Developmental mechanism of the periodic membrane skeleton in axons
Figures
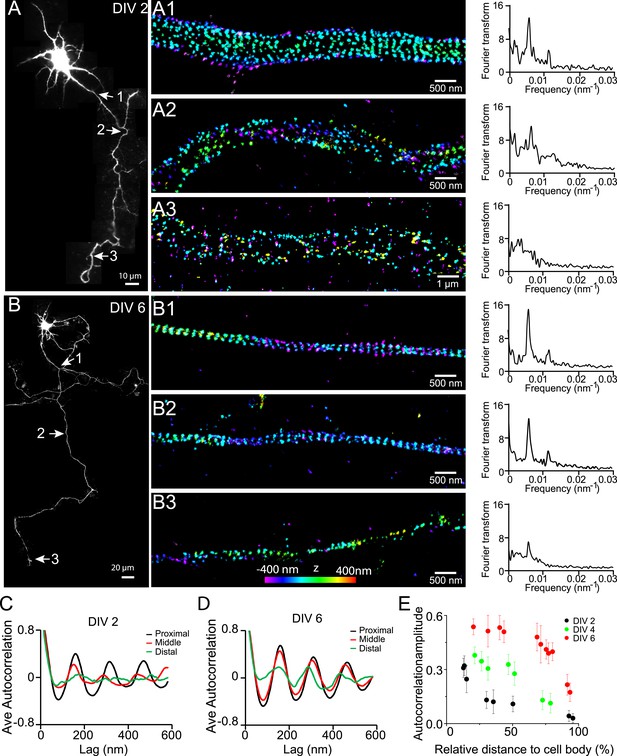
Early development and propagation of the periodic lattice structure in axons.
(A) A DIV 2 neuron was stained with βII spectrin antibody and imaged by 3D STORM. The single long process from the cell is the axon. A1, A2, and A3 are 3D STORM images taken from arrow-indicated regions from A. The Fourier transform analyses of the βII spectrin distribution along the axon shaft are shown on the right. (B) Similar to (A), but for a DIV 6 neuron. (C–D) Autocorrelation analysis of βII spectrin distributions of DIV 2 (C) or DIV 6 (D) neurons at the proximal, middle, and distal regions of axons. Shown are the averaged autocorrelation from multiple segments of axons for each condition. (E) The average amplitude of autocorrelation analysis for different axonal regions of DIV 2, 4, and 6 neurons. The amplitude was measured as the difference between the first peak and the average of the two first valleys of the autocorrelation curve. Error bars are standard deviation from measurements of multiple neurons (n = 7 neurons for DIV 2; n = 6 neurons for DIV 4; n = 9 neurons for DIV 6; from three independent experiments at each DIV). The color bar for 3D STORM image indicates the z-depth of the image and is the same for all of our STORM images.
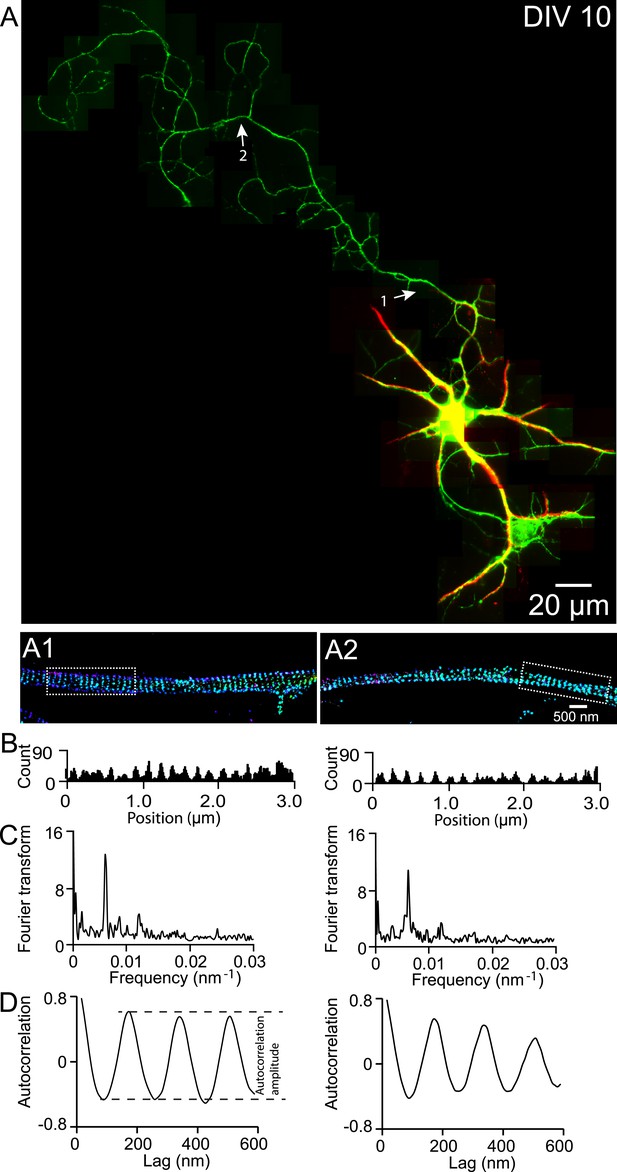
βII spectrin structure in a DIV 10 neuron.
(A) A DIV 10 neuron was immunostained for βII spectrin (green) and Map2 (red) and imaged by conventional fluorescence and 3D STORM microscopy. STORM images of the two arrow-indicated regions along the axon are shown below. (B) Histograms of the βII spectrin localizations from the boxed regions in STORM images. (C) Fourier transform analyses of the βII spectrin localizations from the boxed regions. (D) Autocorrelation analyses of the βII spectrin localizations from the boxed regions.
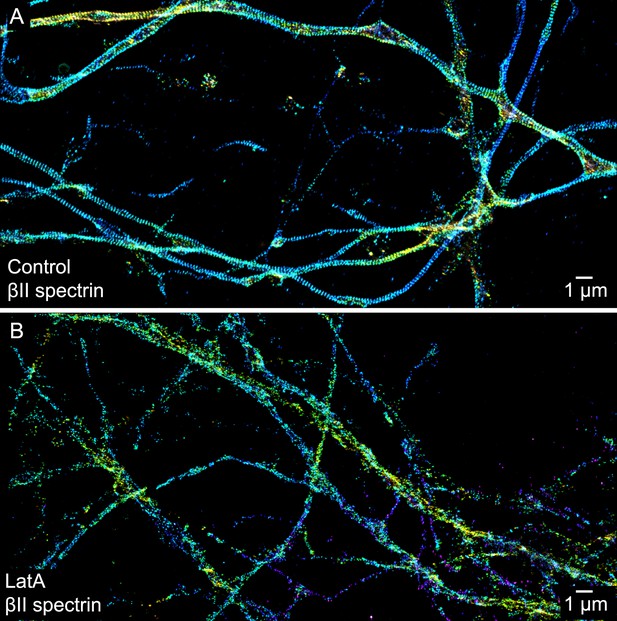
The periodic distribution of βII spectrin depends on actin.
(A) STORM image of βII spectrin in axons of control DIV 10 neurons. (B) STORM image of βII spectrin of LatA-treated DIV 10 neurons (20 µM LatA, 1 hr). Similar results were observed in at least seven independent experiments.
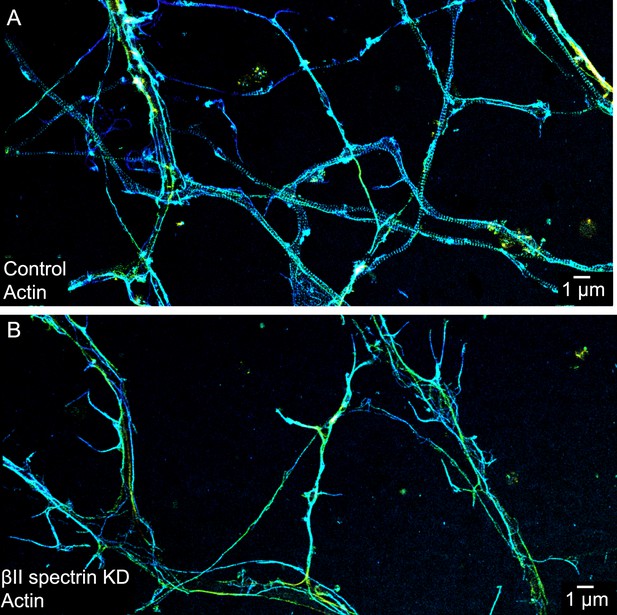
The periodic distribution of actin depends on βII spectrin.
(A) STORM image of actin in axons of control DIV 10 neurons. In some of the thinnest axons, the presence of long actin filaments running along the axons can disguise the periodic structure due to finite resolution. (B) STORM image of actin in axons of βII spectrin-shRNA expressing neurons. Though we picked axon-enriched sample regions for imaging, we cannot preclude the possibility that a small number of the processes may be dendrites. Similar results were observed in 8 independent experiments.
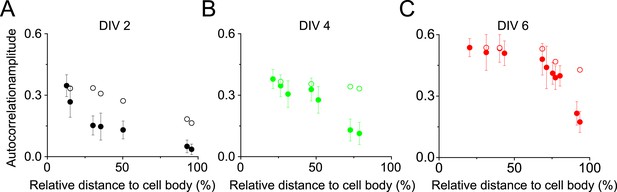
The autocorrelation analysis is not sensitive to the number of localizations present in the periodic structure within the range of the localization numbers that we detected in axon segments.
Since the localization density of βII spectrin decreased towards the distal end of the axons, we tested whether the autocorrelation amplitude measured in Figure 1E would be sensitive to the localization numbers detected in various axon segments. (A) Filled circles: the autocorrelation amplitudes for different axonal regions of DIV 2 neurons reproduced from Figure 1E; open circles: the estimated dependence of the autocorrelation amplitude on the detected localization numbers if the periodic structure remained the same as that in the proximal axon region. To obtain these data, we randomly removed localizations from the proximal axon region to match the localization numbers measured in distal axons at various distances to the cell body, calculated the corresponding autocorrelation amplitudes, and plotted them here as the open circles. (B–C) Same as (A) except that the analyses were for DIV 4 and DIV 6 neurons. Note that the autocorrelation amplitude only decreases slightly when the localization number of a periodic structure was artificially reduced to match those measured in distal axon ends (open circles), and this slight change does not contribute substantially to the measured dependence of the autocorrelation amplitude on the distance to cell body (filled circles).
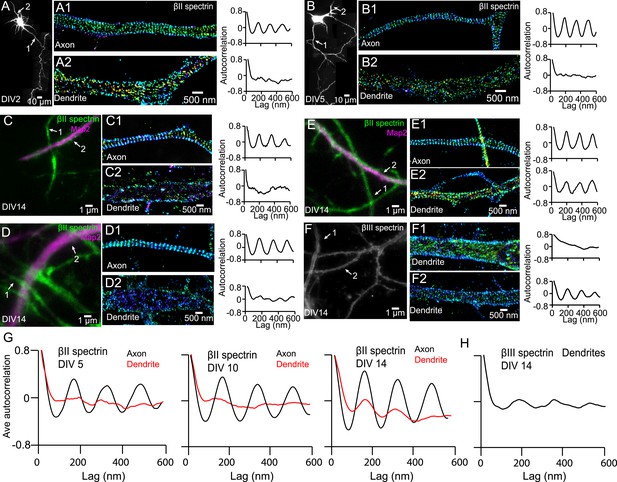
Distributions of dendritic βII and βIII spectrin.
(A–B) DIV 2 and DIV 5 neurons were immunostained with βII spectrin, and both axonal and dendritic processes were imaged by 3D STORM. The reconstructed neuron image, 3D STORM images of axon and dendrite from indicated regions (arrows), and autocorrelation analyses are shown. (C–E) DIV 14 neurons were immunostained with βII spectrin and a dendritic marker Map2, and the βII spectrin was imaged by 3D STORM. The conventional images, 3D STORM images of axons and dendrites from indicated regions (arrows), and autocorrelation analyses are shown. (F) DIV 14 neurons were immunostained with βIII spectrin. The conventional image, STORM images of βIII spectrin from two indicated dendritic regions, and autocorrelation analyses are shown. βIII spectrin only stains for dendrites, and we did not observe detectable βIII spectrin in axons. (G) Average autocorrelation analyses of βII spectrin in axons and dendrites of neurons at DIV 5, DIV 10, and DIV 14 (n = 14 neurons for DIV 5; n = 11 neurons for DIV 10; n = 16 neurons for DIV 14; at least three independent experiments at each DIV). (H) Average autocorrelation analysis of βIII spectrin of DIV 14 neurons (n = 12 neurons, four independent experiments).
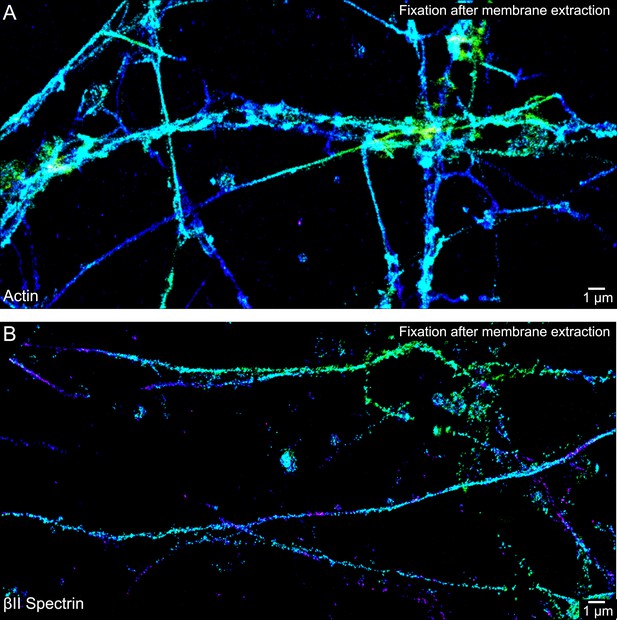
The periodic actin structure is disrupted if the neurons are subjected to membrane extraction prior to fixation.
DIV 10 neurons were extracted with 1% Triton X-100 for 3 min, followed by two quick rinses, and then fixed with 0.2% GA for 20 min. See ‘Materials and methods’, ‘Fluorescence labeling of neurons’ section, for detailed procedure. 3D STORM images of actin and βII spectrin are shown in (A) and (B), respectively. Similar observations were found in six independent experiments.
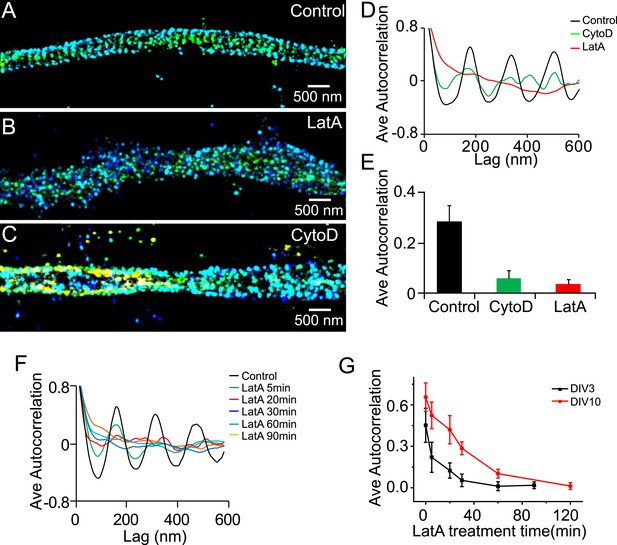
The periodic structure of βII spectrin depends on actin during early development.
(A–C) DIV 3 neurons were either untreated or treated with latrunculin A (LatA, 20 µM) or cytochalasin D (CytoD, 50 µM) for 1 hr and subsequently immunostained with βII spectrin antibody for 3D STORM imaging. Shown here are representative images of βII spectrin in proximal axonal regions from control (A), LatA-treated (B) and CytoD-treated (C) neurons. (D) Average autocorrelation analyses of βII spectrin from multiple axon segments of control, LatA-treated, and CytoD-treated DIV 3 neurons (n = 6 neurons for control; n = 7 neurons for LatA-treated; n = 7 neurons for CytoD-treated conditions; at least three independent experiments for each condition). (E) The average autocorrelation amplitudes from control, LatA-treated, and CytoD-treated DIV 3 neurons. (F) Average autocorrelation analyses of βII spectrin from multiple axon segments of control and LatA-treated DIV 3 neurons at different treatment time (n > 5 neurons for each condition, three independent experiments). The axon segments are taken from the proximal axonal regions near the cell bodies. (G) DIV 3 and DIV 10 neurons were treated with 20 µM LatA for indicated amount of time, and the average autocorrelation amplitude of βII spectrin from these neurons are shown. Error bars are standard deviation from measurements of multiple neurons (n = 6 neurons for DIV 3; n = 8 neurons for DIV 10; four independent experiments at each DIV).
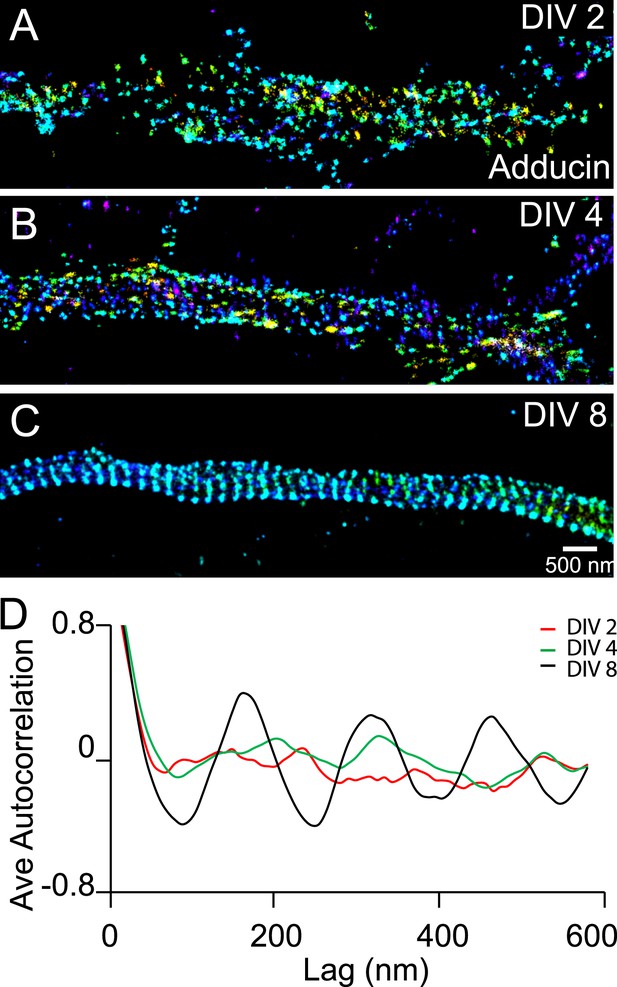
Recruitment of adducin into the periodic lattice structure during development.
(A–C) Neurons were immunostained for adducin and imaged by 3D STORM. Shown here are representative images of adducin at proximal axonal regions from DIV 2, 4, and 8 neurons. (D) Average autocorrelation analyses of adducin at proximal regions of axons near the cell body. The autocorrelation curves are averaged from multiple neurons (n = 5 neurons for DIV 2; n = 7 neurons for DIV 4; n = 6 neurons for DIV 8; three independent experiments at each DIV).
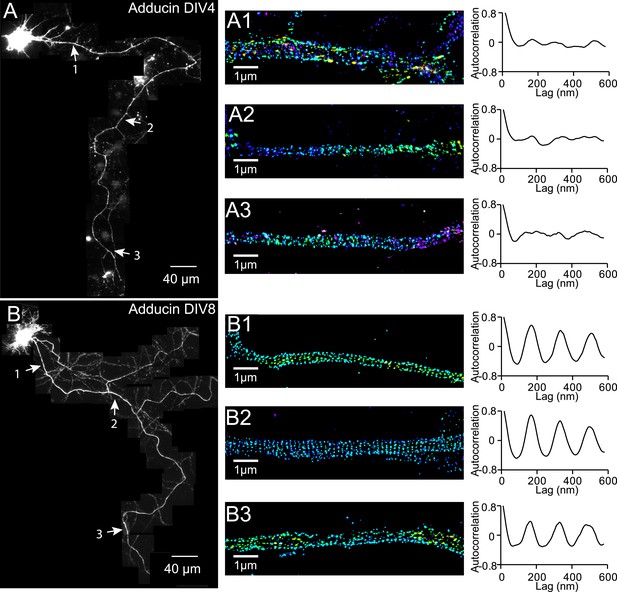
Distribution of adducin in axons.
Neurons were immunostained for adducin and imaged by 3D STORM. The reconstructed neuron image, 3D STORM images of indicated regions (arrows), and corresponding autocorrelation analyses of DIV 4 (A) and DIV 8 (B) neurons are shown.
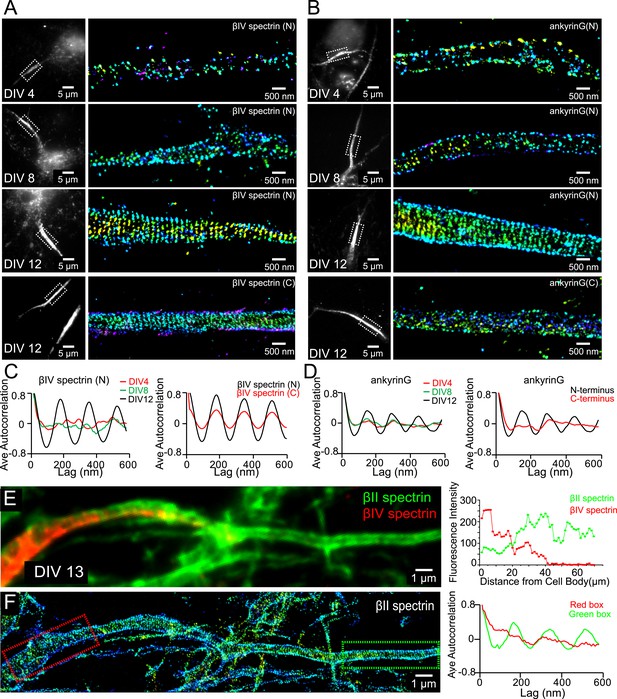
Assembly of AIS components into the periodic lattice structure during late developmental stages.
(A and B) Neurons were immunostained with antibodies against βIV spectrin N-terminus (βIV spectrin (N)), βIV spectrin C-terminus (βIV spectrin (C)), ankyrin G spectrin-binding domain (ankyrin G (N)), or ankyrin G C-terminus (ankyrin G (C)), and imaged at various DIVs by 3D STORM. Representative conventional images and STORM images from the boxed region at different developmental stages are shown. (C) Left: average autocorrelation analyses of βIV spectrin N-terminus from neurons at different developmental stages (n = 9 neurons for DIV 4; n = 12 neurons for DIV 8; n = 16 neurons for DIV 12; at least three independent experiments at each DIV). Right: average autocorrelation analyses of βIV spectrin N-terminus and C-terminus of DIV 12 neurons (n = 13 neurons for N-terminus and n = 15 neurons for C-terminus, three independent experiments). (D) Left: average autocorrelation analyses of ankyrin G spectrin-binding domain (near N-terminus) from neurons at different developmental stages (n = 12 neurons for DIV 4; n = 15 neurons for DIV 8; n = 14 neurons for DIV 12; at least three independent experiments at each DIV). Right: average autocorrelation analysis of ankyrin G N-terminus and C-terminus of DIV 12 neurons (n = 9 neurons for N-terminus and n = 10 neurons for C-terminus, three independent experiments). (E and F) A DIV 13 neuron was immunostained with βIV and βII spectrin antibodies. βII spectrin was subjected for 3D STORM imaging. (E) Left: conventional image of βII and βIV spectrin in axon. Right: fluorescent intensity profile of βII and βIV spectrin along the axon. (F) Left: STORM image of βII spectrin in the same region. Right: autocorrelation analyses of βII spectrin from red- and green-boxed regions.
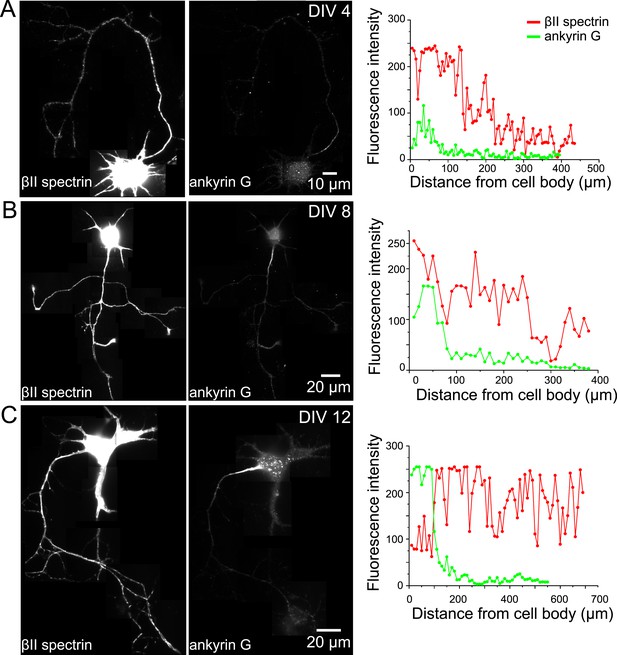
The expression profile of βII spectrin and ankyrin G in axons at different developmental stages.
Neurons at DIV 4 (A), DIV 8 (B), and DIV 12 (C) were immunostained for βII spectrin and ankyrin G. Reconstructed neuron images based on βII spectrin fluorescence, ankyrin G fluorescence, and the fluorescence intensity profiles of both βII spectrin and ankyrin G along axons are shown.
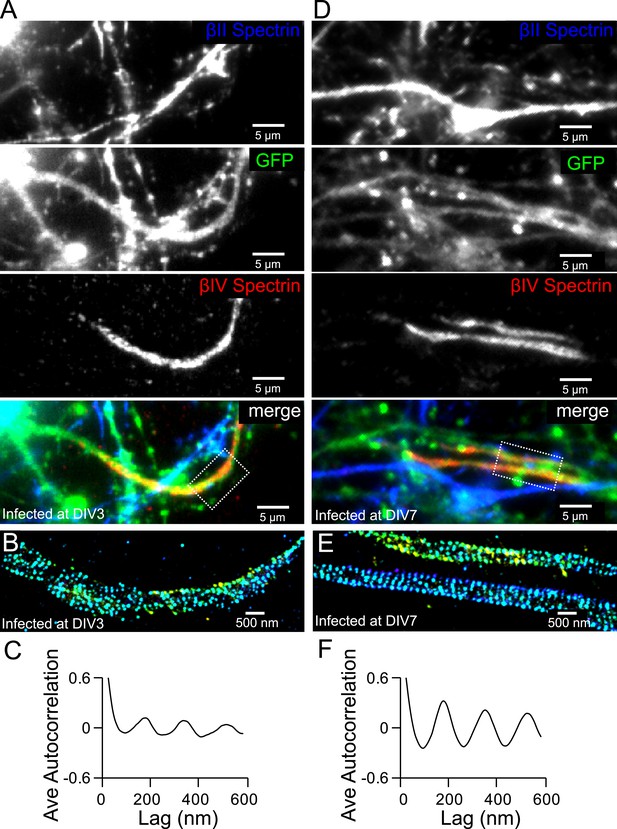
The formation of the periodic βIV spectrin structure in the AIS is dependent on βII spectrin.
(A) Neurons were infected with βII spectrin-shRNA expressing adenovirus at DIV 3 and subsequently stained for βII spectrin and βIV spectrin at DIV 12. βIV spectrin was subjected for STORM imaging. Infected neurons have a GFP signal. The conventional images of βII spectrin, GFP, and βIV spectrin and the overlay image are shown. Efficient knockdown is demonstrated by lack of βII spectrin in GFP-positive process. (B) STORM image of βIV spectrin from boxed region in (A). (C) Average autocorrelation analysis of βIV spectrin from βII spectrin knockdown neurons (n = 11 neurons, three independent experiments). (D–F) Similar to (A–C) except that the virus was added at DIV 7 (n = 9 neurons, four independent experiments).
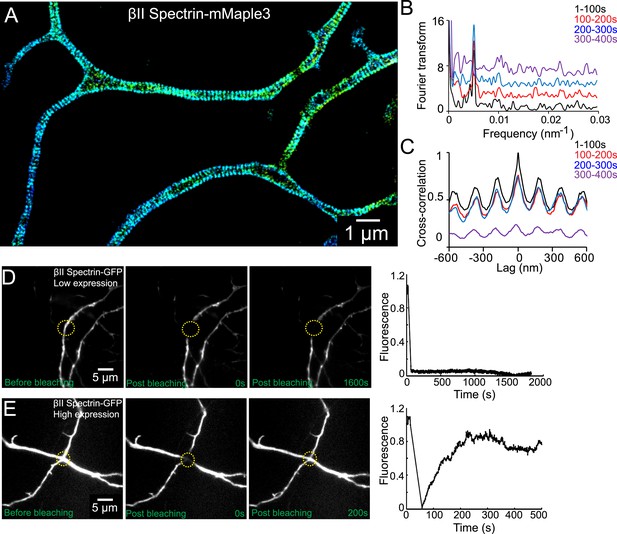
The periodic lattice structure is stable in live neurons.
(A) 3D STORM image of βII spectrin-mMaple3 in live neurons at DIV 10. (B) The STORM movie was segregated into four different time windows. Fourier transform analysis of each time window is shown. The baseline of Fourier traces is shifted manually for clear visualization. (C) Cross-correlation analysis of βII spectrin across different time windows. The black curve is the autocorrelation of the image during 0–100 s. The color curves are the cross-correlation between 0–100 s and later time windows. Similar results were found in six independent experiments. (D–E) FRAP analyses of βII spectrin in DIV 10 neurons. Neurons were transfected with βII spectrin-GFP at DIV 8. (D) Representative neurons at a low βII spectrin expression level, where βII spectrin-GFP molecules were incorporated into the periodic structure. The images before photo-bleaching, 0 s post-bleaching, 1600 s post-bleaching, and the fluorescence recovery trace are shown. (E) The fluorescence recovery of representative neurons with a high βII spectrin expression level, where most βII spectrin-GFP molecules were not incorporated into the periodic structure. Similar results were found in at least nine independent experiments.
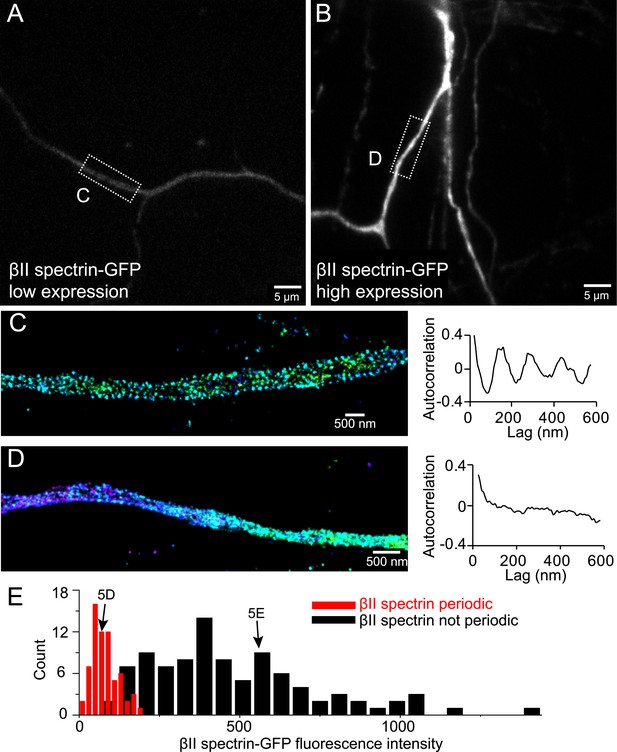
Incorporation of βII spectrin-GFP into the periodic lattice structure in overexpressing neurons.
Neurons were transfected with βII spectrin-GFP at DIV 8 and immunostained for GFP at DIV 10. Representative conventional images of βII spectrin-GFP in low expression and high expression neurons are shown in (A) and (B), respectively. The STORM image and autocorrelation analyses of boxed regions are shown in (C) and (D). (E) Quantification of the expression levels of βII spectrin-GFP in transfected neurons that show (red) or do not show (black) the periodic lattice structure. The expression levels of the neurons subject to the FRAP analysis in Figure 5D,E are indicated by arrows.
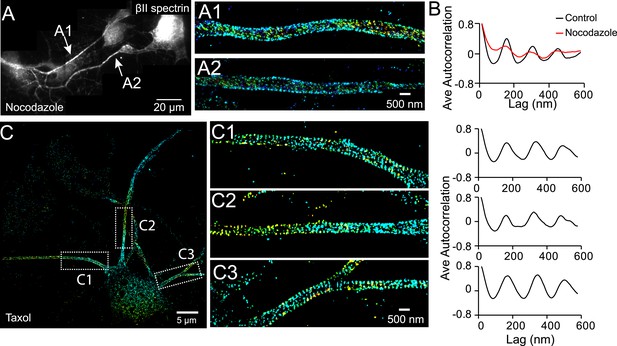
The periodic structure of βII spectrin relies on intact microtubules.
(A and B) DIV 4 neurons were either untreated or treated with nocodazole (50 µM) for 1 hr and immunostained with βII spectrin antibody for 3D STORM. A reconstructed neuron image from nocodazole-treated neurons and two STORM images are shown in A. (B) Average autocorrelation analyses of βII spectrin from multiple control and nocodazole-treated neurons (n = 9 neurons for control and n = 8 neurons for nocodazole-treated conditions; three independent experiments for each condition). (C) Neurons were treated with taxol (5 nM) at DIV 3 for 3 days, which induces the growth of multiple axon-like processes. A representative STORM image of a treated neuron at DIV 6, the enlarged images of the boxed regions, and the autocorrelation analyses (n = 10 neurons, four independent experiments) are shown.
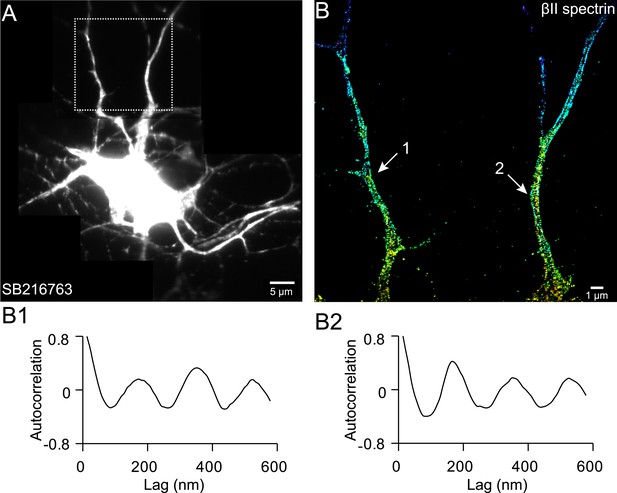
The periodic βII spectrin structure can be induced in multiple processes by axon-promoting small molecules.
Neurons were treated with SB 216763 at DIV 1, a drug that inhibits GSK3β and promotes the growth of multiple axon-like processes, and immunostained for βII spectrin at DIV 5. A representative conventional image of βII spectrin (A), STORM image of boxed region (B), and autocorrelation analyses of the arrow-indicated regions (B1 and B2) are shown. Similar results were found for three independent experiments.
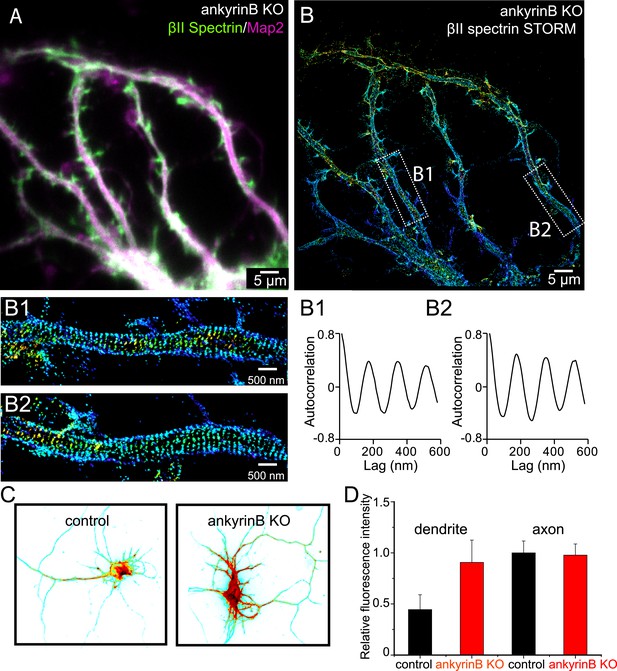
Role of ankyrin B in the regulation of the periodic lattice structure.
(A–B) DIV 10 neurons from ankyrin B knockout (KO) mice were immunostained for βII spectrin and a dendritic marker Map2 and imaged. (A) Conventional image of βII spectrin and Map2. (B) 3D STORM of βII spectrin. The image is taken from the green-boxed region of the neuron in Figure 7—figure supplement 1. The enlarged STORM image and autocorrelation analyses of boxed regions are shown in (B1) and (B2). Similar results were found in four independent experiments. (C) Conventional βII spectrin image from wild-type (control) and ankyrin B KO DIV 10 neurons. The fluorescence intensity is coded by color, with red indicating higher expression. (D) The relative fluorescence intensity of βII spectrin in dendrites and axons of wild-type and ankyrin B KO neurons (n = 14 neurons for wild-type and n = 13 neurons for ankyrin B KO; three independent experiments).
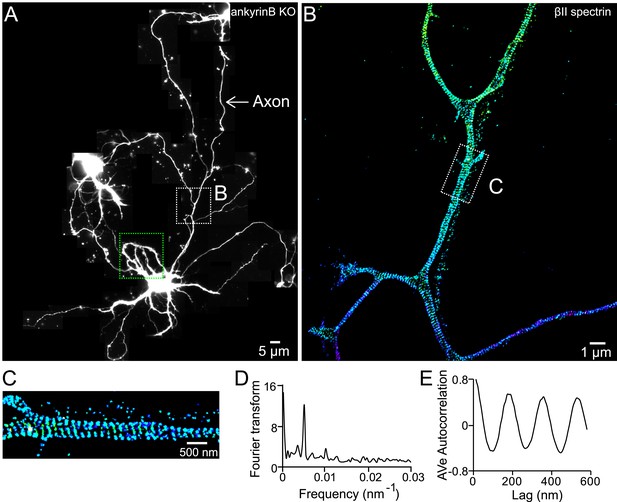
The βII spectrin structure in axons of ankyrin B KO neurons.
(A) Reconstructed image of an ankyrin B KO DIV 10 neuron. βII spectrin was subjected for 3D STORM image in both dendrites and axons. The STORM image of dendrites in green-boxed region is shown in Figure 7. The STORM image of axon in white-boxed region is shown in (B). (C) Enlarged STORM image of the boxed region in (B). (D–E) Fourier transform and autocorrelation analyses of the axon segment shown in C.
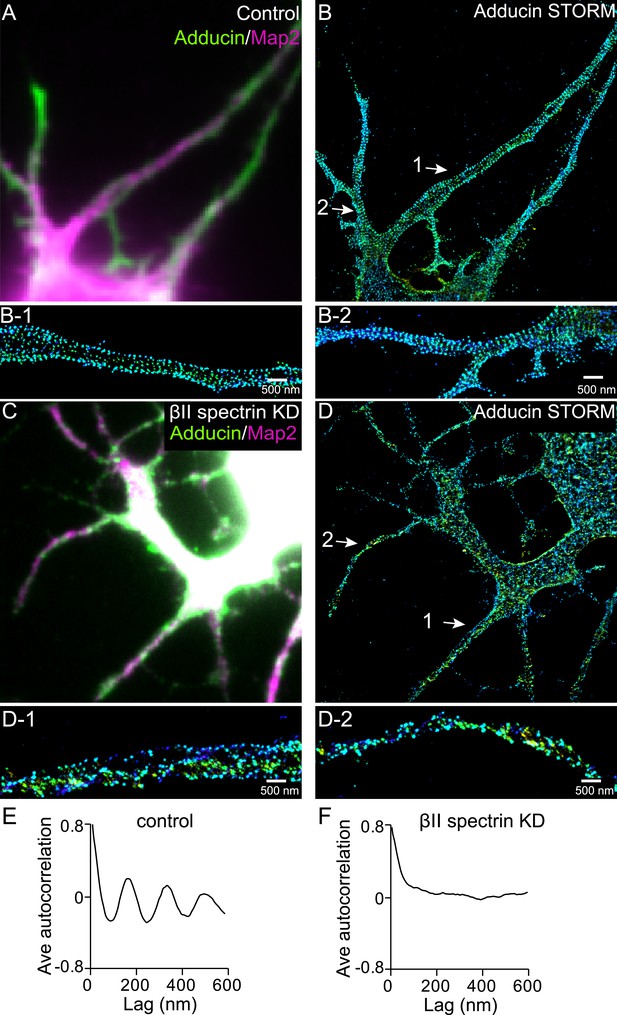
Formation of the periodic lattice structure in dendrites of ankyrin B knockout neurons depends on βII spectrin.
(A–B) Ankyrin B knockout neurons were immunostained for adducin (green) and MAP2 (magenta), and imaged by conventional (A) and 3D STORM (B) microscopy. Magnified STORM images of adducin for arrow-indicated regions are shown in (B-1) and (B-2). (C–D) Ankyrin B knockout neurons were infected with βII spectrin-shRNA expressing adenovirus, immunostained for adducin and MAP2, and imaged by conventional (C) and 3D STORM (D) microscopy. Infected neurons were marked by GFP signal expressed from the virus (not shown). Magnified STORM images of adducin from arrow-indicated region are shown in (D-1) and (D-2). (E) Average autocorrelation analysis of adducin distribution in dendrites of ankyrin B knockout neurons (n = 9 neurons, four independent experiments). (F) Average autocorrelation analysis of adducin distribution in dendrites of ankyrin B knockout neurons treated with βII spectrin-shRNA expressing adenovirus (n = 15 neurons, three independent experiments).
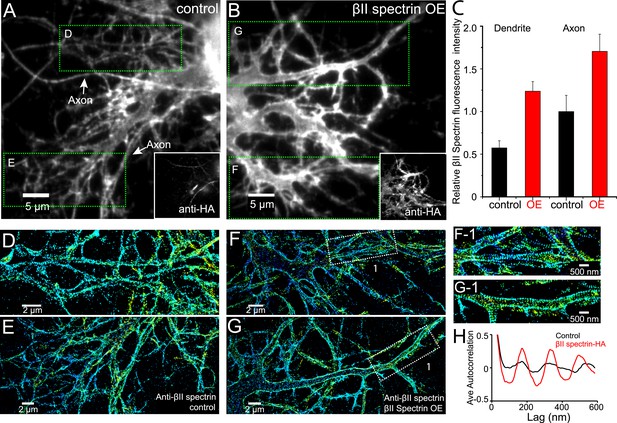
Local βII spectrin concentration determines the formation of the periodic lattice structure.
DIV 9 neurons were either mock-transfected or transfected with βII spectrin-HA and immunostained for HA and βII spectrin. βII spectrin were subsequently imaged by 3D STORM. (A–B) Conventional images of βII spectrin in dendrites of a control neuron and a βII spectrin-HA overexpressing (OE) neuron. The HA image is shown in the insets. (C) The relative fluorescence intensity for βII spectrin in dendrites and axons of control and βII spectrin-HA overexpressing neurons (n = 10 neurons for control and n = 15 neurons for βII spectrin-HA overexpressing conditions; three independent experiments for each condition). (D–E) STORM images of βII spectrin from green-boxed regions in A. (F–G) STORM images of βII spectrin from green-boxed regions in B. (F-1) and (G-1) are enlarged images of the white-boxed regions in F and G, respectively. (H) Average autocorrelation analyses of βII spectrin in dendrites of control and βII spectrin-HA overexpressing neurons (n = 8 neurons for control and n = 12 neurons for βII spectrin-HA overexpressing conditions; three independent experiments for each condition).
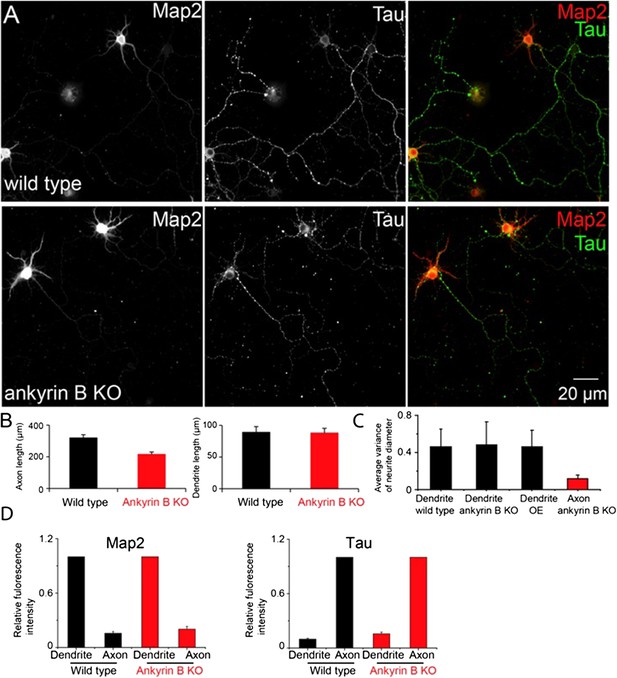
The dendrite morphology and axon-dendrite polarity of ankyrin B knockout neurons.
A) Conventional images of Map2 and Tau in wild type and ankyrin B knockout (KO) neurons. B) The quantification of the average length of axons (left panel) and dendrites (right panel). C) Quantification of the diameter variance for dendrites from wild type, ankyrin B KO and βII spectrin overexpressing (OE) neurons, and the diameter variance for axons from ankyrin B knockout neurons. D) Quantification of Map2 and Tau fluorescence intensity in dendrites and axons from wild type and ankyrin B knockout neurons.
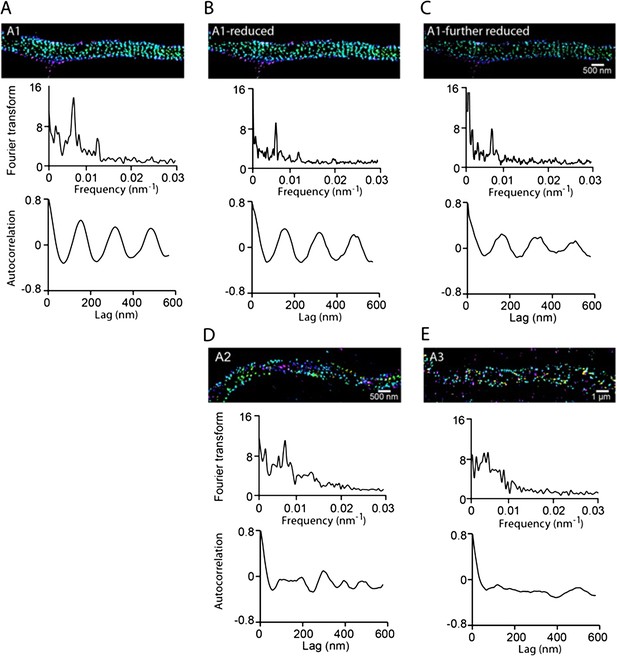
A) Top panel: STORM image of βII spectrin reproduced from Figure 1–A1. Middle panel: Fourier analysis of the localization distribution of this segment of the axon. Bottom panel: autocorrelation analysis of the same segment. B) Same as (A) except that some localizations are randomly removed from the A1 image so that the number of localizations matches that of the A2 image. C) Same as (A) except that some localizations are randomly removed from the A1 image so that the number of localizations matches that of the A3 image. D-E) Top panels: STORM images of βII spectrin reproduced from Figure 1–A2 and Figure 1–A3. Middle panels: Fourier analyses. Bottom panel: autocorrelation analyses.