left panels show merged images of DIC and Alexa fluorescence, and right panels show FISH signals. The Alexa647 served as a volume control to analyze FISH RNA intensity. Group data show that β-thymosin mRNA (C) is evenly distributed in neurites contacting L7 target and L11 non-target MNs. Scale bar in (A) =100 μm; in (B) =20 μm.
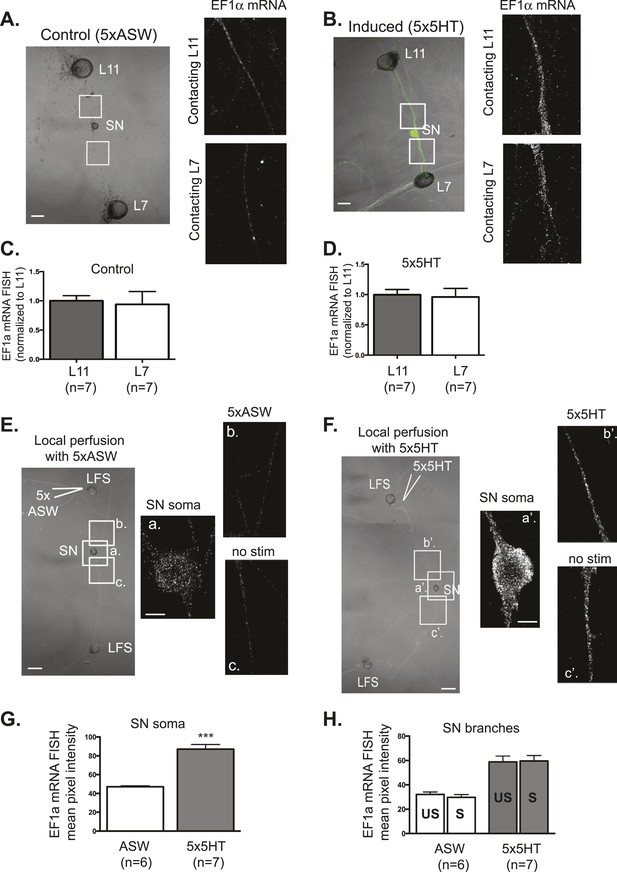
EF1α mRNA is induced by 5x5HT and is delivered throughout the neuronal arbor.
A bifurcated SN was cultured with an L7 target MN and an L11 non-target MN (A and B) for 3 days. Alexa647 (cyan) was microinjected into the SN before imaging to visualize SN processes. On day 3, cultures were stimulated with 5 bath applications of 5HT to induce long-term facilitation (LTF) (B), or mock stimulated with 5 bath applications of artificial seawater (ASW, the vehicle) as controls (A). Four hours later, cultures were processed for Fluorescent In Situ Hybridization (FISH) for EF1α mRNA. Areas in proximal SN neurites outlined with white squares in low magnification DIC image on the left were imaged at high magnification to visualize EF1α mRNA FISH signal. The increase in EF1α mRNA FISH signal in (B) as compared to (A) indicates that EF1α mRNA was induced by bath application of 5x5HT, as described in Giustetto et al (2003). Group data show that EF1α mRNA is evenly distributed between SN neurites contacting L7 target and L11 non-target MNs both in control cultures (C), and in cultures undergoing 5HT-induced LTF (D). A bifurcated SN was cultured with two LFS motor neurons (E and F) for 3 days. On day 3, local perfusion of five pulses of 5HT was performed to induce branch-specific LTF (F), as described in Martin et al (1997); in control cultures, synapses were mock stimulated with five pulses of ASW (E). Four hours later, cultures were processed for Fluorescent In Situ Hybridization (FISH) for EF1α mRNA. Areas in soma and proximal SN neurites outlined with lettered white squares in low magnification DIC image on the left were imaged at high magnification to visualize EF1α mRNA FISH signal. The increase in EF1α mRNA FISH signal in (F) as compared to (E) indicates that EF1α mRNA was induced by local stimulation with 5x5HT. This increase is quantified in SN soma and in proximal neurites in (G) and (H). As shown in (H), basally expressed and local stimulation-induced EF1α mRNA was evenly distributed between 5HT-stimulated (S)- and unstimulated (US)-SN branches. ***p < 0.001, Student's unpaired t-test. Differences in FISH signal between mock (ASW)-stimulated and -unstimulated branches (E) and 5HT-stimulated and -unstimulated branches (F) were not significant, Student's paired t-test (H). Scale bar in (A, B, E and F) =100 μm; in (E(a) and F(a′)) =20 μm.
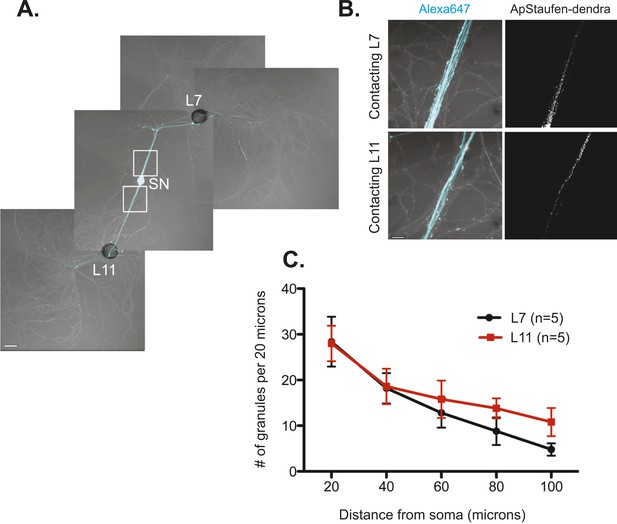
The RNA binding protein Aplysia Staufen targets equally well to SN neurites contacting L7 target and L11 non-target MNs.
(A) C-terminally tagged dendra2-tagged Aplysia Staufen (ApStaufen) was microinjected together with Alexafluor 647 (cyan) into a cultured bifurcated SN contacting an L7 target MN and an L11 non-target MN at 1DIV. Staufen granules in SN neurites were imaged 48 hr later. Representative high magnification images of areas denoted by white squares in (A) are shown in (B). Left panels show merged DIC/Alexa fluor images, and right panels show ApStaufen-dendra2 granules images. (C) Group data reveal that the number of ApStaufen-dendra2 granules per 20 μm was the same in neurites contacting target L7 MNs (black, closed circle) and non-target L11 MNs (red, square), Student's paired t-test at each time point. Error bars represent SEM. Scale bar in (A) =100 μm; in B =20 μm.
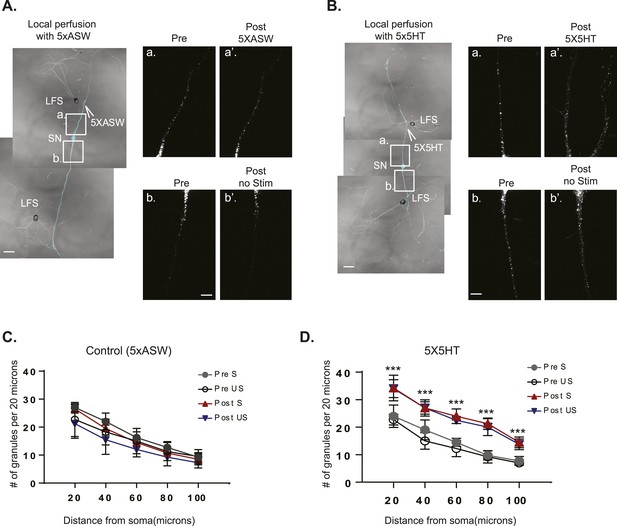
The RNA binding protein Aplysia Staufen targets equally well to stimulated- and unstimulated-SN processes after local perfusion.
(A) and (B) bifurcated sensory neurons (SNs) were cultured with two target LFS motor neurons (MNs). C-terminally tagged dendra2-tagged Aplysia Staufen (ApStaufen) was expressed in the SN, which was also labeled by microinjection with Alexa647 (cyan). Branch-specific long-term facilitation (LTF) was induced by local perfusion of 5X5HT (B); control cultures were locally perfused with 5 pulses of artificial seawater (ASW) (A). Images were acquired before and 24 hr after local perfusion. The upper panels in A (a and a′) and B (a and a′) show ApStaufen-granules in SN branches perfused with 5HT or ASW; the lower panels in A (b and b′) and B (b and b′) show ApStaufen-containing granules in SN branches that were not perfused. Local perfusion with 5X5HT significantly increased the number of ApStaufen-containing granules, to an equal extent, in stimulated- and unstimulated-SN branches (D). ***p < 0.001, Student′s unpaired t-test. Mock stimulation with 5XASW did not increase the number or distribution of ApStaufen-containing granules(C). Student's paired t-test at each time point. Error bars represent SEM. Scale bar in (A and B) =100 μm; in A(b) and B(b) =20 μm.
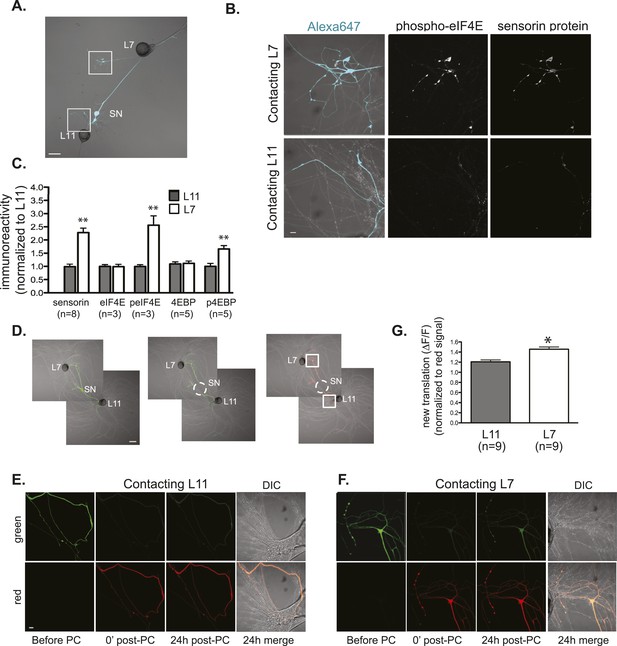
Local translation is greater in SN neurites contacting L7 target MNs than in neurites contacting L11 non-target MNs.
(A) A bifurcated SN was cultured with an L7 target MN and an L11 non-target MN for 3 days. Alexa647 was microinjected into the SN and served as a volume filling control for analysis of immunocytochemistry (ICC). To monitor local translation, we performed ICC for phosphorylated eIF4E (phospho-eIF4E), phosphorylated 4EBP, and sensorin protein. Representative high magnification images of areas denoted by white squares in (A) are shown in (B), with merged DIC/Alexa fluor in the left panel, phospho-eIF4E ICC in the middle panel, and sensorin ICC in the right panel. Group data in (C) reveal that phospho-eIF4E, phospho-4EBP, and sensorin protein concentrations are significantly greater in neurites contacting L7 target MNs than in neurites contacting L11 non-target MNs. As shown in Figure 5—figure supplement 1, total eIF4E and total 4EBP protein were distributed equally well in neurites contacting L7 target and L11 non-target MNs. To directly image local translation, we expressed a translational reporter consisting of sensorin mRNA fused to the photoconvertible fluorescent protein dendra2 (as described in Wang et al. (2009)), removed the SN soma (dotted circle in middle panel of D), and 12–18 hr later photoconverted the dendra2 signal from green to red (right panel of D). Shown in (E, neurite contacting L11) and (F, neurite contacting L7) are high magnification images of regions marked by white squares in (D), including images before photoconversion (pre), right after PC (post), 24 hr later (24 hr post). The top panels show the green channel and the bottom show the red channel (which was used as volume control for quantification of the green signal). Increased green signal represents newly translated reporter. Group data (G) show that there is significantly more translation, measured as ΔF/F, in neurites contacting L7 target MNs than in neurites contacting L11 non-target MNs. Error bars represent SEM. **p < 0.05, unpaired t-test. Scale bars in (A) and (E) =100 μm; in (C), (D), and (F) =10 μm.
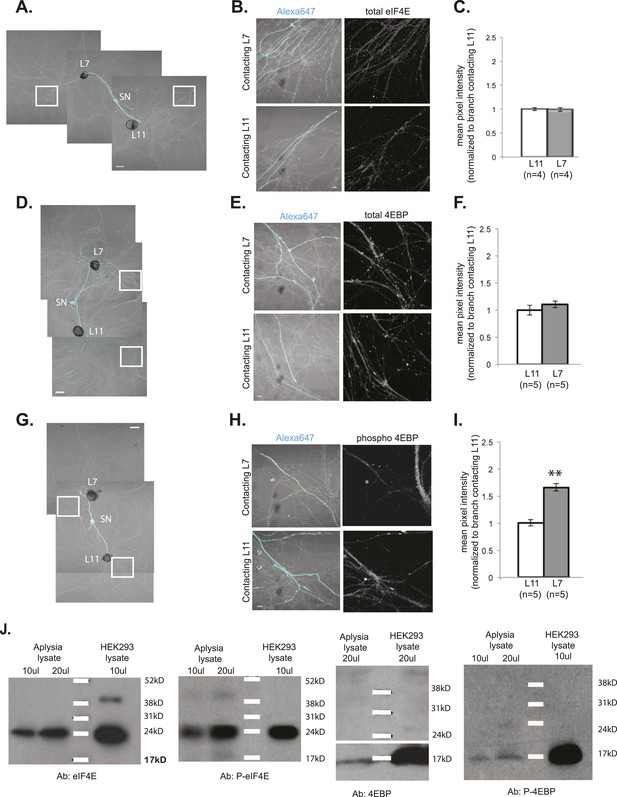
Local translation is enriched in SN branches contacting L7 MN.
A bifurcated SN was cultured with an L7 target MN and an L11 non-target MN (photomicrographs in A, D, and G) for 3 days. Alexa dye 647 (cyan) was microinjected into the SN before imaging (A, D, and G). Cultured neurons were processed for ICC for eIF4E (B; total) and 4EBP (E; total, H; phosphorylated); representative high magnification images of areas denoted by white squares in (A, D, and G) are shown in (B, E, and H), with merged DIC/Alexa fluor in the left panel, total eIF4E (B), 4EBP (E), and phospho-4EBP (H) on the right. Group data in (C, F, and I) reveal that phospho-4EBP accumulation was significantly greater in neurites contacting L7 target MNs than in neurites contacting L11 non-target MNs. However, total eIF4E (C) and total 4EBP (F) protein were distributed equally well in neurites contacting L7 target and L11 non-target MNs. Error bars represent SEM. *p < 0.05, paired t-test. Scale bars in (A), (D), and (G) =100 μm; in (B), (E), and (H) =10 μm. To test that the antibodies specifically recognized the Aplysia homologs, we performed immunoblots of Aplysia CNS lysates and of HEK cell lysate with the eIF4E, phospho-eIF4E (P-eIF4E), 4EBP, and phospho-4EBP (P-4EBP) antibodies (J).
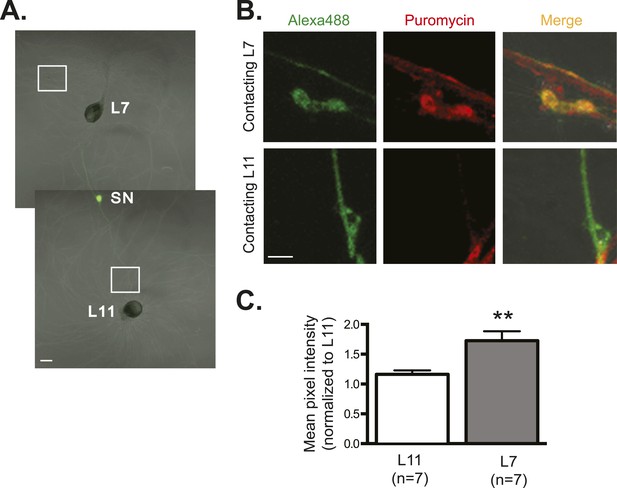
Global translation is also enriched in SN branches contacting L7 MN.
A bifurcated SN was cultured with an L7 target MN and an L11 non-target MN (A) for 3 days. Alexa488 (green) was microinjected into the SN as a volume control for imaging (A). Cultured neurons were processed for ICC for puromycin. 100 μM puromycin was applied to cultured neurons for 10 min in the presence of 200 μM emetine. Puromycin incorporated into newly synthesized peptide was detected by anti-puromycin antibody. Representative high magnification images of areas denoted by white squares in (A) are shown in (B), with Alexa488 (green) in the left panel, anti-puromycin in the middle panel (red), and merged images in the right panel. Arrows indicated puromycin immunoreactivity in SN varicosity (B) in the middle panel. Group data (C) show that the puromycin incorporation is greater in SN branches contacting L7 target MNs than in branches contacting non-target L11 target MNs. Error bars represent SEM. *p < 0.05, paired t-test. Scale bars in (A) =100 μm; in (B) =10 μm.
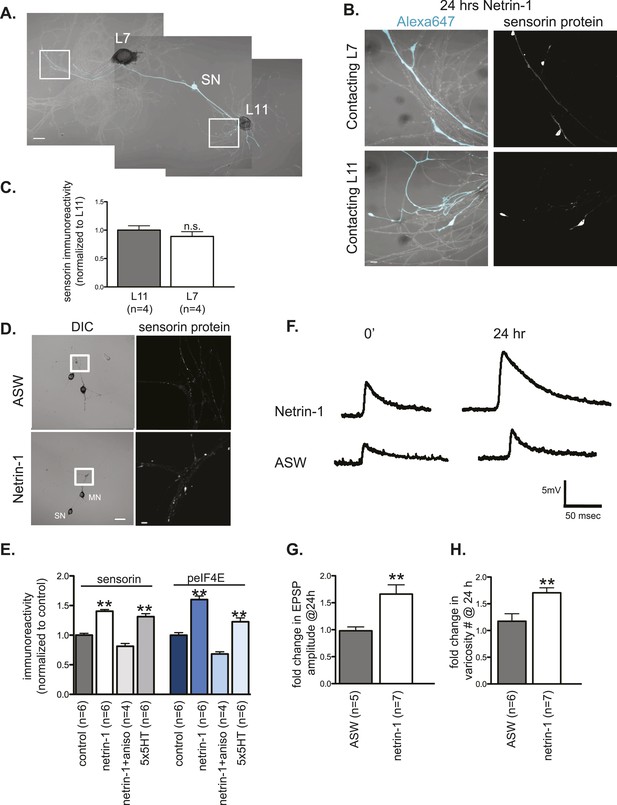
Netrin-1 increases protein synthesis in SNs and strength of SN-target MN synapses.
(A) A bifurcated sensory neuron was cultured with an L7 target MN and an L11 non-target MN for 3 days. Alexa647 was microinjected into SNs and served as a volume filling control for the analysis of immunocytochemistry (ICC). Cultures were incubated with 250 ng/ml recombinant Fc human netrin-1 for 24 hr. Representative high magnification images of areas marked by white squares in (A) are shown in (B), with merged DIC/Alexa488 in the left panels, and sensorin ICC in the right panels. Group data (C) reveal that the concentration of sensorin in netrin-1-treated SNs is the same in neurites contacting L7 target MNs and L11 non-target MNs. We compared sensorin (D) and phospho-eIF4E immunoreactivity (not shown) in SN-LFS target MN cultures in the presence and absence of 250 ng/ml Fc-netrin-1 (24 hr). Representative high magnification images of areas denoted by white squares in left panels of (D) stained with anti-sensorin antibodies are shown in right panels of (D). Group data (E) reveal that netrin-1 triggers an increase in sensorin and phospho-eIF4E immunoreactivity that is equivalent to the increase observed 24 hr after 5 spaced applications of 5HT (which produce long-term facilitation) and that is blocked by the protein synthesis inhibitor anisomycin (10 mM). The effect of netrin-1 on sensorin immunoreactivity in isolated SN processes and on eIF4E and phospho-eIF4e immunoreactivity is shown in Figure 7—figure supplement 1. Netrin-1 also increased synaptic strength: representative traces of EPSPs evoked in LFS MNs after stimulation of SN, at time 0 and 24 hr after incubation with vehicle (artificial seawater, ASW) or netrin-1 are shown in (F); histogram of group data is shown in (G). Histogram in (H) shows fold change in the number of varicosities between SN and LFS MN after 24 hr of incubation with vehicle (ASW) and netrin-1. Error bars represent SEM. **p < 0.001, Student's unpaired t-test for (G) and (H); ANOVA and Dunnett's multiple comparison test for (E). Scale bars in (A) and (D, left panel) =100 μm; in (B), (D, right panel)= 10 μm.
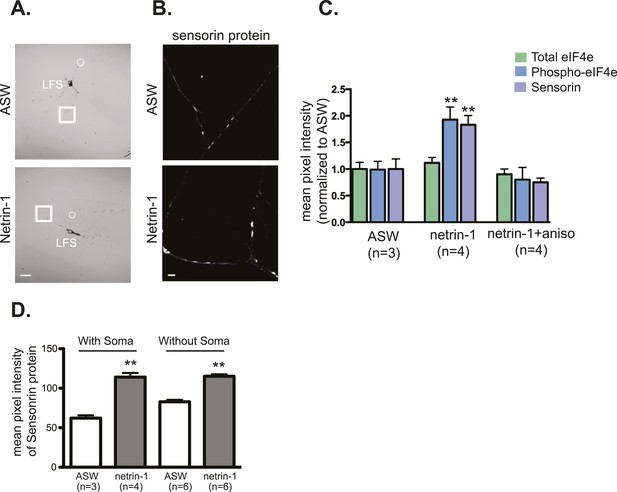
Netrin-1 increases local translation in soma-free SN processes in SN-MN cultures.
SN-MNs were cultured for 3 days and the SN soma (denoted by dotted circle in A) was mechanically removed with sharp glass electrode. 12–18 hr later, cultures were incubated with recombinant Fc human netrin-1 (250 ng/ml for 24 hr) and then processed for immunocytochemistry with sensorin antibodies (B). As shown in the group data in (C), recombinant Fc human netrin-1 increased translation in SN processes in cultures in which the soma was present (with soma) or removed (without soma). Quantification of the effect of netrin-1 (250 ng/ml for 24 hr) in intact SN-MN cultures (3 DIV) on eIF4e and phospho-eIF4e immunoreactivity, with sensorin immunoreactivity as a positive control, is shown in (D). Error bars represent SEM. **p < 0.01 unpaired t-test. Scale bars in (A) =100 μm; in (B) =10 μm.
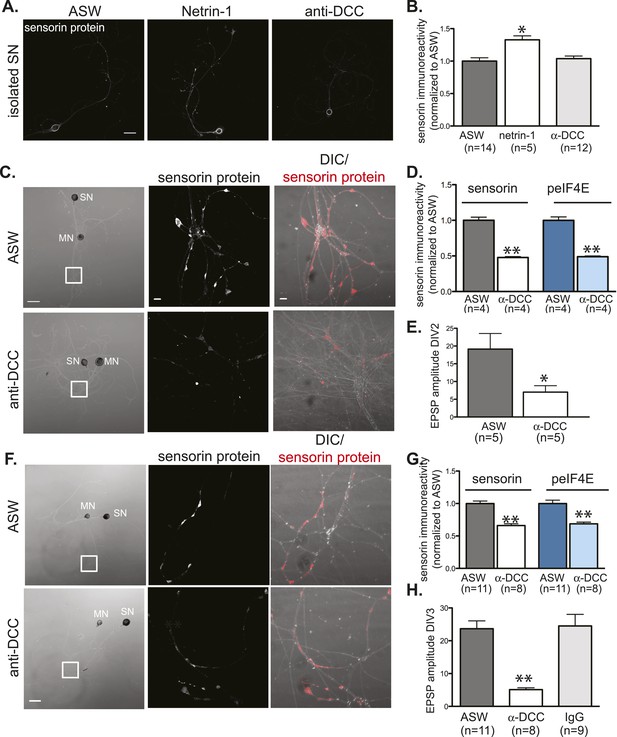
Netrin-1 binds DCC to promote SN translation and to increase strength of SN-MN synapses.
(A) and (B): immunocytochemistry (ICC) of isolated SNs (3DIV) reveals an increase in sensorin immunoreactivity with recombinant Fc human netrin-1 incubation (250 ng/ml for 24 hr), but no change from baseline following 24 hr of incubation with function-blocking anti-DCC antibodies (250 ng/ml). Representative images are shown in (A); group data in (B). To test the effect of function-blocking anti-DCC antibodies on sensorin and phospho-eIF4E (peIF4E) immunoreactivity and synapse formation in SN-LFS target MN cocultures, we cultured isolated SNs for a day, added anti-DCC (250 ng/ml), and 6 hr later paired the SN with a target LFS MN. EPSP amplitude was recorded the next day, and cultures were fixed for ICC. Representative cultures are shown in (C). High magnification images of areas denoted by white squares in left DIC images are shown in the middle (sensorin ICC) and the right (merged DIC and sensorin, red) panels. Group data (D) show that anti-DCC significantly decreases sensorin and peIF4E immunoreactivity, consistent with netrin being released from the MN to drive translation in the SN. SN-LFS MN pairs incubated with anti-DCC antibodies also showed significantly smaller EPSP amplitudes than do control cultures (E). To query the function of netrin-1/DCC signaling in mature, established SN-LFS MN synapses, we cultured SN-LFS MNs for 2 days, added anti-DCC antibodies (250 ng/ml) for 24 hr, and then measured EPSP amplitude and fixed the cultures for ICC. Representative cultures are shown in (F). High magnification images of areas denoted by white squares in left-most panels (F) are shown in middle (sensorin immunoreactivity) and right (merged DIC and sensorin, red) panels. Group data show that anti-DCC antibodies significantly decreased sensorin and peIF4E immunoreactivity (G) and EPSP amplitude between SN-LFS MNs (H). Control cultures were incubated with anti-mouse IgG antibodies (250 ng/ml), which did not affect EPSP amplitude (H). Error bars represent SEM. *p < 0.05, **p < 0.01, unpaired Student's t-test. Scale bars in (A) and in left panel of (C) and (F) = 100 μm; in the middle and the right panels of (C) and (F) =10 μm.
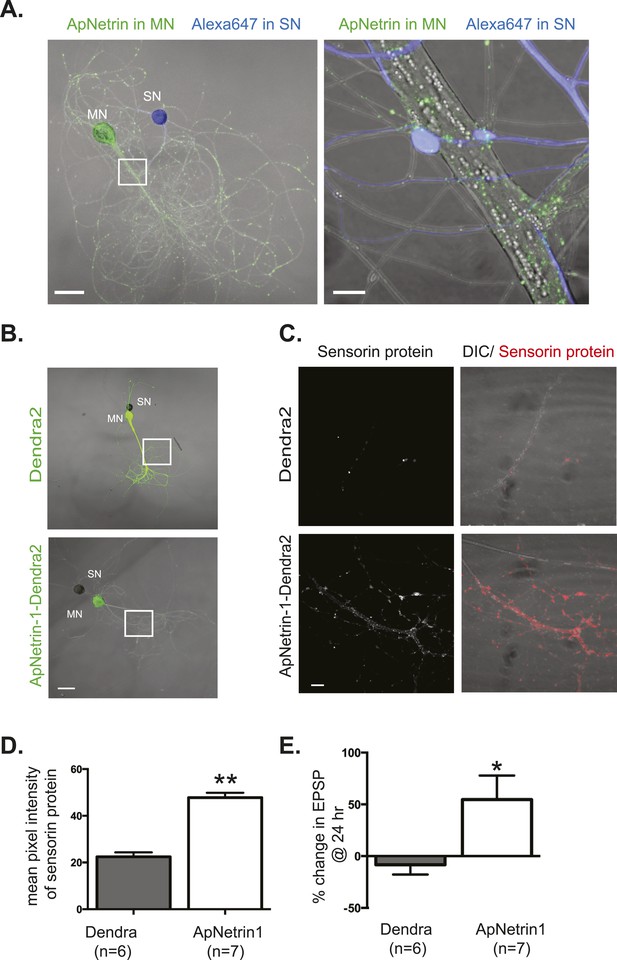
Overexpression of Aplysia netrin-1 increases translation and synaptic strength in SN-MN synapses.
SN-LFS MNs were cultured for 3 days (A–C). The SN was microinjected with Alexa647 (blue) and the MN with a vector expressing Aplysia netrin-1 (ApNetrin-1) c-terminally tagged with dendra2 (green, A). The left panel of A shows a low magnification image, and the right shows high magnification of area outlined with a white square, as a merged DIC/ApNetrin (green) and Alexa647 (SN, blue) image. ApNetrin-dendra is present in a punctate pattern throughout the MN process (A). In (B), on DIV3, vectors expressing dendra2 (upper panel) or Aplysia netrin-1 c-terminally tagged with dendra2 (lower panel) were microinjected into the LFS MN. Basal EPSPs were measured before and 24 hr after microinjection, and cultures were then processed for immunocytochemistry with anti-sensorin antibodies. Representative high magnification images of areas denoted by white squares in (B) are shown in (C) with sensorin protein in the left panel and merged image for DIC/sensorin (red) in the right panel, in cultures in which the MN expresses dendra2 (top) or ApNetrin-dendra2 (bottom). Group data (D) show that sensorin immunoreactivity was increased in SNs paired with MNs overexpressing ApNetrin-1. Overexpression of ApNetrin in the MN also increases the EPSP amplitude, while overexpression of dendra2 in the MN has no effect on EPSP amplitude between SN and MN at 24 hr (E). Error bars represent SEM. **p < 0.01, *p < 0.05, unpaired t-test. Scale bars in (A) = 100 μm; in (B) = 10 μm.
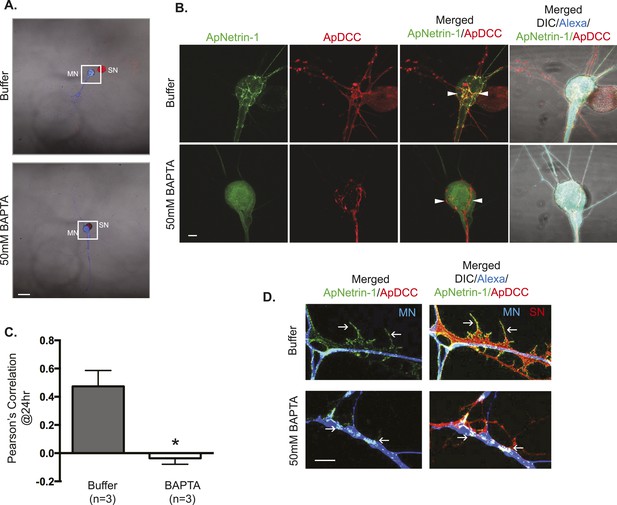
ApNetrin-1 undergoes calcium-dependent release from MN and binds SN.
SN-LFS MNs were cultured for 2 days, and the SN was microinjected with a vector expressing ApDCC c-terminally tagged with mCherry (red) and the MN with Alexa647 (blue) and with a vector expressing Aplysia netrin-1 (ApNetrin-1) c-terminally tagged with dendra2 (green). In the top panel of (A), the MN was microinjected with vehicle; in the bottom panel of (A), the MN was microinjected with BAPTA (50 mM) to chelate calcium. Cultures were imaged before and 24 hr after BAPTA or buffer injection later. The representative micrograph in (A) shows a low magnification image in which the DIC, ApDCC-mCherry (SN, red), and Alexa647 (MN, blue) signals are merged. (B) shows representative images taken 24 hr after microinjection of BAPTA or buffer of the areas highlighted by the white squares in (A). The green signal shows the ApNetrin-1 expressed in the MN; the red signal shows ApDCC expressed in the SN, the merged image is of the green ApNetrin-1 (expressed in MN) and red ApDCC (expressed in SN), and the far-right merged image is of DIC, Alexa647 (blue, MN), ApNetrin-1 (green, expressed in MN), and ApDCC (red, expressed in SN). In cultures in which the MN was microinjected with vehicle, the ApNetrin-1 (green) colocalizes with SN processes that are in contact with the MN soma (arrowheads) (top panel, B). In cultures in which calcium was chelated in the MN by microinjection of BAPTA, the ApNetrin-1 (green) signal is present within the MN soma, and do not colocalize with the ApDCC (red) in SN processes that are in contact with the MN (arrowheads). The amount of colocalization between the ApNetrin-1 signal and the SN (red) in the soma was quantified by Pearson's correlation (C). *p < 0.05, Student's unpaired t-test. Representative images of ApNetrin-1 (green, expressed in MN, blue) signal in distal processes of MNs microinjected with buffer or with BAPTA are shown in (D). In control (buffer-injected MNs) cultures, the green ApNetrin-1 signal decorates the red SN processes (arrows). In cultures in which calcium has been chelated by microinjection of BAPTA into the MN, the green ApNetrin-1 signal remains sequestered within the MN (blue). Scale bars in (A) =100 μm; in (B) = 20 μm; in (D) = 10 μm.
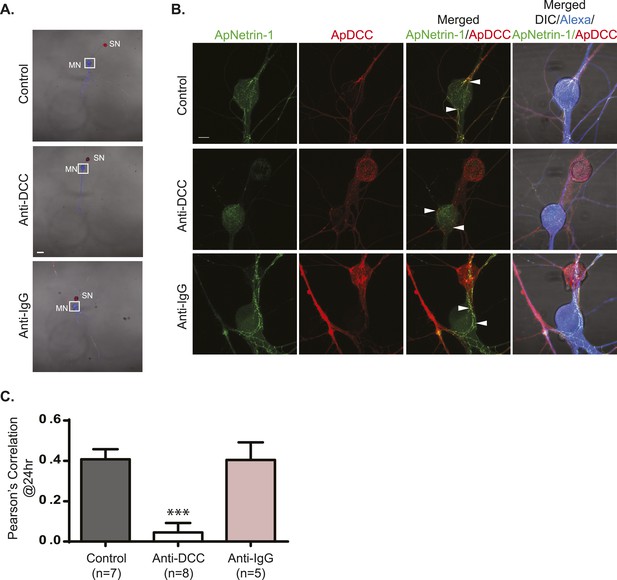
Incubation with anti-DCC antibodies inhibits binding of MN-expressed ApNetrin-1 to SNs.
SN-LFS MNs were cultured for 2 days, and the SN was microinjected with a vector expressing ApDCC c-terminally tagged with mCherry (red) and the MN with Alexa647 (blue) and with a vector expressing Aplysia netrin-1 (ApNetrin-1) c-terminally tagged with dendra2 (green). To test the effect of anti-DCC antibodies on the binding of ApNetrin-1 to the SN, cultures were incubated with anti-DCC antibodies (250 ng/ml) for 24 hr. In control cultures, ASW or non-immune IgG (250 ng/ml) was added for 24 hr. Representative photomicrographs in (A) show low magnification images in which the DIC, ApDCC-mCherry (SN, red), and Alexa647 (MN, blue) signals are merged. (B) shows representative high magnification images taken 24 hr after incubation with anti-DCC antibodies, non-immune IgG, or ASW of the areas highlighted by the white squares in (A). The green signal shows the ApNetrin-1 expressed in the MN; the red signal shows ApDCC expressed in the SN, the merged image is of the green ApNetrin-1 (expressed in MN) and red ApDCC (expressed in SN), and the far-right merged image is of DIC, Alexa647 (blue, MN), ApNetrin-1 (green, expressed in MN), and ApDCC (red, expressed in SN). In cultures incubated with ASW (top panel, B) or non-immune IgG (bottom panel, B), ApNetrin-1 (green) colocalizes with SN processes that are in contact with the MN soma (arrowheads). However, in cultures incubated with anti-DCC antibodies, the ApNetrin-1 (green) signal does not colocalize with ApDCC (red) in SN processes that are in contact with the MN (arrowheads) (middle panel, B). The amount of colocalization between the ApNetrin-1 signal and the SN (red) in the soma was quantified by Pearson's correlation (C). ***p < 0.001, Student's unpaired t-test. Scale bars in (A) = 100 μm; in (B) = 20 μm; in (D) = 10 μm.